, Yaping Hua1, 2, Karl-Henning Kalland1, 2 , Xisong Ke1, 2, Jan Roger Olsen1, 2, Anne Margrete Øyan1, 2 and Yi Qu1, 2
(1)
Department of Clinical Science, University of Bergen, Bergen, Norway
(2)
Department of Microbiology, Haukeland University Hospital, Bergen, Norway
Abstract
Human prostate cancer is initiated in a benign prostate epithelial cell which gains the potential to progress to metastatic disease. The exact cell of origin of prostate cancer has been debated in recent years based upon different models. Primary prostate epithelial cells have restricted life-spans in culture, but can be immortalized. Prostate cancer cell lines have been difficult to establish and new ones are desirable. Attempts to transform benign prostate epithelial cells in vitro have proved difficult without the use of strong carcinogens or oncogenes in processes not likely to mimic closely carcinogenesis in the aging human prostate. Models of epithelial-to-mesenchymal transition (EMT) and cancer stem cells in prostate carcinogenesis have become available, and advances in three-dimensional organoid culture technology represent a breakthrough in prostate cancer research. Organoids may recapitulate multiple features of prostate cancer and have the potential to replace costly and laborious animal experiments. Still, animal models are needed to investigate and validate molecular mechanisms and to develop therapeutic principles in the pipeline between in vitro experiments and clinical applications. Although mice represent the most common experimental animal in prostate cancer research, species like rat, dog, and zebrafish may have advantages depending upon the hypothesis or question. Animal models can generally be categorized into spontaneous or induced development of cancer, immunodeficient animals with xenografts, and genetically engineered animals. In prostate cancer, neuroendocrine differentiation and bone metastases are prevalent in the final stages of cancer progression and animal models that recapitulate these processes are available.
Keywords
Prostate cancerAnimal modelCell culture modelProstate cellOrigin of the Prostate Cancer Cell
The prostate gland consists of multiple small glandular elements embedded in a vascularized connective tissue stroma (Fig. 19.1). Each small glandular element is defined by an outer basement membrane on which a layer of basal epithelial cells is situated (Fig. 19.1). In these basal cells, the androgen receptor (AR) is silenced. One prevailing view regarding normal prostate epithelial differentiation is that when AR is induced by unknown mechanisms in the presence of androgen, basal cells differentiate into luminal cells and a minor population of neuroendocrine cells. These events may be reproduced in in vitro cultures of immortalized basal cells which can be propagated as transit amplifying (TA) cells in growth media with low-calcium concentration [2] (Fig. 19.1). The lineage relationships between basal and luminal cells, and in particular which one is the cell of origin of prostate cancer and of putative prostate cancer stem cells (CSCs), have been vigorously debated [3, 4]. The bulk of prostate adenocarcinoma cells retain mostly luminal cell expression patterns, but evidence has been provided to support both basal cells and luminal cells as the cell of origin of prostate cancer. Recent work has revealed considerable plasticity in the differentiation pathways and suggested that it may be more fruitful to focus on the activated regulatory networks and mechanisms [4]. There are, however, strong clues that the key regulatory mechanisms in normal prostate epithelial differentiation are retained in a perverted form in advanced prostate cancer. This notion is exemplified by the importance of the AR transcription factor during prostate cancer progression, including in castration-resistant prostate cancer (CRPC) [5], and by the neuroendocrine differentiation [6, 7] in end-stage prostate cancer. The unknown activation status of AR in putative prostate CSCs remains an important unresolved question with significant therapeutic consequences [8].


