Fig. 6.1
Schematic representation of the testis and epididymis
The Testis
The primary function of the testis is to form spermatozoa and synthesize steroid hormones . These functions are accomplished by the seminiferous tubules, which are responsible for the production of spermatozoa, and the interstitial Leydig cells which synthesize steroids [10]. The seminiferous tubules are enveloped by a limiting membrane composed of peritubular myoid cells as well as monocytes [10]. The epithelium of the seminiferous tubules is composed of Sertoli cells and developing germ cells, which are formed by spermatogenesis and spermiation. At the end of spermiation, spermatozoa are released into the lumen of the tubules, coiled structures which fuse into the rete testis [13].
Testicular functions are regulated primarily by the hypophyseal hormones : luteinizing hormone (LH) , which regulates steroidogenesis by Leydig cells, and follicle-stimulating hormone (FSH) , which is implicated in Sertoli cell development. In addition, thyroid hormones (TH ) are critical for Sertoli cell differentiation. In the absence of TH, Sertoli cells maintain an immature appearance and continue to proliferate [14–19]. TH act on Sertoli cells to induce the expression of the gap junction protein Cx43, which induces cellular differentiation [20–23].
Spermatogenesis
The process by which spermatogonia develop and differentiate into spermatozoa is referred to as spermatogenesis. This process involves a complex interplay between the developing germ cells and between germ cells and Sertoli cells. The timing of spermatogenesis differs among species and can range from approximately 65 days in the rat to 85 days in humans [10]. This is a well-defined process characterized by a proliferative phase, in which spermatogonia divide by mitosis, a meiotic phase in which spermatocytes undergo two meiotic divisions, leading to the formation of haploid spermatids, and a morphological differentiation phase, or spermiogenesis, in which the cells develop a flagella, an acrosome, and a compacted nucleus, as a result of the replacement of histones by protamines, which bind the chromatin [10, 24].
Histological sections of the seminiferous tubule indicate that developing germ cells are arranged in well-defined associations. These associations can be grouped into different stages of development, which are referred to as the stages of the cycle of spermatogenesis. In the rat, 14 different stages of spermatogenesis have been distinguished and these can be used to assess perturbations in the process of spermatogenesis [25]. The stages of the cycle occur sequentially along the length of the seminiferous tubule. Since there are 4–5 generations of germ cells at each stage of development, each stage reflects the maturation of a particular generation of germ cells. For example, spermatozoa are released into lumen of the testis at stage VIII of spermatogenesis [25]. This requires specific proteins and a spatiotemporal expression so that these may exert their specific roles during spermatogenesis. Thus, the use of immunolocalization of these proteins with respect to the stages of spermatogenesis represents critical knowledge to assess the effects of toxicants on the testis of adults.
Spermatogenesis begins during postnatal development. In the rat testis, pachytene spermatocytes are first observed after the formation of the blood–testis barrier, which occurs between 14 and 15 days of age [26]. The first wave of spermatogenesis is completed at approximately day 42. This coincides with increasing blood testosterone levels, which peak at approximately day 39 [27].
As is the case in most tissues with cell proliferation, spermatogenesis is a delicate balance between cell proliferation and apoptosis. Gestational exposure to dibutyl phthalates was reported to cause a decrease in the number of germ cells in rat fetuses late in gestation by inhibiting cell proliferation [28, 29]. The effect, however, was transient, and germ cell number in exposed rats was similar to vehicle-exposed controls 2 days after birth [28, 29]. In contrast, exposure of rats to mono-(2-ethylhexyl)phthalate (MEHP) induced germ cell apoptosis by increasing the expression of FasL in Sertoli cells. This increase was shown to be mediated via the NF-kappaB pathway which, along with the Sp-1 transcription factor, bound to specific response elements on the FasL promoter to increase its expression [30]. Experiments examining the effects of toxicants that target the Sertoli cells, such as MEHP, 2,5 hexanedione (2,5HD), and carbendazim, were all shown to induce germ cell apoptosis in the testis, as determined by Tunel staining [31]. However, the mechanisms of toxicity and the parameters that resulted from exposure to any of these toxicants were varied, suggesting that standardized histopathological assessment of the testis may be difficult to achieve.
