Chapter 17 General methods associated with the phytochemical investigation of herbal products
The phytochemical investigation of a plant may thus involve the following: authentication and extraction of the plant material; separation and isolation of the constituents of interest; characterization of the isolated compounds; investigation of the biosynthetic pathways to particular compounds; and quantitative evaluations. Parallel to this may be the pharmacological assessment of the separated components, which may, in some investigations, precede the characterization.
EXTRACTION OF PLANT MATERIAL
All plant material used should be properly authenticated, as much time and money can be wasted on the examination of material of doubtful origin. The choice of extraction procedure depends on the nature of the plant material and the components to be isolated. Dried materials are usually powdered before extraction, whereas fresh plants (leaves, etc.) can be homogenized or macerated with a solvent such as alcohol. The latter is also particularly useful for stabilizing fresh leaves by dropping them into the boiling solvent. Alcohol is a general solvent for many plant constituents (most fixed oils excepted) and as such may give problems in the subsequent elimination of pigments, resins, etc. Water-immiscible solvents are widely used—light petroleum (essential and fixed oils, steroids), ether and chloroform (alkaloids, quinones). The extraction of organic bases (e.g. alkaloids) usually necessitates basification of the plant material if a water-immiscible solvent is to be used; for aromatic acids and phenols acidification may be required. Extraction itself may be performed by repeated maceration with agitation, percolation or by continuous extraction (e.g. in a Soxhlet extractor, Fig. 16.2). Special methods for volatile oils, such as the enfleurage process, are considered in Chapter 22. Ultrasound may enhance the extraction process for some plant materials and the BP uses this in the preparation of a 50% ethanolic solution of opium for the assay of alkaloids and in the assay procedure of Agnus Castus. Its use has been studied for the extraction of atropine from Hyoscyamus muticus using various solvent systems (A. Djilana and B. Legseir Fitoterapia, 2005, 76, 148).
Supercritical fluid extraction
Piper nigrum muntok (superior aroma of oil; yield 2.8 per cent volatile oil compared with 0.6 per cent by steam distillation); rose petals (product richer in relevant fragrance compounds compared with steam distillation); rosemary (aroma more closely resembles plant fragrance than distilled oil); also studied: angelica root, celery, coriander, Illicium verum, Maytenus illicifolia, pimento
For additional information on the method, consult the ‘Further reading’.
SEPARATION AND ISOLATION OF CONSTITUENTS
Adsorption chromatography
Various substances may be used as adsorbing materials; alumina is the most common and other materials include kaolin, magnesium oxide, calcium carbonate, charcoal and sugars.
Partition chromatography on paper
The quantity of substance present determines the size of the spot with any one solvent and can be made the basis of quantitative evaluation. Also, the separated components of the original mixture can be separately eluted from the chromatogram, by treating the cut-out spots with a suitable solvent, and then determined quantitatively by some suitable method—for example, fluorescence analysis, colorimetry or ultraviolet adsorption. Drugs so evaluated include aloes, digitalis, ergot, hemlock, lobelia, nux vomica, opium, rauwolfia, rhubarb, broom, solanaceous herbs and volatile oils.
High-performance liquid chromatography (HPLC)/high-speed LC
The columns are costly and it is usual to employ a small precolumn containing a cartridge of packing material to remove adventitious materials which might otherwise damage the main column. Normal flow rates of eluate are 2–5 ml min−1 but can be up to 10 ml min−1, depending on the diameter of the column and the applied pressure. The apparatus is suitable for all types of liquid chromatography columns (adsorption, partition by the use of bonded liquid phases, reversed phase, gel filtration, ion exchange and affinity). The arrangement of such an apparatus, suitable for use with two solvents and giving graded elution, is illustrated in Fig. 17.1. Detection of the often very small quantities of solute in the eluate is possible by continuous monitoring of ultraviolet absorption, mass spectrum, refractive index, fluorescence and electrical conductance; nuclear magnetic resonance can now be added to this list. To improve detection, solutes may be either derivatized before chromatography (this technique can also be used to improve separations) or treated with reagents after separation (post-column derivatization). A transport system for monitoring is commercially available; in this a moving wire passes through the flowing eluate (coating block) and the dissolved solute, deposited on the wire, is pyrolysed and its quantity automatically recorded. It will be noted that, for any particular fractionation, some detector systems would be selective for certain groups of compounds and others would be universal.