Fig. 19.1
Possible normal differentiation pathways from prostate stem cells to epithelial basal cells, luminal cells, and neuroendocrine (NE) cells and possible transformation pathways to prostate cancer stem cells and cancer cells (TA = transit amplifying cell). Stained histological sections of prostate benign (upper) and cancer (lower) tissue are from publication [1]
Cell Culture Modeling of Prostate Carcinogenesis
Primary prostate epithelial cells (PrECs) can be obtained from biopsies and surgical material, as well as commercially, and can be propagated for a limited number of passages in monolayers. PrECs have been immortalized using either hTERT (human telomerase reverse transcriptase) or the transforming elements of DNA viruses [9–11]. The 957E/hTERT cells [12, 13] and EP156T cells [14] were immortalized by exogenous expression of hTERT. PZ-HPV7, CA-HPV10, and RWPE-1 cells were immortalized by human papilloma virus (HPV) transforming elements [15]. Immortalization of PrECs has been achieved without exogenous gene expression [4, 16–18], but there is no model available of PrECs that spontaneously have transformed into malignant cell lines in vitro.
Many attempts have been made to study malignant transformation of benign prostate cells in culture, but the use of strong carcinogens or oncogenic viral elements was necessary to achieve transformation [19]. Forced transformation may be useful for many purposes but is suboptimal when physiological mechanisms of transcriptional reprogramming during prostate carcinogenesis are investigated. Physiological selection pressure was applied to EP156T cells by keeping the cells in a confluent monolayer with regular replacement of fresh growth medium. After several months progeny EPT1 cells with reduced cell-to-cell contact inhibition dominated the culture. EPT1 cells had undergone EMT but were not tumorigenic [19]. EMT turned out to be the first step in the accumulation of malignant traits in a succession of progeny cells, eventually resulting in tumorigenic EPT3 cells (Fig. 19.2) [20, 21]. This model encompasses benign transit amplifying epithelial cells (EP156T), benign (EPT1) and pre-malignant (EPT2) mesenchymal type cells, and tumorigenic (EPT3-N04/EPT3-PT1) and metastatic (EPT3-M1) cells in mice (Fig. 19.2). The very different phenotypes share a common genotype. Forensic grade DNA microsatellite, karyotype, and copy number breakpoint analyses verified progeny authenticity [20]. Each of the different cell types can be passaged indefinitely and to high cell numbers in sub-confluent monolayers. Subpopulations of tumor-initiating EPT3 cells (TICs) show activation of the WNT pathway and an autocrine IL6/STAT3 loop and show increased resistance to apoptosis and anoikis [20]. Genome-wide analyses revealed that epigenetic promoter patterns at different steps of the model corresponded strongly with coordinated expression changes of regulatory gene modules, such as HOX and microRNA genes, and structural gene modules, such as desmosome and adherens junction genes [19, 21, 22]. The model demonstrates, however, an absence of gene expression pattern characteristic of the bulk cellular population of prostate adenocarcinomas. Prostate luminal gene expression is strikingly absent, and the model is more likely to represent features of mesenchymal type cells in prostate cancer progression. In fact, evidence of EMT in the progression of primary prostate cancer has been shown in patient tissue [23]. However, the role of EMT, and its significance, in early prostate carcinogenesis, in metastasis, and in the development of resistance to androgen deprivation treatment (ADT) and other prostate cancer therapy requires further investigation in available cell cultures, preclinical models, and patient samples [24]. A particular pressing issue is the mounting evidence that ADT and highly potent inhibitors of AR function, such as enzalutamide, might induce EMT and more aggressive cancer, possibly involving prostate CSCs [25–27]. Alternative hypotheses have been discussed, such as the existence of a common progenitor prostate cancer stem cell that gives rise to both the neuroendocrine-like and adenocarcinoma components, and both these components continue to evolve and respond to selective pressures in parallel [26]. A negative feedback loop between AR and ZEB1 wherein ADT upregulates ZEB1 expression toward induction of EMT has been demonstrated [28]; ZEB1 in turn is indirectly able to induce stem cell factors through repression of miR200 family members which themselves repress stemness factors including SOX2 and KLF4 [29].