Assessing effects on the development of the testis may also be complicated and the timing of exposure may be important in identifying effects on specific windows of testicular development. In utero exposure to tributyltin (TBT) from gestational days 0–19 or 8–19 resulted in a decreased number of Sertoli cells at the highest dose (20 mg/kg bw/day) and for both exposure time periods, but gonocyte populations decreased only in rats treated from gestational days 8–19 [32]. In the intertubular region between adjacent interstitial cells, immunostaining for the gap junctional protein Cx43 was reduced or completely absent in treated rats. This suggests the need of more than one histopathological approach to understand the effects of toxicants on the developing testis [32].
The Blood–Testis Barrier
The differentiation of Sertoli cells culminates with the formation of the blood–testis barrier [33]. The barrier is composed of basally located tight junctions between neighboring Sertoli cells of the seminiferous tubules. Tight junctions are closely associated plasma membranes from adjacent cells that form an impermeable barrier to large molecules. The tight junctions between Sertoli cells comprise occludin and claudins (Cldns), which are transmembrane proteins. While initial studies identified several claudins in the testis, Gow et al. [34] showed, using transgenic mice, that the blood–testis barrier was dependent on the presence of Cldn11. In the absence of Cldn11, the barrier was compromised and spermatogenesis was arrested. In androgen receptor-null mice, Meng et al. [35] reported that the blood–testis barrier was also compromised and there was a decrease in Cldn3 levels. Whether or not the decreased levels of Cldn3 were the cause, or a consequence, of loss of barrier function in the testis remains to be established. In occludin-null mice, the first waves of spermatogenesis appeared to be normal, although over time the males became infertile. Clearly, alterations in the expression and localization of either occludin or specific Cldns appear to represent critical markers for Sertoli cell barrier function, since these proteins can be sensitive targets of toxicants [36].
The formation of testicular tight junctions and the blood–testis barrier are complex and involve both cell adhesion molecules, in particular neural cadherin (cdh2), and connexin43 (Cx43)-mediated gap junctions. Cadherins are single-pass transmembrane proteins that mediate calcium-dependant cell adhesion [12, 37, 38]. They are responsible for the formation of the zonula adherens around the cells of epithelia. Cyr et al. [39] first described the presence of Cdh2 and placental cadherin (Cdh3) in the rat testis. In the mouse, Cdh3 is expressed in gonocytes and weakly expressed in Sertoli cells during development [40]. In the adult, Cdh3 is localized to the peritubular region of the seminiferous tubule and Sertoli cells [41], and it has been reported in spermatogonia and spermatocytes [42]. In contrast to Cdh3, Cdh2 levels increase during the first wave of spermatogenesis and Cdh2 is localized to the surface of spermatogonia and primary spermatocytes, and possibly early spermatids [39, 43]. Johnson and Boekelheide [44] reported that Cadh2 was present between spermatocytes and Sertoli cells in the rat. In vitro studies showed that Cd disrupted tight junctions between Sertoli cells. This effect was accompanied by decreased levels of occludin and cellular relocalization of E-cadherin [45, 46].
Gap junctions mediate intercommunication between neighboring cells and are critical for cell proliferation and differentiation. They are composed of connexons, or hemichannels, contributed by each of the neighboring cells. Connexons comprise six connexins, and the contribution of Cxs confers selectivity of the pores between the cells [38, 47]. Gap junctions are responsible for intercellular communication by forming intercellular pores between cells that allow for the movement of small molecules (<1 kDa), including secondary messengers (e.g., cAMP), between cells. Gap junctions have been shown to be highly sensitive to chemical insult in many tissues, particularly the liver [48, 49]. In the testis, Cx43 colocalizes with occludin in Sertoli cells in the area of the blood–testis barrier [50]. Several studies have shown that Cx43 is important for the formation tight junctions between Sertoli cells [51–54]. Li et al. [53] have reported that Cx43, along with plakophilin2, can alter the transepithelial resistance of Sertoli cells in vitro, suggesting that these two proteins interact in the regulation of tight junctions. However, in mice lacking Sertoli cell-specific Cx43, a blood–testis barrier was formed and shown to be functional [51]. Furthermore, levels of occludin, N-cadherin, and β-catenin were all increased, while ZO-1 levels were decreased. Similar results were obtained in Sertoli cells in which Cx43 levels were knocked down using antisense siRNA. While these results suggest that having a functional blood–testis barrier is not the only requirement for spermatogenesis to proceed, the question arises as to whether or not the barrier is, in fact, fully patent and functional. Alterations in adherens junctions indicate that the dynamic nature of the blood–testis barrier is altered in Cx43 Sertoli-null mice. The tight junctions of the blood–testis barrier are unique in that developing germ cells must be transported across the tight junctions from the basal to the adluminal compartment. Thus, the dynamic nature of testicular tight junctions requires precise interactions between various types of cellular junctions to coordinate these processes. However, the question remains as to how Cx43 gap junctions accomplish this; perhaps the asymmetric intercellular communication between Sertoli cells and germ cells reported by Risley et al. [55] activates signaling from the germ cells back to the Sertoli cells to regulate both the adherens and tight junctions of the blood–testis barrier.
