Chapter 7 Methods in natural product chemistry
In Chapter 6 we looked at the initial process in the selection of biomass (plant or microbe), its extraction and screening in different formats (high- and low-throughput screening), the isolation of the active components (bioassay-guided isolation) and the evaluation of the drug lead in clinical trials to the final drug. In this chapter we deal with the isolation process in more detail and cover the techniques that are used to isolate and characterize an active compound using chromatographic and spectroscopic techniques.
Bioassay-guided isolation
Bioassay-guided isolation is the physical process used to isolate biologically active chemicals from a natural source. Many of the chemicals described in Chapter 6 are from plant sources, but microbes are also an exceptionally valuable source of chemical diversity, in particular the filamentous bacteria (the Actinomycetes) of which the antibiotic-producing genus Streptomyces is the most widely studied for bioactive compounds. The fungi are also important and microbiologists spend time working in biota-rich environments such as the Amazon basin collecting, typing (identifying) and culturing samples for shipment back to the laboratory to be screened for bioactivity. As with plants, this process can be highly complicated, particularly in the identification of fungi, of which there may be potentially millions of new species waiting to be described in remote locations. This exercise is extremely worthwhile, as it is highly likely that new species will contain new chemistry that may have interesting bioactivity when fully screened. This will be particularly relevant for the Basidiomycetes, a large group of fruiting fungi that produce a mushroom cap (basidium) and are sometimes difficult to grow in solution fermentation.
Preparation and extraction
The most widely used method for extraction of plant natural products is Soxhlet extraction (Fig. 7.1). This technique uses continuous extraction by solvents of increasing polarity. The biomass is placed in a Soxhlet thimble constructed of filter paper, through which solvent is continuously refluxed. The Soxhlet apparatus will empty its contents into the round-bottomed flask once the solvent reaches a certain level. As fresh solvent enters the apparatus by a reflux condenser, extraction is very efficient and compounds are effectively drawn into the solvent from the biomass due to their low initial concentration in the solvent. The method suffers from the same drawbacks as other hot extraction methods (possible degradation of products), but it is the best extraction method for the recovery of big yields of extract. Moreover, providing biological activity is not lost on heating, the technique can be used in drug lead discovery.
If it is known that certain classes of compounds, such as acids or bases, are present in the biomass, they can be extracted using a tailored protocol. The most common group of natural products that are extracted in this manner are the alkaloids (see Chapter 6 and below), which are often present in plant material as salts. A brief outline of how these basic compounds may be extracted is as follows:
1. Alkaloids can be recovered from their salts by making the dry powdered plant material alkaline with aqueous ammonia. This leaves the alkaloids as free bases that are no longer ionic salts and are much more soluble in organic solvents such as dichloromethane or ethyl acetate.
2. This increased solubility in organic solvents allows partitioning of the free bases into ethyl acetate or dichloromethane, which can then be separated from the aqueous ammonia layer in a separating funnel as these solvents form immiscible layers.
3. The dichloromethane solution will contain the free bases, which can be extracted with aqueous acid, for example by extracting three times with 2 M hydrochloric acid, and the alkaloids will transfer from the organic phase to the aqueous phase as hydrochloride salts. The remaining dichloromethane layer can be tested using a specific colour test for alkaloids (e.g. Dragendorff’s reagent) to ensure that all of the alkaloids have been transferred to the acidic aqueous layer.
4. The acidic layer can then be basified, which results in the precipitation of the alkaloids (which are no longer salts and therefore no longer soluble in aqueous media) and can be extracted back into an organic solvent (ethyl acetate or dichloromethane).
Isolation methods
Ion-exchange chromatography
An example of the technique is shown in Fig. 7.2. 2,5-Dihydroxymethyl-3,4-dihydroxypyrrolidine (DMDP) from Lonchocarpus sericeus (Fabaceae) is a nematocidal polyhydroxylated alkaloid (PHA), and also inhibits insect α- and β-glucosidases. Compounds of this type are bases and form cations in acidic solutions. When added to a cation exchanger [e.g. Amberlite CG-120, which has a sulfonic acid bound to the resin which can exchange its proton (cation)], the DMDP cations are retained (bound) by the cation exchanger and protons are displaced. If the cation exchanger is then eluted with a solution containing a stronger cation such as (e.g. from 0.2 M NH4OH), then the DMDP cation is desorbed from the exchanger and is unbound and mobile. This affinity can be used to separate such alkaloids from acidic (anionic) or neutral components which would not be retained by the cation exchanger and may be washed from the resin by water.
Biotage™ flash chromatography
Biotage™ flash chromatography may be used for quick efficient separations. This employs pre-packed solvent-resistant plastic cartridges (Fig. 7.3), which contain the sorbent (silica, alumina, C18, HP-20, or ion exchange resin). These cartridges are introduced into a radial compression module (the metal cylinder in Fig. 7.3), which pressurizes the cartridge and sorbent radially. This results in a very homogeneous packed material (sorbent), reduces the possibility of solvent channelling when the system is run and minimizes void spaces on the column head.
