Ahmad Bashir The mechanism of action of bariatric surgery has evolved over the past three decades. Since the evolution of bariatric procedures, they have been classified into restrictive and malabsorptive, delineating the mechanism they worked through: decreasing the amount of food and calories one could eat, or in the latter, one could absorb, or a combination of both. This stemmed from the notion that obesity is caused by excess consumption of calories without burning the excess off, resulting in storage of the excess in the form of fat. It is actually quite surprising that this definition exists to this date, as this definition has evolved and bariatric surgery played an integral role in the understanding of the complexity of obesity as a disease, as discussed below. Still, the initial design of the procedures revolved around decreasing the intake of food or decreasing its absorption. Though the malabsorptive effects of earlier procedures were obvious, decreased caloric intake and malabsorption of fat, Halmi et al. in 1980 managed to study the positive emotional side of bariatric surgery (gastric bypass and jejuno‐ileal bypass [JIB]) versus dieting patients. They showed a vast change in bariatric surgery patients from chaotic excessive food intake to ‘normalisation’ of eating patterns, decreased snacking and binge eating, with increased ability to voluntarily stop eating without any added effort as opposed to dieting patients. All these findings to them suggested that bariatric surgery produced weight loss through ‘major changes in the biology of the obese person’. They stopped to ask the question as how both a more restrictive and a more malabsorptive produce the same satiety effect, proposing that JIB effects were humoral and gastric bypass effects were anatomical. The humoral known effects back then were attributed to increased levels of enteroglucagon, which was thought to increase satiety. What is even more interesting, the authors raised the issue that these procedures may affect the ‘set point’ for weight regulation in the hypothalamus raised in other animal research at the time, as early as 1966, and in a detailed review report by Keesey in 1978. While Halmi et al. briefly mentioned anatomical effects as reason to why satiety occurs in gastric bypass, Greenstein et al. in 1994 reported that enforced behaviour modification was the reason for weight loss, and patients ate less to avoid vomiting in gastric restrictive procedures. They documented the stark comparison between patients on diet versus vertical banded gastroplasty (VBG). Surgical patients had significantly less hunger, more will power to stop eating, and the main reasons documented were the feeling of discomfort after meals with fear of vomiting, suggesting the restriction of the pouch as the mechanism for this behaviour change. Nevertheless, with time, plenty of unknowns were unveiled. Although still relied on the concept of reduction of calorie intake, in 1995, Pories et al. in their landmark ‘Who would have thought it?’ paper reported the outcomes of Roux‐en‐Y gastric bypass (RYGB) series in 608 patients, and noted that in patients with diabetes or impaired glucose tolerance (IGT) (298 patients [49%]), euglycemia occurred prior to any significant weight loss, and any therapy for hyperglycaemia was usually discontinued at within one week of the operation. Euglycemia continued in 91% of patients, while 9% remained hyperglycemic. When analysing causes for persistence of their hyperglycaemia, the breakdown of the staple line of the non‐divided gastric pouch, with the restoration of normal passage of food, was seen in 37% of patients who did not improve, while the rest were older and had diabetes for a longer period of time. They hypothesised that bypassing the hormonally active antrum, duodenum and proximal jejunum, presenting undigested food to mid‐jejunum, with delay in the transit of food from the small pouch to the small intestine, may all play a role in mechanism of action of gastric bypass in achieving euglycemia, on top of calorie and carbohydrate reduction. When matching six of RYGB patients with a stable weight at least two years after the procedure to six matched controls, Hickey et al. reported, in 1998, less leptin hormone levels overall per unit of fat mass and higher insulin sensitivity in surgical group as opposed to controls, thus excluding weight loss as a variable in the explanation of how euglycemia is achieved and maintained after gastric bypass, and stressing the potential role of the bypassed foregut in euglycemia, and connected it to incretin hormone release from ingesting oral glucose, mainly glucagon‐like peptide 1 (GLP‐1), which is expressed distally (large bowel and ileum), and gastric inhibitory peptide (GIP), which is