3 Local anaesthetics and analgesics
Introduction
Pain is a sensation that lets us know about damage occurring to our tissue. It is one of our defence mechanisms and is important to our survival. Therefore, when we consider modifying the experience of pain, it is important to remember that, although distressing and unpleasant, pain has a role to play in protecting us. Having said all this, it is possible to suffer severe pain without any obvious tissue damage or injury – for example, trigeminal neuralgia (severe burning or stabbing pain in the face arising from one of the nerves carrying sensation). On the other hand, pain may become a dominant feature in a person’s life long after tissues have healed – for example, phantom limb pains. It is also possible that tissue damage in one part of the body can lead to pain being perceived in another part of the body. This is called referred pain.
Pain is therefore a very difficult concept to define. As it becomes more chronic in nature (i.e. more than six months in duration) it begins to affect all activities of daily living, not just from a physical but also from a psychological and sociological perspective. The nurse has a unique role to play in minimizing pain for patients. In order to carry out this role effectively, nurses need to have some understanding of the drugs used in pain relief.
However, before we plunge headlong into the classification of local anaesthetics and analgesics, we need to remind ourselves of the basic principles of, first, the initiation and conduction of nerve impulses, and second, the body’s pain pathways and analgesic system.
Neurones
Neurones are specialized cells whose function is to transmit messages. A neurone has branch-like processes called dendrites that convey incoming messages (action potentials) towards the cell body (see Figure 3.1). The cell body houses the nucleus and therefore is the metabolic centre. Conducting impulses away from the cell body we have structures known as axons, which terminate in axon terminals. Housed in the axon terminals are hundreds of small sacs (vesicles), which contain neurotransmitters. The nerve impulse therefore travels from the dendrites along the axon and finishes at the axon terminal where the impulse stimulates the release of the neurotransmitter.
Quite a number of neurones are covered with a substance called myelin, formed by supporting cells called Schwann cells, which wrap themselves around the axon. The myelin covering acts as an insulator for the nerve and also affects the speed at which messages can travel along individual neurones.
Neurones carrying messages from the internal organs and skin into the central nervous system are called sensory (afferent) neurones. Conversely, neurones carrying messages out of the central nervous system to the muscles, glands and internal organs are called motor (efferent) neurones.
The nerve impulse
At rest, the inside of a neurone has a negative charge compared to the outside, which is positive. This is because the inside of a neurone carries a lot of negatively charged particles called anions. Anions are large particles that find difficulty in moving through the wall of the neurone. The outside of the neurone on the other hand contains lots of positively charged particles called cations. The major cation in our discussion here is sodium.
In order to trigger the sensation of pain, the inside of the neurone must become positively charged as compared to the outside. Chemicals liberated by damaged tissue (e.g. bradykinin, histamine, serotonin, potassium and other proteolytic enzymes) bring about a change in the neuronal membrane, allowing sodium to pass into the neurone. As the sodium enters, the membrane becomes even more permeable to sodium. This continues until the inside of the neurone is positively charged in relation to the outside. This build up of positively charged sodium is termed depolarization.
At the synapse, where one neurone meets another, neurotransmitters must be released in order to stimulate the next neurone to continue to pass the message onwards. This process is important when considering analgesics and how they work. Once the neurone has converted to a positive environment, calcium channels in the membrane open, so allowing calcium to enter. The influx of calcium attracts small sacs called synaptic vesicles, which are full of neurotransmitters, towards the synapse. When these vesicles reach the synapse they fuse with the neurone membrane and pour their cargo of neurotransmitter into the synapse itself (see Figure 3.2).
The neurotransmitters which are important in the pain pathway are substance P, neurokinin A and B, and glutamate. Once the neurotransmitter attaches itself to receptors on the other side of the synapse, this neurone then becomes permeable to sodium and the process continues (see Figure 3.3).
Having discussed the initiation and transmission of a nerve impulse, we need now to consider the body’s pain pathways.
Pain reception
Pain is received via specialist receptors called nociceptors, which are free nerve endings that lie in the tissues. They are most plentiful in the skin; as you move deeper into the tissues of the body, they become fewer in number. Nociceptors are stimulated by mechanical, thermal and chemical means and have a high threshold. This means that only stimuli indicating some degree of tissue damage are perceived as pain. For example, if you were to apply a heat pad to your skin you would not stimulate your nociceptors until it reached a heat of 40°C, at which point you would feel a sensation of pain. Nociceptors are non-adapting, which means they are stimulated at the same level of intensity each time the threshold level is reached. In other words, you do not raise the threshold at which the nociceptors are stimulated just because the pain is constant. This is an important aspect to our survival.