Studies by Aravindakshan et al. [56] showed that lactating female rats fed fish captured at a site where endocrine-disrupting chemicals induced estrogenic effects in fish resulted in alterations in spermatogenesis in the pups when they became adult. This effect was accompanied by decreased immunoreaction of Cx43 between Sertoli cells of the testis. To assess whether or not the effects on the testis were due to estrogenic chemicals, Aravindakshan and Cyr [57] exposed murine Sertoli cells (TM4 cells) to varying doses of nonylphenol, a prominent contaminant near the site where the fish were captured, and estradiol. Both intercellular gap junctional communication assays and Cx43 immunofluorescence studies indicated a dose-dependent response with increased concentrations of nonylphenol. However, estradiol exposure had no effect on either Cx43 levels or intercellular communication, indicating that the effects on gap junctions mediated by nonylphenol did not occur via an estrogenic signaling pathway (Fig. 6.2).
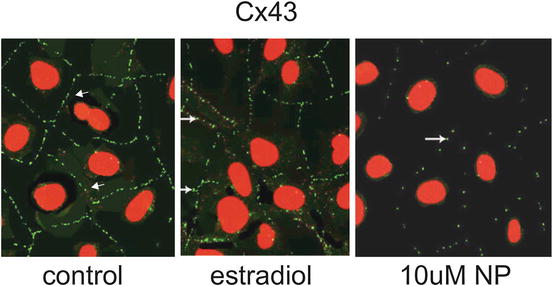
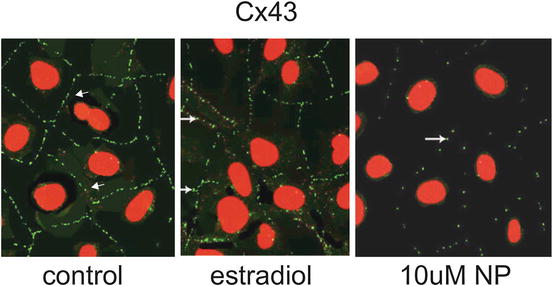
Fig. 6.2
Photomicrographs of TM4 murine Sertoli cells showing the immunolocalization of Cx43. In control cells, punctate Cx43 immunofluorescence (arrows) is prominent at the plasma membranes between adjacent cells. Cells treated for 24 hrs with 17β-estradiol did not show significant changes in Cx43 staining. In cells incubated with 10 μM of nonylphenol for 24 hrs, Cx43 immunostaining (green) is dramatically decreased (arrows). Nuclei are stained with propidium iodide (red) (×600)
The Epididymis
Spermatozoa from the testis are immotile and cannot fertilize. They must transit through the epididymis, where they acquire the ability to both swim and fertilize, a process referred to as sperm maturation . Testicular input to the epididymis is conveyed via 3–5 efferent ductules which anastomose to form a unique and highly convoluted epididymal duct. Early studies by Bedford [58] and Orgebin-Crist [59] indicated that epididymal sperm maturation was due to the exposure and interaction of the spermatozoa with components of the fluid of the epididymal lumen. Thus, the ability of the epididymis to provide the appropriate milieu for sperm maturation is critical; this is dependent on the polarized functions of the epithelial cells that secrete and remove proteins and other biomolecules into the lumen. The luminal environment is also dependent on the presence of the blood–epididymis barrier , which is formed by tight junctions between epithelial cells that line the lumen of the epididymis [12, 60].
Structure of the Epididymis
In most species, the epididymis comprises four segments identified according to functional and morphological differences: the initial segment, the caput, the corpus, and the cauda epididymidis. In the human, there is no morphologically distinct initial segment as the proximal caput region appears to comprise primarily of efferent ducts [61]. Whether or not some of the physiological functions of the initial segment exist in the efferent ducts of the human or immediately adjacent to these in the human epididymis is not known.