Thin-layer chromatography
• It is cost-effective compared with instrumental methods and requires little training or knowledge of chromatography
• Easy scale-up from analytical to preparative mode with quick isolation of milligram to gram amounts of product
• Flexibility of choice of mobile and stationary phases
• A separation may be readily optimized to ‘zero in’ on one component and methods may be quickly developed
• Practically any separation can be achieved with the correct mobile and stationary phases
• A large number of samples may be analysed or separated simultaneously.
The major disadvantages of TLC are that:
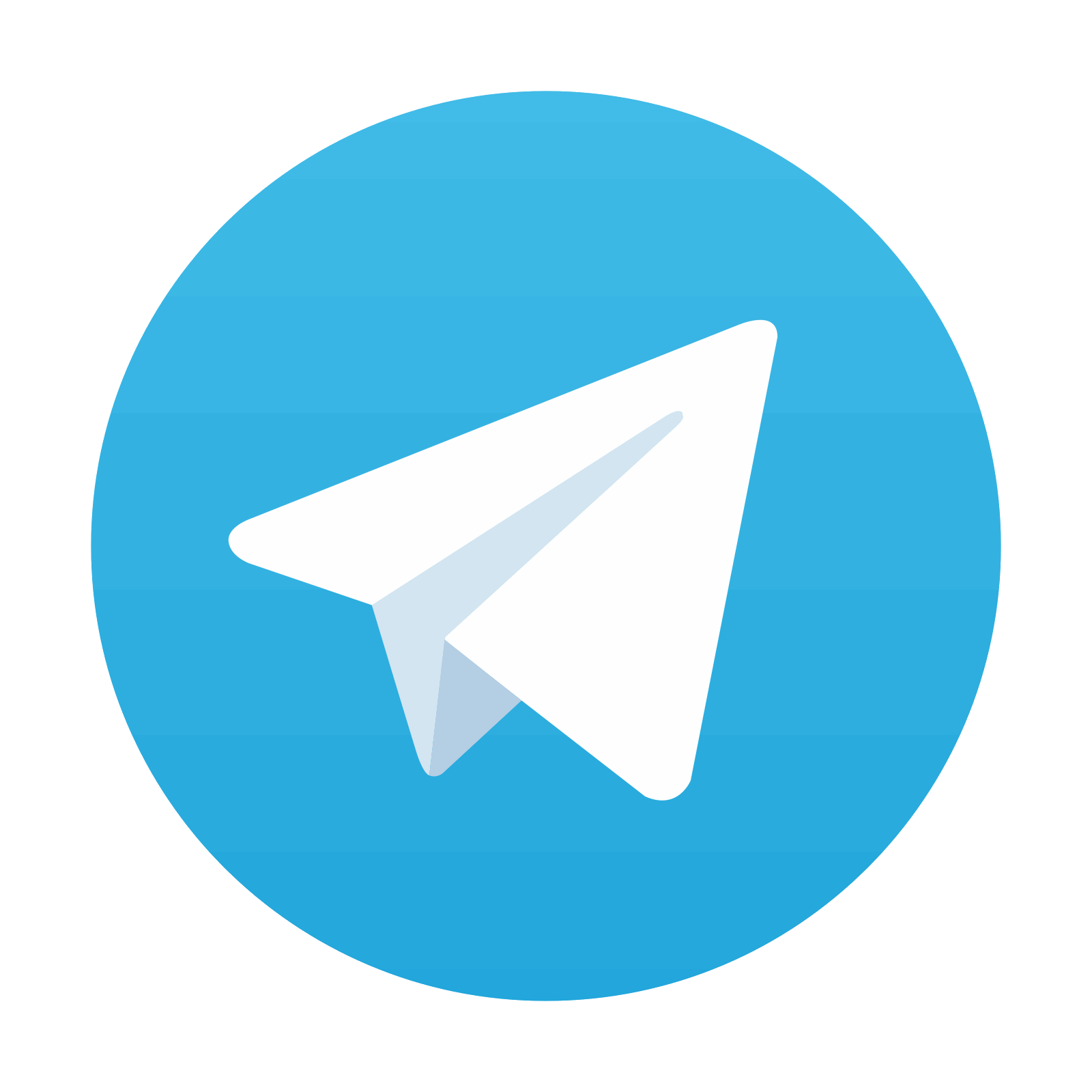
Stay updated, free articles. Join our Telegram channel

Full access? Get Clinical Tree
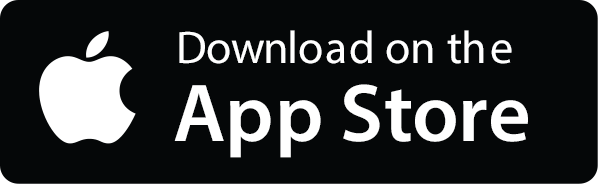
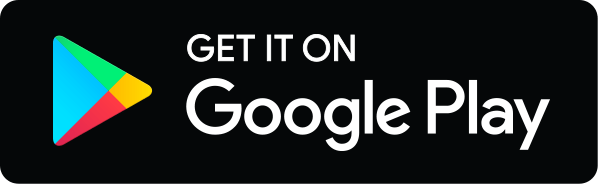