Liver and Renal Disease
LEARNING OBJECTIVES
• INTRODUCTION
Pharmacists in a variety of patient care practice environments are routinely required to evaluate patient’s liver function tests (LFTs) and renal function tests (RFTs) to differentiate between adverse medication effects and other causes of hepatic and renal dysfunction. The purpose of this chapter is to teach pharmacists how to evaluate patients for damage to the liver and the kidney, including those caused by complications of chronic disease, adverse drug effects, and other diseases of the liver and kidney. Pharmacists also interpret LFTs and RFTs to monitor patients taking medications with potential hepatotoxic or nephrotoxic adverse effects. Pharmacists also interpret certain RFTs to assist in the dosing of medications that are primarily excreted by the kidney.
• LIVER FUNCTION TESTS
The term liver function tests is a misnomer, because unlike renal function tests that measure both damage to and the effectiveness of the kidney, LFTs only measure damage to various structures in the liver and gallbladder. While RFTs can be used to determine the dose of medications primarily cleared from the body via the kidney, LFTs, since they measure only damage and not function, cannot be used to determine doses of medications metabolized in the liver (Table 24.1).
TABLE 24.1 | Laboratory Tests Used to Evaluate Liver Function |
Aminotransferases
Aspartate aminotransferase (AST) and alanine aminotransferase (ALT) are synthesized primarily in hepatocytes. Upon the destruction of hepatocytes these enzymes are released into the blood stream, causing serum levels to rise. The presence of elevated levels of AST and ALT in the serum usually indicate of hepatocellular damage. However, there are several issues that need to be considered when interpreting elevated levels of AST and ALT. The first issue concerns normal values. Most laboratory tests have a normal bell-shaped distribution in a given population; therefore, assessment of normal is more reliable. AST and ALT levels have a skewed distribution characterized by a long tail at the high end of the scale. This means that 2.5% of patients’ purported “abnormal” levels are actually normal for them. This skewing is more pronounced in nonwhite males. Therefore, slight elevations of aminotransferases (less than 1.5 times the upper limit of normal) may not indicate liver disease. The second issue involves the lack of relative specificity of these enzymes to the liver. AST is widely distributed throughout skeletal and cardiac muscle as well as the liver. Prior to the advent of serum troponin levels, elevated AST levels were used to diagnose acute myocardial infarction. In addition, vigorous exercise or muscle trauma can cause marked elevations in serum AST levels. While ALT is far more specific to hepatocytes, it is found in other tissues and may occasionally be the source of spurious elevations of ALT.
Alkaline Phosphatase
Alkaline phosphatase (AP or alk phos) is a group of iso-enzymes that cleave inorganic phosphate from organophosphates primarily in the bone and microvilli of the canaliculi lining the intrahepatic bile ducts. In LFTs, elevated levels of AP indicate obstruction of the biliary system. In extrahepatic obstruction such as gallstones, the AP may rise more slowly than other LFTs. Isolated elevations of AP can also occur normally in pregnancy (placental source), growing children, and in bone disorders, such as Paget’s disease.
Serum Proteins
The liver is responsible for protein synthesis. Therefore, in chronic liver disease such as cirrhosis, synthesis of protein is eventually impaired and serum levels fall. Because albumin, one of the major indicators of chronic hepatocellular damage, has a half-life of 20 days in the body, it is unaffected by brief periods of acute or mild hepatocellular damage. Serum globulin measures α- and β-globulins, which are synthesized in the liver, plus γ-globulins, which are synthesized by plasma cells and lymphocytes. Prothrombin, a clotting agent with a half-life of 60 hours, is more sensitive to liver damage. Prothrombin levels are approximated with the prothrombin time, which measures the time for a blood sample to clot when tissue clotting factors are added to the sample and combine with prothrombin to make the blood clot. Because of variations in the strength of different manufacturers’ tissue factors, an international normalized ratio (INR) is used to express prothrombin activity (and levels) instead of the prothrombin time. The normal INR is 1. As prothrombin activity decreases the INR value rises. Because a decrease of 80% in prothrombin levels is required for the INR to start to rise, it makes an excellent indicator of chronic hepatocellular damage along with a decrease in serum albumin. Another manipulation of serum protein levels, the albumin to globulin ratio (A/G ratio), can be an even more sensitive indicator of chronic hepatocellular damage. Normally the A/G ratio is greater than 1. As chronic liver damage increases over time, the production of albumin falls along with that of α- and β-globulins. Simultaneously, the liver damage decreases the liver’s ability to process antigens, which causes an increase in the synthesis of γ-globulins outside the damaged liver, which maintains a normal or elevated serum globulin level. If not given on the lab test report, the globulin can be calculated by subtracting the albumin serum level from the total protein serum level. An A/G ratio of less than 1 indicates significant, permanent destruction of a large number of hepatocytes as found in cirrhosis.