In all species, the basic structure of the epididymal epithelium remains very similar and is composed of a pseudostratified columnar epithelium containing principal, clear, and basal cells throughout the epididymis. Narrow and apical cells are present exclusively in the initial segment region. Principal cells are the most abundant cell type of the epididymis and are involved in protein secretion and absorption. They have an extensive stereocilia and an elaborate Golgi network. Clear cells are dispersed between principal cells in the epithelium and their numbers increase along the length of the epididymis from the proximal to distal regions [62]. These cells have poorly developed stereocilia and are primarily involved in absorption of materials from the lumen. Basal cells are located at the base of the epithelium and have long thin projects that interact with other basal cells and may extend apically to the lumen. Basal cells are thought to have a role in immunity and/or in the regulation of principal cell functions [63–65].
The Blood–Epididymis Barrier
The ultrastructure of tight and gap junctions in the epididymis was first described by Friend and Gilula [66] in the rat. They reported the presence of an extensive tight junction between adjacent principal cells localized to the apical region of the cells. Pelletier [67] reported the presence of tight junctions between principal and clear cells in the mink epididymis. Using thin section electron microscopy, Cyr et al. [68] demonstrated that the length of the junctional complex in the rat is reduced along the lateral plasma membrane of principal cells in the corpus and cauda, as compared with the initial segment.
Using immunofluorescence and confocal microscopy, Cyr et al. [50] showed that the tight junction protein occludin was expressed as early as embryonic day 13.5 in the murine epididymis. At this age, however, occludin was localized primarily in the cytoplasm. By embryonic day 18.5, however, occludin was localized to the lateral margins of plasma membrane of epithelial cells. Occludin is localized to the apical cell surface of the mouse epididymis by embryonic day 18.5, which coincides with peak androgen levels, suggesting that androgens may be important in the regulation of embryonic epididymal tight junctions. The immunolocalization of occludin correlated well with studies of Suzuki and Nagano [69] who had reported that the number of tight junctional strands in the apical region of the epididymis increased as a function of age. Cldns are also present between adjacent principal in the area of the tight junction and, in the case of Cldn1, along the lateral margins of cell [70, 71] (Fig. 6.3). The importance of cell–cell interactions in the developing epithelium is critical to toxicology with respect to assessing the effects of toxicants on these interactions. Tight junctions have been shown to be sensitive targets for environmental toxicants, which affect primarily the levels of proteins implicated in the formation of tight junctions.
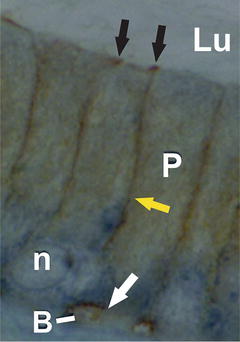
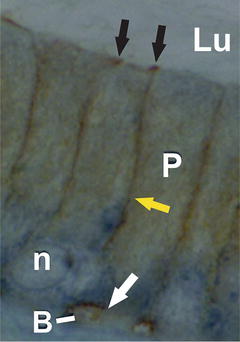
Fig. 6.3
Immunocytochemical localization of Cldn1 in the caput region of the epididymis. Cldn1 was localized to the apical region of the epithelium where tight junctions are located (black arrows). Immunostaining was also observed along the lateral margins of the plasma membrane (yellow arrow) and between basal and principal cells (white arrow). P principal cell, B basal cell, Lu lumen, n nucleus
In addition to tight junctions, gap junctions are present in the apical region of the epithelium and were observed by freeze fracture electron microscopy to be intermixed with the tight junctions of the blood–epididymis barrier [66]. Pelletier [67], using freeze fracture electron microscopy in the mink, a seasonal breeder, showed that gap junctions were present basal to the tight junctions but also at the basal region of principal cells. He also reported that gap junctions were present between principal and clear cells. Cyr et al. [72] using immunohistochemistry showed that connexin 43 (Cx43) was localized between principal and basal cells of the adult epididymis. Furthermore, their results showed that the cellular localization of Cx43 varied as a function of circulating levels of androgens. In castrated or orchidectomized rats, Cx43 was immunolocalized to the apical region of the epididymal epithelium between adjacent principal cells, as well as between basal and principal cells. In castrated animals administered testosterone, Cx43 remained localized between principal and basal cells and no apical staining was observed. Thus, its localization has potential for identifying the effects of anti-androgenic endocrine-disrupting chemicals on epididymal function since the cellular localization of Cx43 varies as a function of androgens in the epididymis.