In addition to noxious stimuli like heat and pressure, nociceptors can become sensitive to a variety of chemicals which are present after local tissue injury. These substances include potassium, serotonin, bradykinin, histamine and prostaglandins. Prostaglandins are normally produced by the body to ensure the smooth running of our internal environment (homeostasis). They are of particular importance when considering certain medicines, such as NSAIDs.
Painful stimuli are received by the nociceptors and then transmitted by two different types of nerve fibres: A delta fibres and C fibres. A delta fibres are myelinated fibres, small in diameter, which are stimulated by mechanical means. A myelinated nerve is one covered by a fatty insulating substance called myelin. As the fibres are myelinated they convey impulses very quickly. Stimulation of these fibres leads to sharp prickling pain that the person can easily localize. C fibres are also small diameter fibres but are unmyelinated and so convey impulses more slowly, resulting in a ‘burning’ or ‘aching’ pain which is harder to pinpoint. It will tend to be more general in nature, and a good example is abdominal pain.
Both A delta and C fibres synapse with the next neurone in the pathway in the dorsal horn of the spinal cord. It is in this area of the dorsal horn (i.e. the substantia gelatinosa) that Melzack and Wall ((1965)) proposed a gate-controlled entry for the sensation of pain into the central nervous system. If the gate was opened a person would perceive pain; if the gate was closed a person would not.
The sensation of pain is then carried by what are referred to as the spinothalamic tracks, which carry impulses from the spinal cord up to the thalamus. One track carries information from the A delta fibres and the other from the C fibres. These tracks once again synapse in the thalamus and the impulses are conveyed to a number of areas including the sensory cortex, where they are interpreted as pain (see Figures 3.4 and 3.5).
The body’s analgesic system
As with other sensory systems in the body, the pain pathway has a descending mechanism (see Figure 3.6). This mechanism constitutes the body’s attempt to modify the pain we interpret. In other words, it is our inbuilt analgesic system.
This analgesic system commences at an area of the brain called the periaqueductal grey (PAG). This is a tiny part of grey matter that lies in the mid-brain. It receives input from a number of brain areas, among them the hypothalamus, cortex and thalamus. The PAG is thought to be the mechanism by which the gate to pain can be closed. It would certainly explain in physiological terms why psychological approaches to pain management are successful.
The PAG connects to an area in the medulla called the nucleus raphe magnus (NRM). This in turn sends fibres down the spinal cord and they interact at the dorsal horn. Therefore, higher brain centres have the infrastructure to open or close the gate to pain in the substantia gelatinosa.
While we suggested that substance P was a neurotransmitter which has been implicated in the transmission of pain, there are also a number of peptides that have been discovered which have been implicated in the brain’s analgesic system. The three families of these peptides are endorphins, dynorphins and enkephalins. The discovery of these proteins has been very valuable in our understanding of why opiate drugs, such as morphine, have such a unique effect on the body.
Types of pain
Nociceptive (tissue) pain
Nociceptive pain results from tissue damage. Intact pain pathways report this damage via their neurons, and pain is experienced. Nociceptive pain can further be subdivided into somatic and visceral (gut) pain. It can be experienced as sharp, dull, or aching. There may be radiation of the pain, especially visceral pain, but it will not be in a direct nerve distribution. For example, gall bladder pain can radiate to the tip of the left scapula. Nociceptive pain generally responds to NSAIDs and opioids, both of which will be introduced further in this chapter. Conditions associated with inflammation, bone pain, and joint disease are particularly responsive to NSAIDs.
Neuropathic (nerve) pain
Neuropathic pain may occur when there is either damage to or dysfunction of nerves in the peripheral or central nervous system. This results in faulty signals being sent to the brain and experienced as pain. Neuropathic pain can be either peripheral (outside the central nervous system) or central (spinal cord and brain) in origin. Examples of neuropathic pain include diabetic neuropathy, trigeminal neuralgia, postherpetic zoster pain (peripheral pains) and thalamic pain syndrome (a central pain). Neuropathic pain frequently exists alongside nociceptive pain. Examples include trauma that damages tissue and nerves, burns (that burn skin as well as nerve endings) and external nerve compression. Examples of the latter include tumours that press on (compress) nerves and the pain of sciatica, caused by herniated intervertebral discs pressing on nerves.
Patients often describe neuropathic pain as a ‘burning sensation’. They may also describe it as feeling like an electric shock or lightning bolt. Sometimes stimuli that usually do not cause pain, such as light touch, may elicit a painful response. A light stroke of the cheek that results in the sudden pain of trigeminal neuralgia is an example of this type of pain. Sometimes patients do not describe the sensation as being ‘painful’ but rather as feeling unpleasantly strange or ‘tingly’, rather like an arm feels when it wakes up from ‘going to sleep’. This is called a dysesthesia. Diabetic neuropathy commonly results in this type of sensation.