expressed proximally in duodenum and jejunum theoretically without actual measurement of these hormones. Cummings et al. followed in 2002 with measuring 24‐hour ghrelin hormone profile in RYGB participants, diet‐induced weight‐loss participants with matched controls with obesity and normal weight group. With better weight loss in surgical group at 36% excess body mass loss, compared to 17.4% in diet group, and 17% in obesity controls, the ghrelin hormone levels were much reduced in the surgical group (3.5 times lower than obesity controls) as opposed to increased levels in diet‐induced weight‐loss group at six months after weight loss, compared to before weight loss. Moreover, ghrelin hormone pattern lost the diurnal variation and meal‐related change in surgical group compared to other groups. This study confirmed that adaptive mechanism of ghrelin induced hyperphagia after diet‐induced weight loss, limits the amount of weight lost through diet. In addition, it introduced the concept that ghrelin suppression may play a role in weight reduction and satiety after gastric bypass beyond the effects of a restrictive gastric pouch (Figure 14.1). Figure 14.1 24‐hour plasma ghrelin level in RYGB and controls. Source: From Cummings et al. (2002) / with permission of Wolters Kluwer Health, Inc. Le Roux et al. followed, in 2006, the comparison of lean or obesity‐matched controls, with patients who underwent RYGB and gastric banding 6–36 months prior to the study. They measured peptide YY (PYY), GLP‐1, pancreatic polypeptide (PP) and ghrelin before meals and postprandial at an interval of 30 minutes up to 3 hours, together with assessing insulin response and glucose levels. Insulin level pronouncedly peaked in RYGB patients early after meals, corresponding to early increase in both GLP‐1 and PYY, in comparison to lower levels in lean controls, and even lower in obesity controls and gastric banding patients. Ghrelin levels were lowest in RYGB, but no statistical significance was found compared to gastric banding or obesity controls, and were highest in lean controls. Authors also investigated PYY effect on rodent rats’ food intake, which increased when PYY was blocked with neutralising antibody in bypass rats, while decreased in sham rats with exogenous PYY administration. These findings suggested that PYY and GLP‐1 had the highest effect on weight loss, satiety and glycemic control after RYGB. PP release was similar amongst all groups (Figure 14.2). Figure 14.2 (a) Difference in insulin, GLP‐1 and PYY responses in lean ( Source: Republished with permission of Wolters Kluwer Health, Inc., from le Roux et al. (2006), permission conveyed through Copyright Clearance Center, Inc. All the above findings steered the bariatric community into a newer direction of further research and acknowledging more powerful mechanisms of bariatric procedures than restriction and malabsorption. American Society of Bariatric Surgery (ASBS) became American Society of Metabolic and Bariatric Surgery (ASMBS) in 2007, while International Federation for the Surgery of Obesity (IFSO) added ‘and metabolic disorders’ at the end of its name. More research continued to correlate the science of gut hormones to satiety, weight loss and metabolic improvements. But, what was the role of calorie restriction? Lips et al. studied five groups: very low‐calorie diet (VLCD) with DM, RYGB with DM, gastric banding with impaired glucose tolerance (IGT), RYGB with IGT and normal weight controls. All groups received an oral glucose tolerance test before and two to three weeks after intervention (surgery or diet initiation). Several blood draws were taken over three hours after drinking a high‐calorie drink for GIP, GLP‐1, PYY and ghrelin together with insulin and glucose levels. To everyone’s surprise given the previous data, RYGB did not improve glucose metabolism any more than VLCD did in patient with DM. RYGB did cause gut hormone alterations that were observed previously: increased GIP, GLP‐1 and PYY, decreased ghrelin with enhanced insulin response (marked increase in postprandial insulin compared to VLCD or banding), but the reduction of fasting and postprandial glucose levels were equivalent to VLCD, suggesting that caloric restriction and reduction was the reason for early improvement in glucose homeostasis. In fact, HOMA‐ir was reduced more in VLCD in comparison to RYGB in that study, raising more questions about the gut hormones known on the impact of glucose homeostasis (Figure 14.3). Figure 14.3 Glucose and insulin concentrations during mixed meal tolerance test before (open squares) and after intervention (closed squares) in IGT‐GB group (a), IGT‐RYGB group (b), DM‐RYGB group (c), DM‐VLCD group (d) and AUC glucose and insulin before (white bars) and after (black