Other Common Biomarkers of Liver Damage
The γ-glutamyl transpeptidase (GGTP) and serum bilirubin levels can be used as adjuncts in the diagnosis of types of liver diseases. While specific to the liver, the GGTP is not routinely included in LFT panels because it is too sensitive and is frequently elevated in the absence of liver disease. Medications that cause CYP450 enzyme induction markedly raise GGTP levels. However, GGTP should be done in a case of isolated elevation of AP to confirm its liver origin, since both AP and GGTP are elevated in obstructive liver disease.
Since the advent of AST, ALT, and AP enzyme tests, the utility of serum bilirubin levels has markedly decreased, but can still be used to confirm results from other tests. Bilirubin is a by-product of hemoglobin catabolism and is transported to the liver, where it is quickly conjugated via hepatic glucuronidation and sulfation and is immediately excreted via the bile duct into the intestine. Roughly 10% of serum bilirubin is conjugated (also known as direct bilirubin). The remaining 90% is unconjugated (also known as indirect bilirubin). Ratios of direct and indirect bilirubin were used historically to attempt to differentiate between hepatocellular and obstructive liver disease, but were too unreliable. When total bilirubin levels near 3 mg/dL, check the conjunctiva, frenulum of the tongue, and nail beds for the yellow discoloration of jaundice. In addition, hemolysis can cause marked elevation of total bilirubin and is accompanied by a normochromic, normocytic anemia and a reticulocytosis on the complete blood count.
• INTERPRETING LIVER FUNCTION TESTS
The etiology and type of liver disorder or disease cannot be diagnosed by laboratory test alone. The combination of LFTs with the clinical picture, via a careful history and physical examination are essential to accurate diagnosis. There are four major types of liver damage: acute hepatocellular, severe chronic hepatocellular (cirrhosis), obstructive (cholestatic), and mild chronic hepatocellular (hepatitis C). All four types can be caused by medication and other pathological conditions of the liver. The pharmacist is frequently asked to try to differentiate between drug-induced liver disease and that caused by traditional etiologies. To be effective in this role, the pharmacist needs to know the type of liver injury each drug causes, matching the LFTs and the clinical picture to specific drugs. For example, phenothiazines cause cholestatic liver disease. In a patient on a phenothiazine, a cholestatic pattern would be seen, i.e., AP levels at least three to five times the upper limit of normal with mild elevations of AST and ALT. If the patient’s values showed AST and ALT levels >1000 units/dL and a normal AP, then the phenothiazine could be ruled out as a cause of the patient’s liver injury. Similarly, the pharmacist must be familiar with the LFTs and clinical presentation patterns of other common causes of liver injury and disease. Unfortunately, many times the exact etiology may elude accurate identification because of the limitations inherent in the available laboratory tests, or patient history and physical findings. In those cases, the suspected offending medication can be discontinued and the patient monitored for resolution of subjective and objective parameters, which can sometimes help confirm a drug-induced cause.
Acute Hepatocellular Damage
Regardless of the cause, acute hepatocellular damage presents with markedly elevated (>300 units/dL) AST and ALT levels as the primary laboratory test abnormality. Mild elevations of AP and unconjugated (indirect) bilirubin can also occur. Isoniazid, acetaminophen, allopurinol, valproic acid, and kava kava are examples of drugs/herbs that cause classical acute hepatocellular damage. These drugs are also said to cause drug-induced hepatitis since the symptoms, physical findings, and LFT patterns mimic viral hepatitis. Typically, other LFTs are normal or only mildly elevated. These medications have all been reported to cause liver failure and death if the drug is not discontinued. Patients may also present with symptoms associated with acute viral hepatitis, including fatigue, malaise, mild fever, anorexia, and nausea. Other than jaundice, physical examination is usually normal. Occasionally, patients will experience mild hepatic tenderness on palpation or percussion. To differentiate from other etiologies, patients should be questioned about herbal and nonprescription medication use, recent travel, transfusions, unprotected sexual intercourse, or tattoos. Given the routine immunization for hepatitis, blood tests for active hepatitis A, B, and C should be done, i.e., anti-hepatitis A IgM for hepatitis A, both anti-hepatitis B surface antigen and anti-hepatitis B core antigen IgM for hepatitis B, and HCV-RNA for hepatitis C. Laboratories may list these as a Hepatitis Panel on their order forms.