Neuropathic pain in the peripheral nervous system frequently follows a nerve distribution. This distribution may follow a particular nerve, as in sciatic pain or trigeminal neuralgia, or may represent the distribution of terminal nerve endings, as in the ‘stocking-glove’ distribution of peripheral neuropathies. Neuropathic pain is relatively resistant to NSAIDs and opioids, although they may be helpful in certain cases. The other major classes of medications useful for neuropathic pain, tricyclic antidepressants, anticonvulsants and sodium channel blockers are referred to as adjuvant therapy which will be discussed later.
Local anaesthetics
Local anaesthetics are analgesic drugs designed to be used clinically to produce a reversible loss of sensation in a particular part of the body. Generally, there are two classes of local anaesthetic: ester agents, such as cocaine, procaine and amethocaine, and amides such as lignocaine, pilocaine and bupivacaine. The difference between the two comes down to biochemistry.
- Esters are more unstable in solution than amides and are relatively quickly broken down by enzymes in the plasma. One of the products produced when this group of drugs is broken down is a compound known as para-amino benzoate acid (PABA). Unfortunately, this has been associated with allergic responses and hypersensitivity reactions.
- The amide group do not have any of the above problems. Therefore, you may find in practice that you come across more drugs from this class.
Mode of action
Local anaesthetics block the sodium channels in the neuronal membrane. We can quite clearly link this back to our discussions on initiating an action potential. If sodium cannot move over the semi-permeable membrane then the inside of the neurone does not become depolarized – i.e. the environment remains negative rather than becoming positively charged. Local anaesthetics tend to block conduction more effectively in small diameter nerve fibres and this makes them perfect for the A delta and C fibres described earlier. Therefore, pain is blocked more effectively than other sensations, such as touch. Also, this makes it possible for the doctor to provide a good level of analgesia without too much loss of motor function. You can observe this for yourself in your patients or may even have experienced it following an epidural anaesthetic during labour.
Preparations of local anaesthetics
Local anaesthetics are usually dissolved in a solution which also contains a preservative and fungicide. The dilute preparations are usually presented as percentage solutions of the drug. For example, lignocaine 1 per cent contains 1g of lignocaine in every 100ml. The number of milligrams/millilitres can easily be calculated by multiplying the percentage of the strength by 10. Therefore, a 1 per cent solution of lignocaine contains 10mg of lignocaine to every millilitre of solution.
One of the problems encountered when giving a local anaesthetic is that many of them cause the surrounding vessels to dilate. This leads to rapid absorption of the drug, reducing its effect. For this reason, a vasoconstrictor is sometimes added to increase potency and prolong action. Vasoconstrictors also decrease the chance of systemic side-effects, making local anaesthetics safer. The most common drug added to local anaesthetics is adrenaline.
The effect of vasoconstrictors on the potency and duration of action of local anaesthetics varies according to the drug being given and the site at which it is being used.
Topical anaesthesia
Local anaesthetics are produced that are effective for the skin, eyes, ears, nose and mouth (e.g. pilocaine, lignocaine and amethocaine). When used topically, the onset of action is around 5 to 10 minutes, the drug action lasting between 30 and 60 minutes. This method of application numbs a small area of skin so is ideal for relieving pain from sunburn, minor burns and insect bites. Topical application can also be administered to the surface of the eye allowing certain operative procedures to take place. It must be remembered that these drugs can be absorbed in a significant amount, especially if applied to very vascular areas.
This technique is used to provide anaesthesia for minor surgical procedures such as dental work. Lignocaine and pilocaine are again frequently used and the result is that the patient will feel pressure but no pain. The onset of action is very rapid, while the duration depends on the drug being used, pilocaine having the shortest period of action (15 to 30 minutes), while lignocaine provides a moderate length of anaesthesia (60 minutes). Bupivacaine has the longest duration of action, at around 200 minutes. Often the effects of the local anaesthetic continue after the procedure is concluded, so providing extra pain relief. As discussed earlier, the addition of adrenaline will increase the quality and duration of these anaesthetics.
Nerve blockade (conduction anaesthesia)
As the name implies, in conduction anaesthesia, the nerves relating to the area being operated upon are blocked with a local anaesthetic. The blockade may be minor (e.g. intercostals) or deep (e.g. brachial plexus). Drugs such as lignocaine are used for minor blocks and have an onset of 5–10 minutes and duration of 1–2 hours. In a major blockade, the onset can be more variable so communication with the patient is paramount. The local anaesthetic in this case takes a little longer to act (10–15 minutes), but has a more pronounced period of analgesia, at about 3–4 hours. Bupivacaine can be effective for up to 10 hours. The advantages of this approach are that a relatively small amount of the drug can be administered to achieve a wide area of anaesthesia. Nerve blockade can also be used in conjunction with a general anaesthetic to reduce the amount of drugs used in the procedure and the resulting side-effects.