Fig. 19.2
Overview of the EPT prostate stepwise tumorigenesis model. EPT1 cells were selected for loss of cell contact inhibition. EPT2 cells were selected from foci of confluent EPT1 cells and cloned in soft agar. EPT2-D5-HS was selected in protein-free medium. EPT3 cells were selected following subcutaneous injection. Cells were recovered from the EPT3 tumor. The progressive accumulation of malignant hallmarks is summarized [20]
Prostate Cancer Cell Lines
LNCaP, PC-3, and DU-145 and their metastatic derivatives are still the most widely used human prostate cancer cell lines despite the length of time these “classical” cell lines have been in culture since isolated from human metastases [11, 28–31]. It has proven to be difficult to establish stable cell lines from primary prostate cancer. The Prostate Cancer Cell Lines Database provides an updated online overview of registered prostate cancer cell lines (http://www.capcelllines.ca/). LNCaP cells are androgen responsive in contrast to the AR-negative PC-3 and DU-145 cell lines, but are less effective in forming tumors and metastatic colonies in mouse xenografts. Reviews summarize in vitro models of AR signaling in prostate cancer [28] and useful cell lines for mouse xenografting [31]. Among additional prostate cancer cell lines, the VCaP and DuCaP cell lines express AR and the androgen-responsive TMPRSS-ERG fusion, and the 22Rv1 cell line is considered an in vitro model of CRPC [28]. 22Rv1 cells express the androgen-independent AR splice variant denoted AR-V7 [32]. These and additional prostate cancer cell lines have provided important information on prostate cancer but also have many limitations. With their origin in metastatic tissue and lack of exact passage history, they cannot be used to recapitulate prostate carcinogenesis, and it is difficult to estimate which genetic changes are due to in vitro culture selection. Thus, in one genome-wide ChIP-seq study, only 3% overlap in AR-binding sites were found between prostate cancer cell lines and prostate cancer tissue prior to treatment [33]. Since most cell lines were isolated from patients who had undergone treatment, this could also be a factor in the differential gene expression. In prostate cancer research, isolation of additional prostate cancer cell lines is desirable, in particular from primary cancer tissue, in addition to more complex and emerging models.
In Vitro Modeling of the Prostate Cancer Microenvironment and Three-Dimensional (3D) Growth Conditions
Cancer cells develop, proliferate, and invade in crosstalk with a microenvironment consisting of fibroblasts, immune cells, vessels, and nerves embedded in a connective tissue matrix. The simplest experimental approximation to the in vivo situation is to co-culture prostate cancer cells and stromal cells in monolayer or double layers. Several techniques are available to culture thin tissue slices of the cancer tissue as ex vivo explants [34, 35]. The advantage of this system is that it preserves most of the features of the tissue architecture as well as its heterogeneity, although it is difficult to maintain and propagate the cultures for more than a few weeks. Ex vivo explants can also be useful for hormone and drug testing. This model also has potential usefulness in designing personalized medicine and in experiments that assay morphological or signal pathway changes in a tissue context. Many models recapitulate selected aspects of cancer growth in 3D or microenvironment conditions. Gels consisting of extracellular matrix substances, such as Matrigel, Geltrex, and collagen, or alternatively synthetically bioengineered scaffolds may support 3D growth of both benign and malignant prostate cells. Several techniques are available to support 3D spheroid growth of prostate cells with or without extracellular matrices. When grown on surfaces with ultralow attachment, prostate cells tend to form spheroids or prostaspheres resulting in the enrichment of cells with stem cell features [20]. Spheroids grown either in extracellular matrix or in ultralow attachment plates or as hanging drops may all reproduce the nutrition, oxygen, and pH gradients that are found in cancer tissues that outgrow their blood supply [35].