bars) intervention (e), suggesting restrictive component playing a major role in glucose homeostasis early after the operation. Source: Republished with permission of John Wiley & Sons, from Lips et al. (2014), permission conveyed through Copyright Clearance Center, Inc. Fast forward to today and we now have more knowledge of additional gut hormones that influence the changes seen after various bariatric procedures. Neilson et al. compared the hormonal response in patients undergoing RYGB and sleeve gastrectomy (SG) with mandatory pre‐operative 8% weight loss prior to both interventions. They studied glicentin and oxyntomodulin, recently discovered gut hormones, which are co‐secreted from L‐cells together with GLP‐1 and have longer half‐lives than GLP‐1. They correlated hormone basal and postprandial levels with variations in weight loss and appetite, especially energy‐dense foods. They reported higher glicentin, oxyntomodulin, GLP‐1 and PYY levels in RYGB as opposed to SG, while SG had lower more suppressed ghrelin level. Of note, ghrelin increased in both groups pre‐operatively with mandatory pre‐operative weight loss, confirming the effects of diet on increasing its level. Ghrelin level remained at the same elevated pre‐operative level post‐operatively in RYGB, while decreased in comparison in SG. Basal levels of glicentin and oxyntomodulin, on the other hand, increased only after RYGB, while basal GLP‐1 and PYY levels did not increase in RYGB or SG. This basal increase at 6 months, predicted successful weight loss in patients at 18 months’ follow‐up. It also was associated with decrease energy‐dense food intake as opposed to other hormones. Authors estimated that both had direct effects on weight loss accounting for 62–64%, while 36–38% was weight loss due to their effects on decreasing high‐energy food intake. Postprandially, only GLP‐1 increased in both RYGB and SG, with higher increase in RYGB. Meanwhile, PYY, glicentin and oxyntomodulin increased postprandially only in RYGB, while remaining same in SG. Ghrelin remained reduced in both, more so in SG. Combining the analysis of all five hormones at 3 and 6 months, the authors were able to predict 60% of the variability in weight‐loss patterns at 18 months. They established a synergistic effect of these hormones on weight loss and suggested using these levels early to be able to predict patients who will need more guidance to achieve better results. Only glicentin and oxyntomodulin basal and postprandial increases correlated with both higher weight loss and decreased high‐energy food intake. Other hormones had no impact on food intake in this study. These changes also could explain the difference in better weight loss and potentially better metabolic control of diseases in RYGB compared to SG. We also know that biliopancreatic limb (BPL) lengths affect the outcome of weight loss and metabolic control through changes in gut hormone. Patrício et al. studied hormonal changes in 60–90 cm versus 200 cm BPL with a constant Roux limb length in RYGB. They did not measure glicentin or oxyntomodulin. However, they reported a significant increase in fasting basal GLP‐1 and another hormone, neurotensin, levels in longer BPL. These were also higher after a mixed meal test. Neurotensin is another hormone that is co‐secreted with GLP‐1 and PYY from L‐cells in response to fat, and bile salts in particular. It plays an important role in anorexia and decreasing food intake. Other hormones, PYY, glucagon, GIP and PP, were not significantly different. PYY was higher postprandially, while GIP was lower in longer BPL but without statistical significance. Of note, while glucose levels were similar in both groups, insulin levels were lower, suggesting a better, more efficient metabolic process generated by the longer BPL. All these studies suggest that we are only scratching the surface of the important changes and mechanisms that occur after bariatric surgery that potentially solve the mystery of obesity. Below we summarise each gut hormone, its action, source and the changes that occur in patients with obesity at baseline, with dieting and after various bariatric procedures (Table 14.1: gut hormones and effects: Representing table 1 in Pucci and Batterham (2000) with modifications). In 1990, Boozer et al. reported that ileal transposition in mice not only produced weight loss but also attenuated any weight gain by high fat diet in comparison to sham rats. Although the mechanisms are still unclear until now, we now know that bariatric surgery significantly alters the bile acid levels in the enterohepatic circulation.
14
Mechanism of Action of Bariatric Procedures
Background
The Beginning of Change
Gut Hormones
), (b) patients with obesity (
), (c) RYGB (
) and (d) gastric banding (
) patients.