Cholestatic or Obstructive Damage
Regardless of the cause, cholestatic liver disease presents with markedly elevated serum AP levels, at least three and usually more than five times the upper limit of normal, plus a mild elevation of AST/ALT (<300 units/dL). Anabolic steroids, estrogens, phenothiazines, cyclosporine, and erythromycin are examples of drugs that cause a classical cholestatic pattern of liver injury (also known as cholestatic jaundice or drug-induced cholestasis) (Table 24.2). Drugs that cause cholestasis affect canaliculi of the intrahepatic bile ducts. Patients may present with symptoms that mimic gallbladder disease which includes cramping right upper quadrant (RUQ) abdominal pain or may be relatively asymptomatic. Physical examination may reveal a positive Murphy sign (pain during inspiration while palpating just under the right rib cage) or RUQ tenderness on palpation. Serum conjugated bilirubin (direct) is usually increased (>20%) if the swelling in the canaliculi obstructs the bile ducts, because the hepatocytes are mostly undamaged and continue to efficiently conjugate bilirubin. To differentiate drug-induced cholestasis from other etiologies, patients should be questioned about a personal or family history of gallbladder disease since Native Americans and Hispanic populations have a high incidence of gallbladder disease, relative to other ethnic populations. In addition, the patient should be questioned about recent fractures, medications that may cause osteomalacia, or recent growth spurts in adolescents, all of which cause isolated elevations of AP values. Abdominal examination should include inspection for scars typical of cholecystectomy. Abdominal ultrasound can reveal gallstones, obstructive masses of the gallbladder, or head of the pancreas if present. A GGTP level should be ordered to confirm the liver as the source of AP since it too is concentrated in the bile canaliculi. A normal GGTP indicates a further workup for bone disorders such as osteomalacia and Paget’s disease.
TABLE 24.2 | Lab Findings in Common Liver Diseases |
Some references refer to “mixed” drug-induced liver disease, where all three enzymes are elevated (AST/ALT/AP). Of interest is that viral hepatitis, particularly hepatitis A, can present with what appears to be a cholestatic pattern early in the course of the disease before changing to a pattern typical of acute hepatocellular injury. Whether or not medications, which cause liver injury, also present with that pattern is unknown. However, a careful review of cases labeled as mixed reveal a variety of criteria about lab test values. For example, some cases used any AP elevation to label it mixed, while some used 1.5 to 2 times the upper limit of normal. Some cases looked for other organs as the source of the excess AP and some ignored the possibility of an alternate source. Similar variations and issues also occurred in criteria for AST and ALT. Application of the criteria used in this chapter for acute hepatocellular and cholestasis to the purported mixed cases greatly clarifies the true nature of the liver injury, sorting all but a few into the four categories of hepatic injury.
Chronic Hepatocellular Damage (Cirrhosis)
The hallmark of cirrhosis or severe chronic liver disease is the decrease in capacity to synthesize proteins by the liver. Albumin and prothrombin are two primary proteins whose synthesis becomes impaired as fatty metamorphosis and fibrosis of the liver permanently decreases hepatic function. Albumin is measured via the serum albumin and the A/G ratio, while prothrombin levels are measured via the INR. In cirrhosis, serum albumin levels fall and the A/G ratio falls below 1. Simultaneously, the INR rises above 1.0. The changes in laboratory values show that the patient’s hepatocytes and hepatic sinusoids are being replaced by fibrous material, which eventually leads to the classic signs and symptoms of cirrhosis. The fibrosis eventually leads to decreased blood flow from the portal vein through the fibrous liver into the hepatic vein, leading to portal hypertension. Low albumin levels decrease plasma oncotic pressure. Portal hypertension and low albumin serum levels trigger a series of steps including activation of the renin-angiotensin system that causes hyperaldosteronism and increased intravascular volume. The increased intravascular volume, portal hypertension, and low albumin combine to push fluid into the abdominal cavity, creating an enlarged and distended abdomen, known as ascites (Figure 24.1). Worsening portal hypertension causes splenomegaly, and varices in the splanchnic bed, primarily in the walls of the esophagus (esophageal varices) and rectum (hemorrhoids). Physical examination reveals palmar erythema, spider angiomata (spider nevi) on the trunk, gynecomastia, testicular atrophy, hepatomegaly (a firm, hard enlarged liver), splenomegaly, and ascites with fluid wave. Serum bilirubin levels are increased and the patient appears jaundiced, AST and ALT levels are usually normal to or only modestly elevated. As the fibrosis worsens and liver function falls, the liver’s capacity to convert amino acids to urea falls and the serum blood urea nitrogen (BUN) falls below normal. A low BUN is a particularly ominous sign, indicating near total liver failure and an inability to metabolize toxins and medications. Eventually, esophageal varices may rupture, leading to significant upper gastrointestinal bleeding. Along with a low BUN, terminal patients develop hepatic encephalopathy and hepatorenal syndrome when the kidneys fail (Figure 24.2).