Extradural, epidural and caudal anaesthesia
For these procedures, the local anaesthetic is injected between the dura (the outside covering of the brain and spinal cord) and the periosteum of the bones lining the vertebral cord. Either lignocaine or bupivicaine are used in this technique. The advantage of an epidural approach is that a carefully placed needle can achieve a pain-free band from about the mid-chest to the mid-thighs but at the same time allowing the legs to maintain their normal strength. A further local anaesthetic can be administered into a reservoir connected to a fine tube placed in the patient’s lower back.
Spinal anaesthesia
This is a specialized procedure which anaesthetizes the lower half of the body and does not maintain leg strength: the patient is unable to move their legs. The local anaesthetic in this case is put directly into the cerebrospinal fluid. The drugs are rapidly taken up, which leads to a faster onset and requires a smaller dose. Care must be taken if using this route of administration in pregnant women, as the volume of the subarachnoid space (see Figure 3.7) is diminished; therefore, reduced doses are required.
< div class='tao-gold-member'>
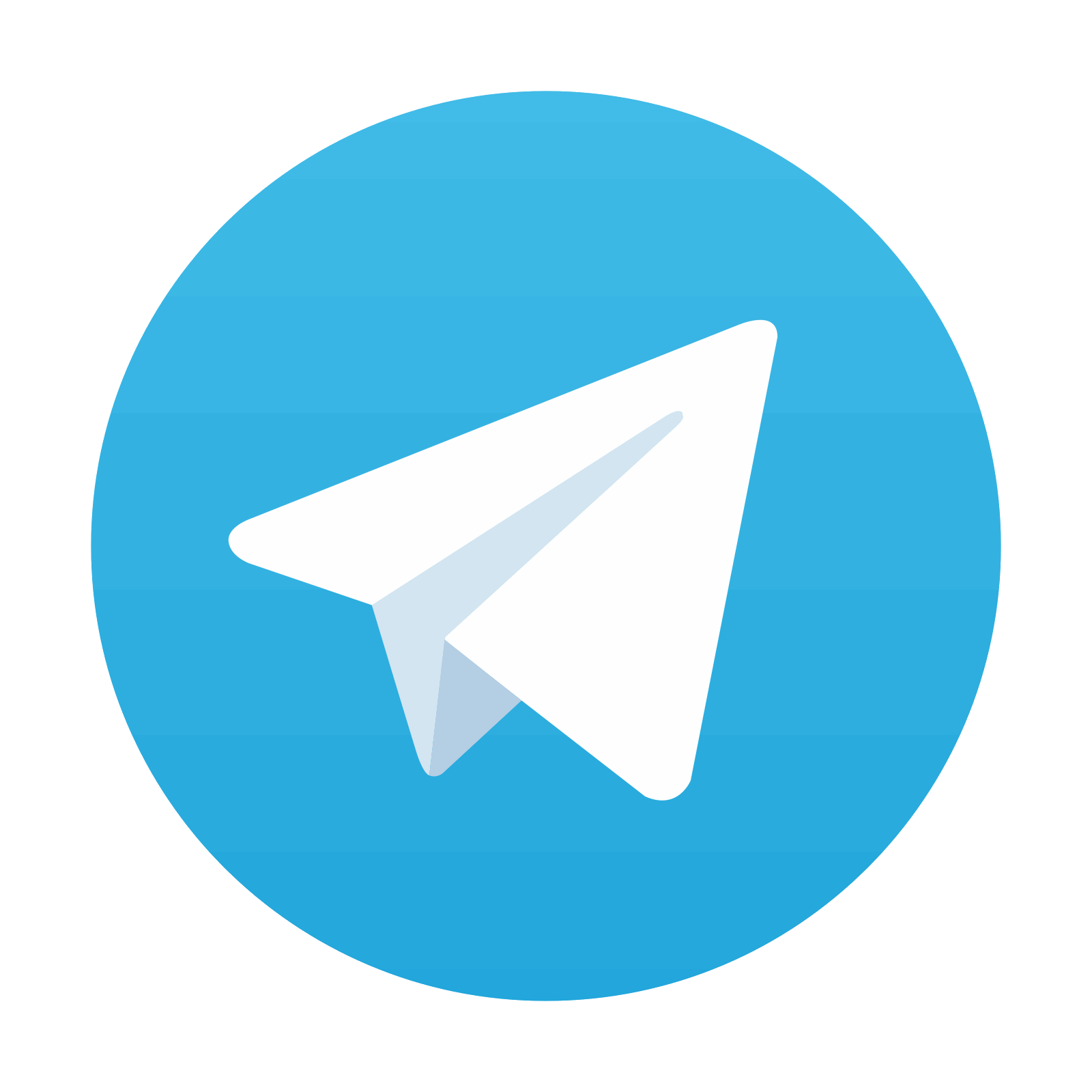