< div class='tao-gold-member'>
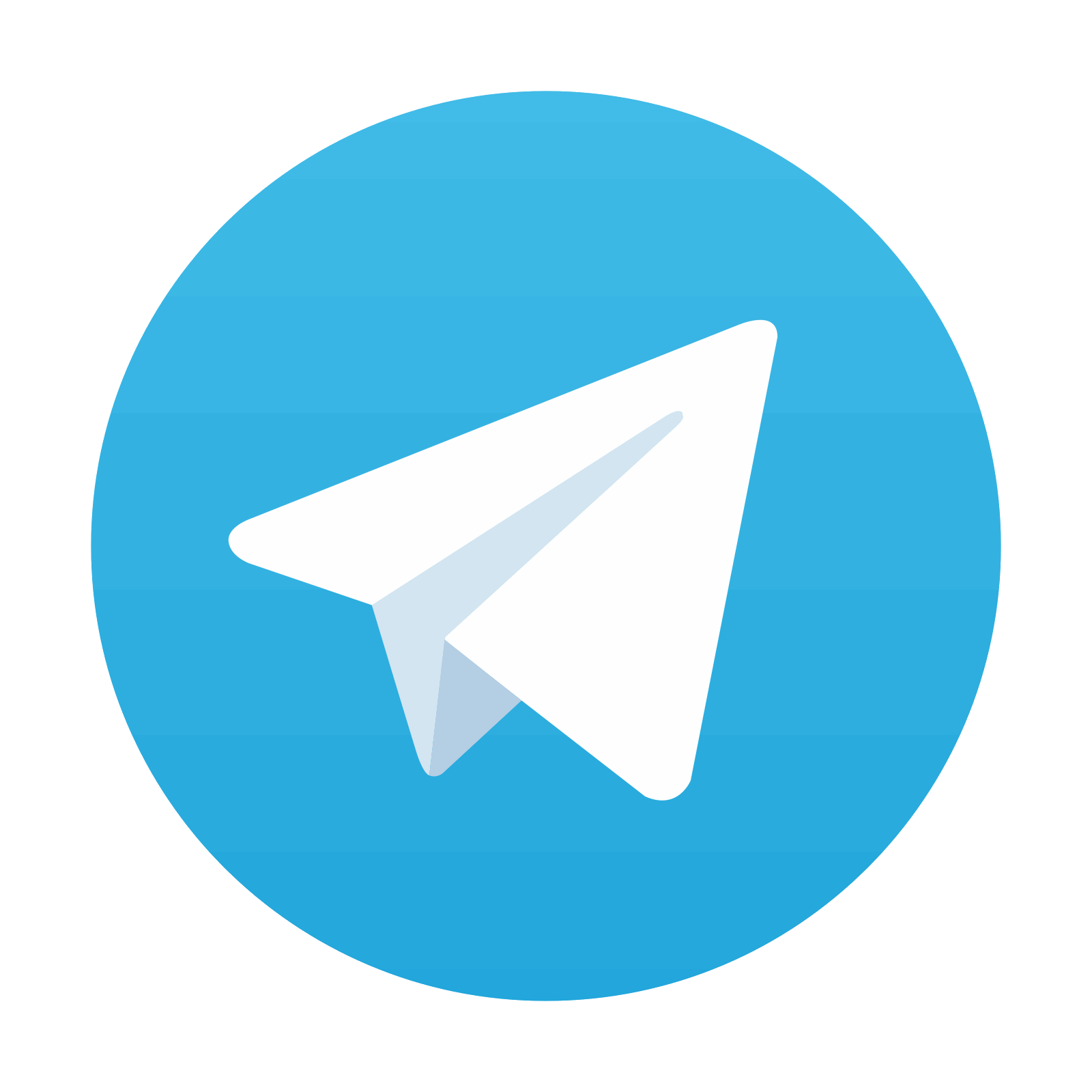
Stay updated, free articles. Join our Telegram channel

Full access? Get Clinical Tree
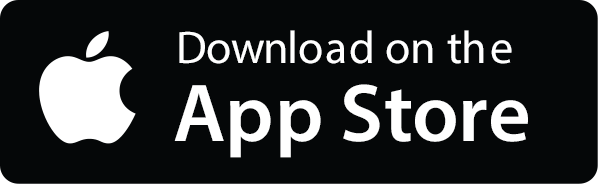
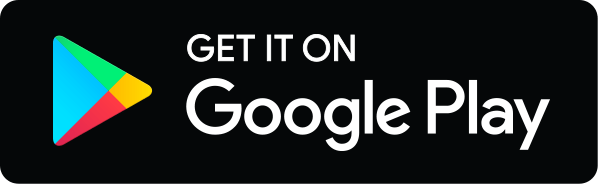