Bile Acids
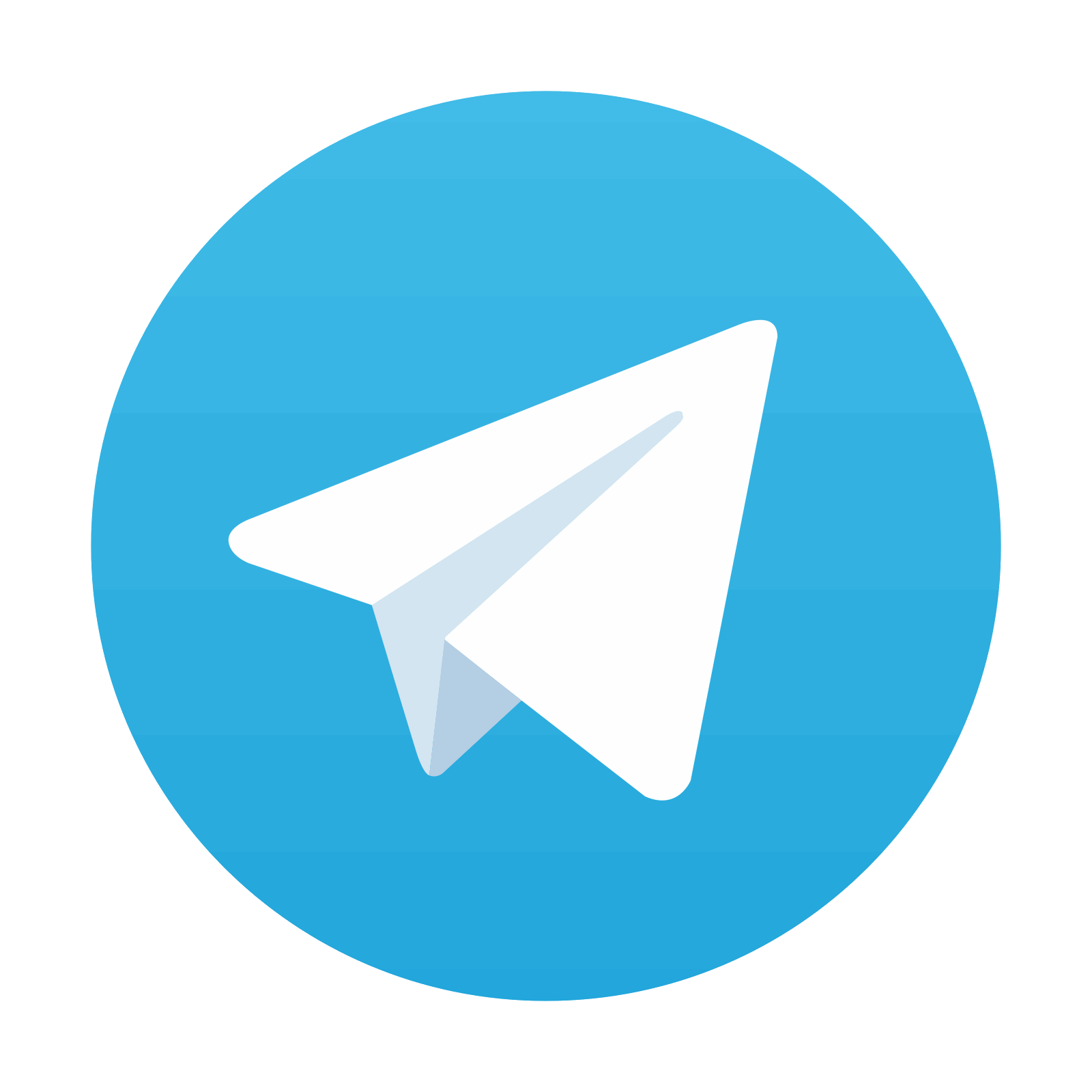
Stay updated, free articles. Join our Telegram channel

Full access? Get Clinical Tree
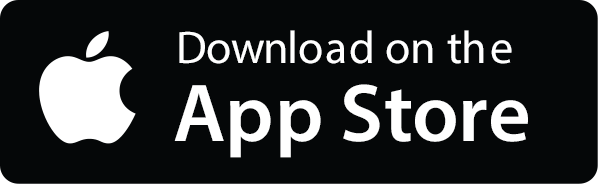
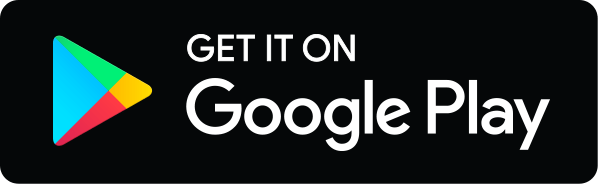