Dufresne et al. [73] identified other epididymal Cxs, Cx26, 32, 31.1, and 30.3, in addition to Cx43. Unlike Cx43, both Cx26 and Cx32 were localized between adjacent principal cells, using immunofluorescent techniques in cryosections of the rat epididymis. These two Cxs were colocalized in young rats, but their localization along the lateral plasma membrane of epididymal epithelial cells was distinct in adult rats [73].
Markers of Epididymal Function
There have been relatively few toxicology studies that have focused on epididymal function from the perspective of assessing changes in immunolocalization of specific proteins. However, since the epididymis is critical to sperm maturation, and requires well-coordinated functions along the epididymal tubule in order to regulate sperm maturation, the use of immunohistochemistry markers to assess the function of specific cell types within the epithelium of the epididymis is crucial to understanding toxicant-induced alterations to cauda epididymal sperm.
The most abundant cell type of the epididymis is the principal cell. These cells line the lumen of the epididymis and are involved in the secretion and reabsorption of proteins from the lumen. Several proteins secreted by the principal cells can be used to examine the secretory capacity of principal cells and these have been previously reviewed. A detailed list of secreted proteins, cell types, and which epididymal region secretes these proteins has been produced [74].
Basal cells are located at the base of the epididymal epithelium. Using immunofluorescence and confocal microscopy with three-dimensional reconstruction, it was possible to demonstrate that these cells have extensive lateral projections that form a basket-like structure [63, 64, 75]. Immunohistochemical studies indicated that basal cells express superoxide dismutase-1 (SOD1), glutathione-s-transferase (GST), and metallothioneins (Fig. 6.4) [63, 76, 77]. Studies with GST immunohistochemistry suggested that basal cells may have projections that extend apically. A recent confocal microscopy study using paraformaldehyde-fixed thick sections and antisera against the tight junction protein Cldn1 indicated that basal cell projections may reach the lumen of the epididymis . The authors suggested that these may act as epididymal sensors. Given the fact that these cells express a number of proteins and enzymes implicated in the scavenging of free radicals, it appears likely that one of the functions of these cells is to protect the epithelium, and possibly maturing spermatozoa, from free radicals. While heavy metals, such as mercury, can induce metallothionein in the epididymis, few studies have examined the role of basal cells in the scavenging of free radicals following chemical insult. Clearly, however, these cells may play an integral role in detoxification in the epididymis.
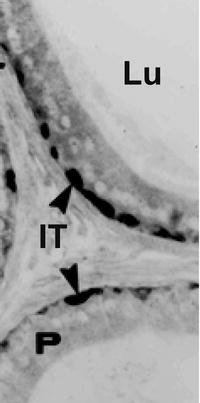
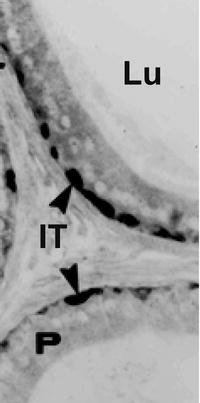
Fig. 6.4
Immunocytochemical localization of metallothionein in the rat epididymis. In the cauda epididymidis of adult rats, metallothionein was immunolocalized in basal cells (arrowheads). No reaction was observed over principal cells or spermatozoa in the epididymal lumen. P principal cells, Lu lumen, IT interstitium
Clear cells are endocytic cells present in the caput corpus and cauda epididymidis. Compared to principal cells, the cells have poorly developed stereocilia and contain a vast number of endosomes, multivesicular bodies, and lysosomes. The cells endocytose the cytoplasmic droplets that are released from maturing spermatozoa during epididymal transit in the corpus epididymidis [62]. Clear cells are also implicated in the regulation of luminal acidification via a number of proton-secreting ATPases (V-ATPase) which are activated via bicarbonate-sensitive adenylate cyclases [78].