Organoid Cultures
The basis of organoid cultures is the availability of a matrix that supports 3D growth in vitro and an essential cocktail of compounds that modulate defined signal transduction pathways. In this way adult stem cells have been able to differentiate and self-organize into organoids that retain many features of the organ of origin [36, 37]. Organoid culture technology has significantly improved the success rate of establishing in vitro cultures of cancer cells [38]. Organoid technology has successfully generated benign epithelial prostate cultures [17, 39] and cultures that represent different subtypes of prostate cancer [40]. Prostate organoids have been established from metastatic cells, though establishment of in vitro cultures of primary prostate cancer cells remains a challenge [18, 40]. Organoid cultures have the additional advantage of being able to be propagated indefinitely and can be stored in liquid nitrogen as a living biobank. The experimental potential of prostate organoids has only begun to be exploited. Compared to monolayer cultures of stable cancer cell lines, organoid cultures may recapitulate more features of the original cancer although more experience needs to be gathered regarding the extent and for how many passages essential aspects of the original tumor can be preserved. The outcome will be very important for the use of organoids in personalized medicine in order to test drug sensitivity in vitro and to have an expandable antigen source that may be exploited in individualized immunoassays and dendritic cell-based vaccine development in the expanding immunotherapy field. In prostate cancer experimental research, the availability of organoids established from distinct cancer subtypes should facilitate investigation of critical molecular signaling pathways. Presently, microenvironment and immune interactions are not established in organoid methodology. Organoids may find their place between traditional cell cultures and animal models [38] (Fig. 19.3). In the design of animal experiments, it should be considered whether organoids could replace traditional cell lines for ethical, cost, and capacity reasons. The CRISPR-Cas9 genome editing system has recently transformed genome editing by its efficiency to knock out or knock in genes in cells and animals [43–45]. When used in combination with organoid technology, CRISPR-Cas9 technology may generate attractive experimental systems with systematic manipulation of single cancer relevant genes or combinations of genes [17, 41].


Fig. 19.3
Prostate cancer cell lines have most commonly been used for mouse xenograft models. Patient-derived xenografts (PDXs) have several advantages in retaining heterogeneity and features of original cancer tissue. Organoids can be established directly from prostate cancer tissues or via PDXs and vice versa. Patient-derived organoids (PDOs) have advantages when it comes to capacity and biobanking and several experimental types. In the panel to the right are shown short-term organoids grown from a primary prostate cancer core biopsy obtained at our Haukeland University Hospital from a patient in a Phase I clinical trial of cryoimmunotherapy against metastatic castration-resistant prostate cancer
Animal Models
Animal models are needed for better understanding of how cancer cells interact with the tumor microenvironment and with the entire organism during metastasis. Spontaneous development of prostate cancer is relatively common in dogs and some rat strains, but less common in mouse strains. Mouse models can be broadly divided into xenograft models and genetically engineered models [42]. While immunodeficient mouse strains are necessary for xenograft models, the current interest in immunotherapy has increased the demand for immunocompetent (syngeneic) mouse models and humanized mouse models.
Mouse Xenograft Models
Xenografts can be grown from any tumorigenic prostate cell culture or pieces of tissue. LNCaP, PC-3, and DU145 are the three most commonly used prostate cancer cell lines in xenograft models and have provided significant insight into disease biology [43]. Technically, the simplest approach is to inject tumorigenic cells subcutaneously with or without an intercellular matrix support, such as Matrigel. Tail vein injection or technically more demanding orthotopic injection into the prostate gland may be advantageous to answer questions related to metastasis and stromal invasion. Subrenal capsular injection of cells that are otherwise difficult to graft may be successful in part due to the high vascularization at this site. Tissue recombination models in which dissociated adult prostate cells are combined with embryonic urogenital sinus mesenchymal cells and implanted under the renal capsule have been useful for cell differentiation studies and epithelial-stroma interactions [44].
The choice of mouse strain, and in particular the extent of immunodeficiency, may also affect the efficiency of xenograft formation. The “nude” mouse was first established more than 40 years ago, and the advancement of immunodeficient mice to model human tumor growth has been reviewed recently [45].
Patient-Derived Xenografts
Although cell culture-based xenografts may provide useful information on cancer biology, these models have important limitations. In recent years the cancer research field has become highly aware of the importance of cancer cell heterogeneity which cannot be recapitulated by available cell culture-based xenografts. Patient-derived tumor xenografts (PDX) have emerged as a powerful technology: capable of retaining the molecular heterogeneity of their originating sample [46] and have been shown to exhibit genomic clonal dynamics reminiscent of their originating tumor sample [52]. In contrast to cell-based xenografts, PDXs have original tumor morphology [47]. PDXs have the potential to improve basic research on cancer subtypes with specific genomic lesions and could provide mouse avatars in personalized medicine drug evaluation and co-clinical trials [48]. Relevant stromal or immune drivers of malignant progression could, however, be missing [46] when immunodeficient mice are used as recipients.
