There are many cases of new herbal drugs for which no TLC-related information is found in the literature. In other cases, the official methods or other established procedures are not adequate to answer a given analytical question, and the usual optimization attempts (HPTLC instead of TLC plates, improved sample application, changing chamber condition, chemical derivatization, multiple detection, etc.) have failed. This is the time when it becomes necessary to develop an HPTLC method from scratch. Method development always begins with clarification and complete understanding of the analytical goal. For example, methods targeting monitoring the content of known constituents of herbal drug preparations or herbal medicinal products during stability tests may be different from those developed for distinguishing suitable raw material from adulterants. Chapter 5 is divided in two sections. The first covers theoretical aspects including some common models for optimizing the mobile phase. In the second section, practical aspects are discussed emphasizing a systematic trial-and-error approach, which has proved helpful in our laboratory. ♦ Theoretical Aspects General Considerations HPLC and GC separations usually take place in an equilibrated system of stationary and mobile phase. The situation is different in TLC. During separation, such equilibrium is approached but in many cases only partially reached. As a consequence, predictability of the chromatographic result is rather limited, and method development remains a widely empirical process. Theoretical models or computer-aided strategies provide satisfactory solutions applicable to “real” analytical problems only for defined systems with a strictly controlled set of parameters. TLC methods are different also in another sense. Because of the off-line character of the technique and the great choice of parameters for each individual step, there are almost unlimited possibilities for combinations. In HPLC, once settled on a given column and, for example, DAD detection, method development is focused on working out a suitable mobile phase gradient. Besides plate, mobile phase, and densitometric multi-wavelength detection, in TLC we can choose and define additional parameters: Each parameter can affect the result if all others remain constant. On the other hand, a change in one parameter can easily be compensated by changes in another. At the end, there are several possible combinations that may give different but adequate chromatographic results. From a practical point of view, method development in TLC can be quite simple and straightforward, as long as it is performed in a structured trial-and-error approach. When the analytical goal is accomplished, method development must stop, otherwise we waste time searching for that “better” alternative, which always will be just “different.” Defining the Analytical Goal Starting point of any method development is a clear understanding of the analytical goal. It is a good idea to think about it thoroughly and write down precisely what the method must, should, or could do. In other words, before we begin we define acceptance criteria against which we can later validate the method (see Chapter 6). Assume we have been charged with the development of a method for quality control of a certain botanical. Initially, we should ask questions like: In the ideal case, the final method can do everything, but in reality it is often easier to develop two or more dedicated and complementary methods. Models for Mobile Phase Selection For a moment, we want to set aside the individual steps of a TLC method and look briefly at some models that illustrate the selection of suitable mobile phases. From Chapter 2, we remember that there are two important solvent properties to consider: Many publications deal with theoretical concepts for method development in TLC. Even software-based solutions like “ChromSword” and “LSChrom” have been described.1Unfortunately, they don’t seem practical for the analysis of botanicals because too little is known about the analytes. Figure 5–1 The PRISMA Model As far as semiempirical concepts for TLC are concerned, the PRISMA model2 and the “four-solvent approach”3 are particularly well established. The PRISMA Model The PRISMA model (Fig. 5–1), developed for TLC by Nyiredy and co-workers, derives its theoretical basis from Snyder’s selectivity triangle (see Fig. 2–29 on page 53). In the first step, 8 to 10 neat solvents are selected as mobile phase for separation. They should represent all selectivity groups. Of those, the three solvents offering best selectivity are taken for construction of the prism in the second step. The corners of the triangular base (points 1, 2, 3) represent the different selectivity of the individual solvents. The height at each corner is equivalent to their P values. The regular portion of the prism is used for samples of low and medium polarity. In any triangle congruent to the base, all solvent mixtures are equi-eluotropic. That means the average RF values for the sample components obtained with those mixtures will be about the same. By mixing them with a certain amount of hexane (Pʹ=0) in the third step, the stronger solvents (points 4 and 5) are reduced to arrive at the strength of the weakest solvent (points 7 and 8 to the height of point 6). For samples of very low polarity, it may be necessary to reduce the strength of all three solvents. In the fourth step, selectivity of the mobile phase system is varied by changing the proportions of the adjusted “corner” solvent until resolution is satisfactory. This is done beginning with the center point (9) and then moving to points close to the corners (6, 7, and 8). In the irregular top portion of the prism, optimization can be performed for very polar samples. Only small changes should be made to the mobile phase composition in this region, because they will also affect the average RF value of the sample. If during the first step the solvent strength of the neat solvents is not sufficient to yield RF values between 0.2 and 0.8, small proportions of modifiers such as acids, bases, or water can be used to deactivate the stationary phase. These modifiers are represented as pedestal of the prism. If during this optimization process no satisfactory separation is obtained, a new stationary phase or different solvents have to be chosen. For separations in reversed-phase systems, the PRISMA model can also be employed. In this case, solvent strength is adjusted with water (ST=0). One of the principal drawbacks of the model is the need for extensive calculation, which only defines the strength of a given mixture, but cannot predict the required proportions for a given strength or the corresponding RF value. The Four-Solvent Approach The four-solvent approach is based on solvent strength (ε°) and selectivity with respect to localization on the adsorbent. Three types of solvents are used for optimization: One of the three solvents can also be localizing protic (e.g., isopropanol). In any case, the fourth required solvent is “diluting” (e.g., heptane or octane). It is used to reduce the solvent strength. FC-113 (1,1,2-trifluorotrichloroethane) because of its ideal miscibility is also a suitable diluting solvent. Unlike the PRISMA model, which first evaluates selectivity, the four-solvent approach starts with finding the appropriate solvent strength. To do this, several mixtures representing different solvent strengths have to be prepared upfront. It is possible to have mixtures covering ε° values from 0.00 to about 0.80 in increments of 0.05 to 0.10 (Table 5–1). Once prepared, these solvent mixtures are stable and can be stored for future use. With the development of a couple of plates (selection of only a few different ε°), the suitable solvent strength affording RF values between 0.3 and 0.5 can be found. In the next step, two solvents of the same strength but different selectivity are taken.