Many of the physiological functions of the epididymis are highly dependent on the actions of androgens. As with the ventral prostate and seminal vesicles, the weight of the epididymis is highly correlated with circulating levels of androgens [79, 80]. The level of testosterone in the luminal fluid of the epididymis is approximately 10 times higher than circulating levels [81]. The androgen-metabolizing enzyme 5α-reductase, which converts testosterone to dihydrotestosterone, is present in epididymal principal cells, particularly in the initial segment, which contains high levels of 5α-reductase type 1while type 2 distributed all along the epididymis. Androgen receptors are present in both principal and basal cells throughout the epididymis [81]. Several genes have been identified that are either androgen stimulated or androgen repressed [79, 81]. Many of these have been identified immunohistochemically and represent markers that could be used to assess the effects of toxicants on androgen action. For example, clusterin (also known as sulfated glycoprotein-2) is an androgen-repressed gene [82]. It is expressed in the principal cells of the distal initial segment and caput and weakly expressed in the corpus and cauda epididymidis [83]. Orchidectomy and orchidectomy with androgen replacement experiments have indicated that both carbonic anhydrase (CA) II, which is expressed throughout the epididymis, and CAIV, which is expressed in the corpus, are androgen-dependent genes [84]. The cellular distribution of certain junctional proteins has been shown to vary in response to the absence of testosterone in orchidectomized rats. For example, Cx43, which is localized in normal intact animals between principal and basal cells, is localized not only between basal and principal cells in orchidectomized rats but also between adjacent principal cells. In rats whose testosterone levels have been maintained using subdermal implants, Cx43 is localized exclusively between basal and principal cells [72]. These observations highlight the importance of using an immunohistochemical approach to assess the effects of hormones or toxicants on epididymal proteins.
Epididymal Cell Lines
Several in vitro models have been used to study the epididymis. Most of the in vitro models initially relied on organ cultures, tubule cultures, or primary cell cultures. Telgmann et al. [85] developed one of the first immortalized epididymal cell lines from the dog by transfecting primary epididymal cultures with plasmids expressing either the SV40 Large-T antigen (SV40 LTAg) or c-myc. Murine epididymal cell lines were also derived from mice harboring a temperature-sensitive SV40 LTAg transgene [86]. Several other murine epididymal cell lines were derived from transgenic animals expressing an SV40 LTAg transgene under the control of the glutathione peroxidase-5 promoter [87]. Our laboratory has developed an immortalized rat epididymal principal cell line [88]. These cells (RCE-1) express transcripts for hormone receptors, epididymal-specific genes, and junctional proteins including several Cxs and Cldns. Immunofluorescence studies have revealed that these junctional proteins were localized to the points of cell–cell contact, indicative of functional intercellular junctions. More recently, we used a similar approach to develop human epididymal cell lines from both fertile and infertile patients [89, 90]. These cell lines form functional tight junctions and using siRNA, we demonstrated that downregulation of a single Cldn could alter the formation of tight junctions, as determined by measuring transepithelial resistance (TER) across the cells in culture. This indicated that a loss, or mistargeting of a single Cldn, as observed in nonobstructive infertile patients [89], is sufficient to compromise the blood–epididymis barrier. To further understand the role of Cldns and the blood–epididymis barrier in male infertility, we examined epididymides in infertile patients with obstructive azoospermia (OA). We observed that mRNA levels of epididymal Cldns 1, 4, and 10 were altered downstream from the obstruction site in OA patients. Epithelial cell lines were derived from the caput epididymidis of OA patients (infertile human caput epididymal cell line (IHCE) ) [90]. IHCEs cells did not express many Cldns and were unable to form tight junctions. The use of cell lines has obvious limitations for immunolocalization studies since they comprise a single cell type, whereas the epididymis is a pseudostratified tissue with multiple cell types. Nevertheless, these cell lines have been particularly useful in elucidating cellular and molecular mechanisms. A recent study on the characterization of the ATP Binding Cassette B1 (ABCb1), also known as the multidrug resistance 1 transporter, used immunohistochemical techniques to characterize the cellular localization of ABCb1 in the rat epididymis [91]. This was complemented with studies using rat epididymal RCE cells to show that ABCb1 could be induced by alkylphenols, in this case nonylphenol and octylphenol, which could help explain the limited accumulation of alkylphenols in the epididymis of rats treated with octylphenol [92]. Thus, these cell lines from various animal models provide critical tools that can be used for toxicology studies.