Genetically Engineered Mouse Models (GEMMs)
Genetically engineered mouse models (GEMMs) allow the overexpression or deletion of selected genes in order to study the effect of defined pathways on carcinogenesis and tumor progression [42, 49–54]. Advantages compared to xenografted mouse models are that GEMMs are compatible with intact immune systems and stromal microenvironments of the same species as the tumor. The limitation of GEMMs is related to differences between mouse and men, regarding prostate architecture, cancer propensity in rodents, and small size of mice compared to humans. The TRAMP model is one of the most commonly used early transgenic models [55]. The model was generated by the introduction of a gene construct with the minimal rat probasin promoter driving expression of the SV40 virus early region. Androgen-responsive expression of the SV40 large T antigen inhibits p53 and Rb, and the small t antigen inhibits protein phosphatase 2A [51]. C57BL/6 TRAMP mice develop prostatic intraepithelial neoplasia (PIN) by 3 months of age. PIN typically progresses to neuroendocrine carcinoma within half a year with the lymph nodes and lungs as metastatic predilection sites. The model has been extensively used in preclinical testing and studies on carcinogenesis and tumor progression, but several limitations exist [51, 52]. DNA virus oncoproteins, such as SV40 large T antigen or human papilloma virus E6 or E7, have a special power to force cancer development, but these viruses have not been shown to induce prostate cancer. Furthermore, neuroendocrine differentiation is a feature of end-stage human prostate cancer and is seen in less than 2% of primary human cancer [6]. Furthermore, TRAMP mice rarely develop bone metastases, a common event in human patients.
The LADY model provides a modification compared to the TRAMP model by using a larger region of the rat probasin promoter to drive the SV40 large T antigen expression without small t antigen. Thereby a panel of less aggressive tumor lines, collectively referred to as LADY, was generated to study cancer-preventing factors and synergistic effects of different oncogenes [42, 49–51, 53].
A number of transgenic mouse models have since been generated to study signal transduction pathways involved in prostate carcinogenesis and progression in humans. Overexpression of the transcription factor MYC is prevalent in early prostate cancer [56], overexpression of which immortalizes primary prostate cells, induces PIN in normal prostate tissue, and stimulates growth of both early stage and CRPC [57, 58]. Mouse models of prostate cancer based on c-Myc have been reviewed [42, 49–52, 59].
The most frequently mutated single genes in primary prostate cancers are SPOP, TP53, FOXA1, and PTEN [60]. In a recent analysis of 333 primary prostate carcinomas, 15% harbored homozygous deletions spanning the PTEN locus [61]. The homozygotic knockout of Pten is lethal in mouse embryos, while heterozygotic knockout results in a spectrum of prostate phenotypes that, combined with other genetic lesions, such as p27Kip1-/- or Nkx3.1-/- mice, result in the progression to PIN and invasive prostate cancer [49]. The health problems associated with Pten knockout mice and the value of this genetic background in the study of additional genes and pathways in prostate cancer have encouraged the development of several conditional Pten knockout mouse models [42, 49–53, 59].