5
Method Development
ε° | Solvent mixture | Proportions |
---|---|---|
0.00 | Octane | 100 |
0.05 | DCE, octane | 3.5:96.5 |
0.10 | DCE, octane | 10:90 |
0.15D | DCE, octane | 18:82 |
0.20 | DCE, octane | 32:68 |
0.25 | DCE, octane | 58:42 |
0.30 | DCE | 100 |
0.35 | ACN, FC-113 | 8:92 |
0.40 | ACN, FC-113 | 24:76 |
0.45 | ACN, FC-113 | 52:48 |
0.50 | ACN, FC-113 | 88:12 |
0.60 | ACN | 100 |
0.70 | MeOH, DCE | 28:72 |
0.80 | MeOH, DCE | 52:48 |
Adapted from Geiss F. Fundamentals of Thin Layer Chromatography. Heidelberg: Hüthig; 1987.
They are made by dilution of so-called Master Mixtures with octane or FC-113. Further details and composition of Master Mixtures can be found in Ref. 4
At that point, we are back to a triangle representing three selectivity types. By changing the proportions of the three involved solvents, the separation of the sample can be optimized (Fig. 5–2). Within the triangle, any mixture can be used without changing the solvent strength.
Figure 5–2 The four-solvent approach (schematic). Points A, B, and C represent equi-eluotropic solvents (or solvent mixtures) of different selectivity.
The four-solvent approach requires extensive preparation but is otherwise quite simple. It can be applied to silica gel and polar bonded phases as well. The principal shortcoming is that at higher solvent strength (ε° greater than 0.4), the choice of suitable solvents is limited.
Method Development in Practice
Method development for the analysis of botanicals can be summarized with the scheme presented in Fig. 5–3. For the remainder of the chapter, we will refer back to the individual positions of this scheme by their alphanumeric designations (e.g., A1, A2B, C).
Initial Decisions
Developing a method for use in the analysis of botanicals is no problem! Referring to Fig. 5–3, we either know what we want to analyze or we don’t. In the first case (A1), we can try out already established methods for the given substance class, such as flavonoids, triterpene saponines, and so forth, and optimize the result. In the second case (A2), we start from scratch. That will be the focus of the following sections.
Unfortunately, there is usually little information about the botanical at hand. Because method development will go smoother the more we know, it is recommended to research the literature for anything published about the chemistry of the sample. TLC can analyze many different substance classes, so it helps if we find out for which the method will be developed. If that decision cannot be made, several additional tests have to be performed.
Figure 5–3 Method development, schematic.
Before we begin, we need to remember a few things:
- The zone capacity of the TLC system is limited. That means baseline resolution can be obtained for only about 10 sample components during a single development. This is important for quantitative determinations.
- TLC is not suitable for the separation of large biomolecules such as proteins and polysaccharides.
- TLC is an off-line process and although we optimize one step, all others are kept constant.
- The TLC process includes two drying steps, following sample application and chromatogram development. Both can affect the sample. Highly volatile samples such as essential oils can partially evaporate from the layer and may be difficult to quantify. Samples sensitive to oxidation require special experimental conditions to avoid decomposition.
Sample Preparation and Screening Methods
Let us begin with the sample preparation, which particularly for qualitative analyses can be reduced to a minimum. A good starting point would be sonicating 0.5 g of powdered sample with 5 mL methanol. Methanol usually extracts a broad spectrum of compounds, but if we don’t know what to expect, we can set up a complete screening (A2a) with the 15 solvents in Table 5–2.