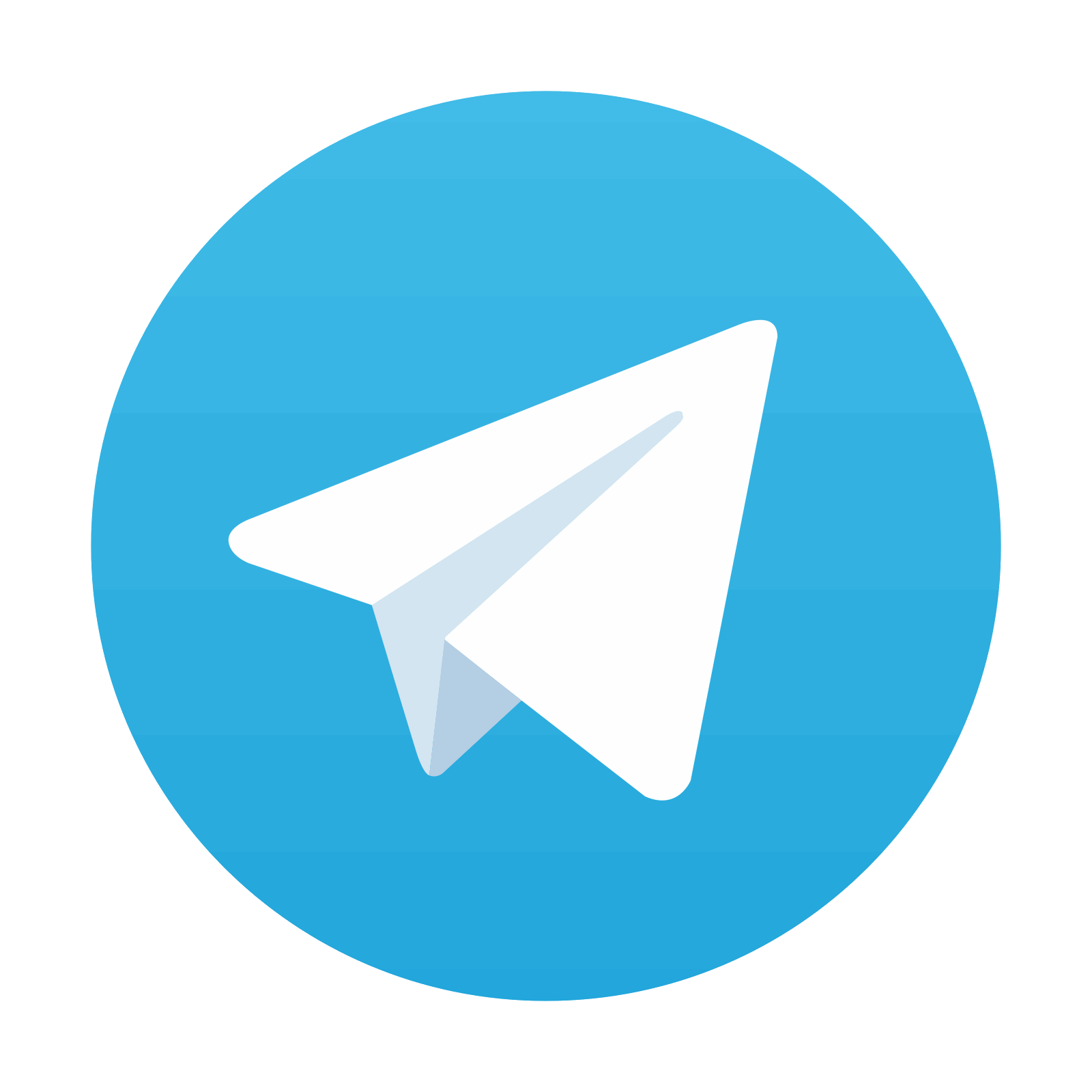
Stay updated, free articles. Join our Telegram channel

Full access? Get Clinical Tree
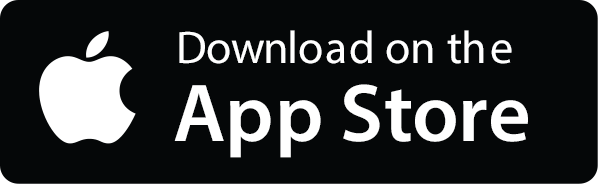
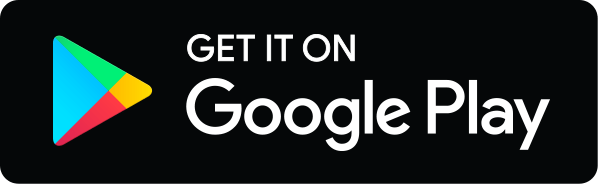