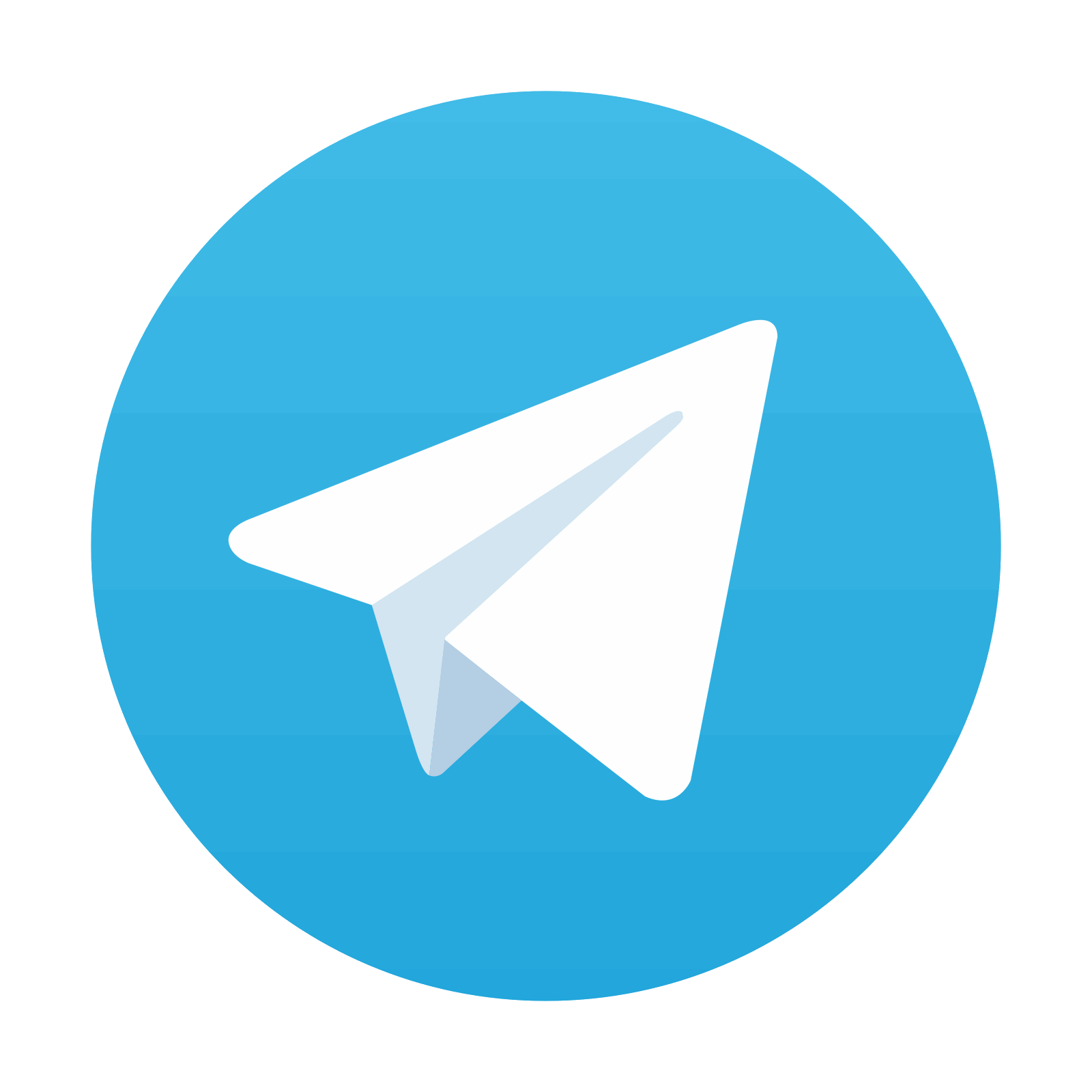
Stay updated, free articles. Join our Telegram channel

Full access? Get Clinical Tree
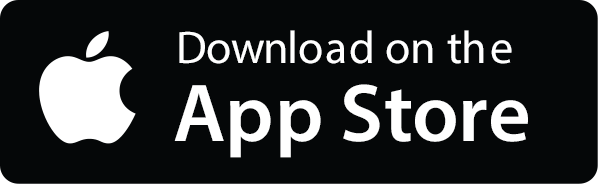
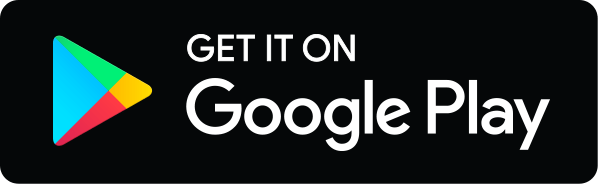