After centrifugation or filtration, 5μ L of each extract can be applied as bands onto five 20× 10–cm HPTLC plates with silica gel 60 F254 as stationary phase following the general SOP (Appendix B). We need to find a mobile phase that gives at least some separation so that we can determine a suitable detection mode (A2b). Each of the following five systems evaluates a different polarity segment of the sample:
- Toluene, ethyl acetate (95:5) for nonpolar compounds and essential oils.
- Chloroform, methanol, water (70:30:4) for saponines and lignanes.
- Ethyl acetate, acetic acid, formic acid, water (100:11:11:27) for flavonoids.
- Acetonitrile, water, formic acid (30:8:2) for amino acids.
- 1-Butanol, acetic acid, water (7:1:2) for polar compounds.
Neutral | Acetone | Acidic |
Heptane | Ethanol | Methanol–acetic acid (9:1) |
Toluene | Methanol | Water–acetic acid (9:1) |
TBME | Ethanol-water (7:3) | Basic |
DCM | Methanol-water (8:2) | Methanol-ammonia 25% (8:2) |
Chloroform | Water | Water-ammonia 25% (8:2) |
For detection after development with systems 1, 2, and 5, sulfuric acid or anisaldehyde reagent work well. Natural Products reagent is suitable for system 3, and ninhydrin reagent should be used for system 4. It is important to evaluate each plate prior to evaluation under UV 254 nm and UV 366 nm. After derivatization, evaluation should be performed under white light and UV 366 nm. A list of common reagents and their preparation is found in Appendix E.
Figure 5–4 illustrates the process using a sample of an unknown botanical. At the end we have one or more combinations of extraction and detection, which can be taken further into the actual method development.
Selecting Stationary Phase and Separation Principle
Silica gel is by far the most widely used adsorbent. In most cases according to Fig. 5–3 (A2b), it will have already proved suitable and it should be the first choice for most samples of the type A1.
Let us recall some general aspects:
- Although it might be somewhat cheaper to work on TLC material, we highly recommend using HPTLC silica gel 60 F254.
- Results obtained on precoated plates from different manufacturers are not always comparable. We need to settle on one material before method development begins.
- For initial steps of method development, plates do not have to be prewashed.
- It is important to record relative humidity during method development.
Figure 5–4 Initial investigations on an “unknown” botanical. MP:(A) toluene, ethyl acetate (95:5); (B) chloroform, methanol, water(70:30:4); (C) ethyl acetate, acetic acid, formic acid, water (100:11:11:27); (D) acetonitrile, water, formic acid (30:8:2);(E) 1-butanol, acetic acid, water (7:1:2). D:(A, B, and E) sulfuric acid reagent, white light; (C) NP PEG reagent, UV 366 nm; (D) ninhydrin reagent, white light. Extraction solvent: Track 1, heptane; track 2, toluene; track 3, TBME; track 4, DCM; track 5, chloroform; track 6, acetone; track 7, ethanol; track 8, methanol; track 9, ethanol-water (7:3); track 10, methanol-water (8:2); track 11, methanol—acetic acid (9:1); track 12, methanol-ammonia 25% (8:2).For details, see Box 5–1.
(E) 1-butanol, acetic acid, water (7:1:2). D:(A, B, and E) sulfuric acid reagent, white light; (C) NP PEG reagent, UV 366 nm; (D) ninhydrin reagent, white light. Extraction solvent: Track 1, heptane; track 2, toluene; track 3, TBME; track 4, DCM; track 5, chloroform;track 6, acetone; track 7, ethanol; track 8, methanol; track 9, ethanol-water (7:3); track 10, methanol-water (8:2); track 11, methanol—acetic acid (9:1); track 12, methanol-ammonia 25% (8:2).For details, see Box 5–1.
There are several reasons for which it may be necessary to consider other stationary phases:
- At the end of step C in Fig. 5–3, the analytical goal is not achieved on silica gel.
- No or very little migration might be observed in step A2b.
- The knowledge about the sample’s constituents in step A1 may suggest a separation mode other than adsorption chromatography.
Box 5–1 How to Treat an Unknown Botanical
Many times nothing or very little is known about the chemistry of a given botanical. Before method development can begin, we need to find out how to extract and detect compounds that provide a characteristic fingerprint. Starting with simple sonication using solvents of increasing polarity in parallel, a set of samples is obtained that can be analyzed with several chromatographic systems. In Fig. 5–4, the sample was extracted with 12 solvents (water, water–acetic acid, and water-ammonia were incompatible with the sample). The first solvent system illustrates that nonpolar compounds are equally well extracted regardless of solvent polarity up to methanol (track 8). The polar compound profiles separated with the other, polar solvent system require at least methanol for completeness. In all systems, the presence of water, acid, or base during extraction affects the “clarity” of the fingerprint negatively. As a conclusion, we would select methanol as the extraction solvent and develop a method focusing on the medium polar compounds as seen in Fig. 5–4B.
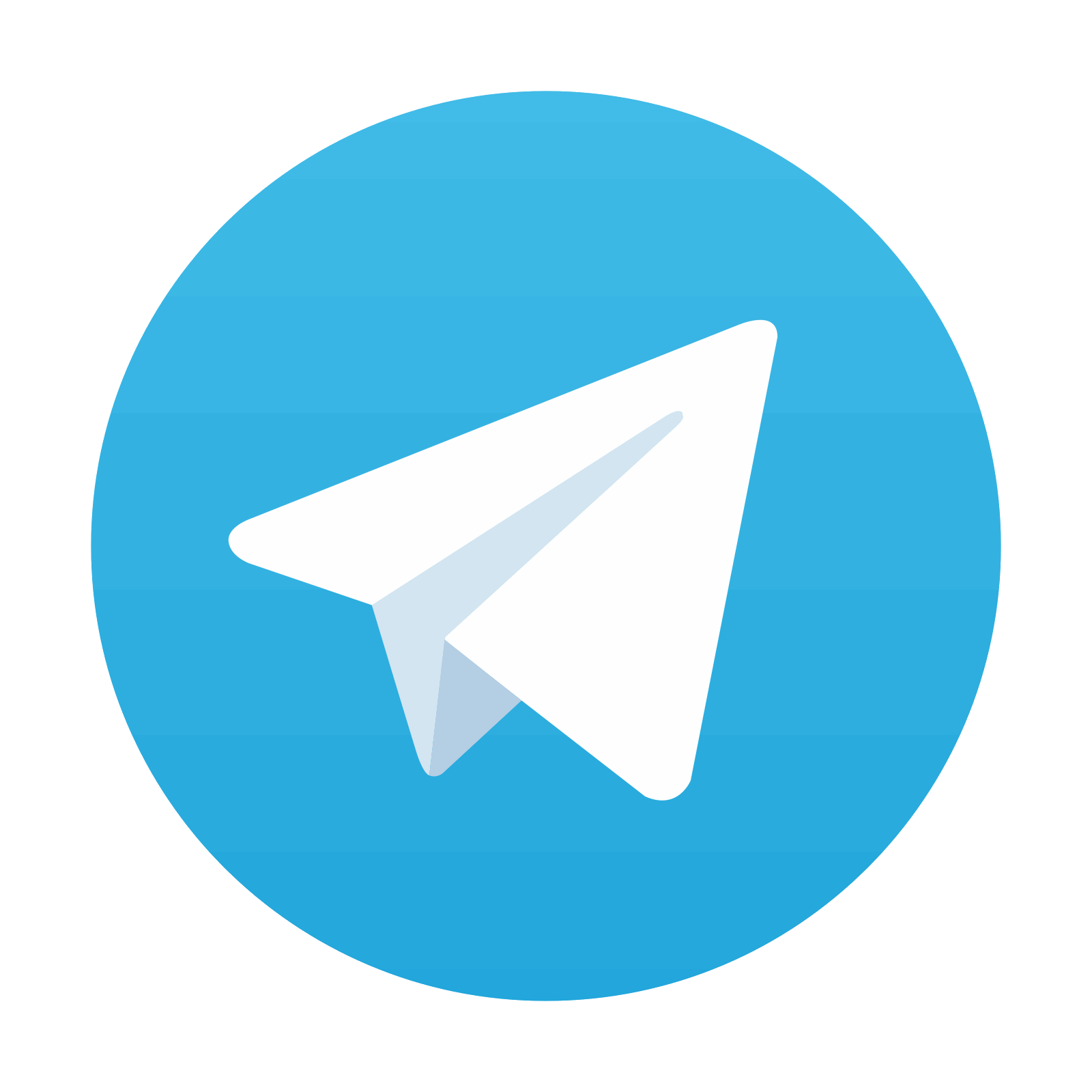
Stay updated, free articles. Join our Telegram channel

Full access? Get Clinical Tree
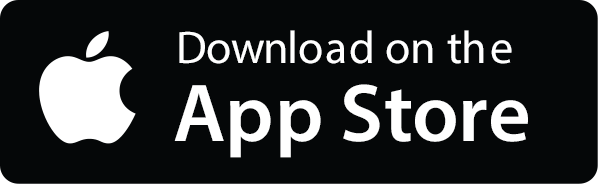
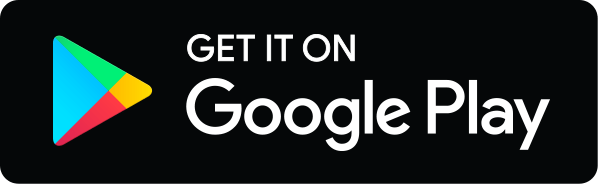