FIGURE 24.1 Factors involved in the development of ascites. (From Chung RT, Podolsky DK. Cirrhosis and its complications. In: Kasper DL, Braunwald E, Fauci AS, et al., eds. Harrison’s Principles of Internal Medicine. 16th ed. New York, NY: McGraw-Hill; 2005:1858-1869, with permission. Copyright © McGraw-Hill Education LLC.)
FIGURE 24.2 Clinical effects of cirrhosis of the liver. (Redrawn, with permission, from Chandrasoma P, Taylor CE. Concise Pathology, 3rd ed. Appleton & Lange; 1989. Copyright © McGraw-Hill Education LLC.)
Chronic/Recurrent Mild Hepatocellular Damage
Currently, one of the more perplexing situations to evaluate is the asymptomatic patient with a single abnormal finding or periodically recurring mildly elevated AST and ALT (<100 units/dL).
First, is this the patient’s normal value? The issue is already clouded by the skewing of the distribution, discussed earlier. In addition, the inherent limitations of the accuracy of the testing process further widens the range of actual “normal” values compared to listed normal ranges. Second, has the patient recently ingested more than normal levels of ethanol or is abusing ethanol and has the early stages of cirrhosis? Third, does the patient have chronic hepatitis B or C? Fourth, is it due to the statin the patient is taking? Finally, is it due to nonalcoholic liver disease because the patient has the metabolic syndrome? All of these are possible answers, which makes assessment more complex.
Nonalcoholic fatty liver disease (NAFLD) is one of the major causes of chronic or recurrent mild hepatocellular damage. NAFLD comprises two major variants. Simple steatosis, which is benign, comprises the majority of patients with NAFLD, whereas nonalcoholic steatohepatitis (NASH) is associated with significant inflammation and eventually leads to cirrhosis. The estimated incidence of NAFLD is 20% to 50%. Fortunately, the incidence of NASH is only 3% to 5%. NAFLD is associated with the metabolic syndrome and insulin resistance. Type 2 diabetes is a major risk factor for developing NASH. At this time, the only way to distinguish between the types of NAFLD is a liver biopsy and that may not be accurate in early stages of the disease. While there are some computerized calculations based on a combination of physical factors and laboratory tests, they are still being studied for accuracy and clinical utility. Similarly, hepatic elastography, which measures steatosis via liver tissue elasticity using computerized ultrasonography, can determine the extent of the disease but cannot distinguish between NAFLD variants. Generally, NASH should be suspected when ALT and AST levels exceed 100 units/dL but do not reach acute hepatocellular levels (>300 units/dL).
Recently, the US Preventive Services Task Force (USPSTF) recommended that all adults born between 1945 and 1965 get screened for hepatitis C, since 75% of the nearly 4 million people in the United States with hepatitis C were “baby boomers.” Hepatitis C is the leading cause of death from liver disease in the United States. Most patients (80%), regardless of the severity of symptoms of acute hepatitis C, go on to develop chronic hepatitis C, which can manifest itself in intermittent or chronic mild elevations in ALT and AST. Interestingly, up to one-third of patients with chronic hepatitis C have normal LFTs. If untreated, it may eventually cause cirrhosis and all its complications. Similarly, hepatitis B can become a chronic disease. The risk of developing chronic hepatitis B depends on the age of the initial infection. Twenty-five to fifty percent of children who become infected and 6% to 10% of children whose initial infection occurs after age 5 go on to develop chronic hepatitis B. If untreated, it too eventually leads to cirrhosis and its complications. Therefore, patients with mild chronic or intermittent mild elevations should be tested for hepatitis B and C. In chronic hepatitis C, anti-HCV antibodies, measured by either enzyme immunoassay (EIA) or radioimmunoblot assay (RIBA) will be positive. Due to the potential number of false positives with the EIA method, most experts recommend the HCV-RNA, which directly measures viral products through polymerase chain reaction (PCR) assay. In chronic hepatitis B, the hepatitis B surface antigen (HBsAg) is the primary screening test. If the HBsAg is positive, a panel of other hepatitis B antigens and antibodies is ordered to determine the specific stage of the infection (acute versus resolving versus chronic).
The role of ethanol ingestion as a cause of mild chronic or intermittent AST or ALT elevations is unclear. While guidelines suggest up to two alcoholic beverages a day is beneficial, the implication is that higher levels of intake will lead to hepatocellular damage and elevated ALT and AST values. Unfortunately, there is a great deal of variability among individuals regarding a dose-response relationship between ethanol ingestion and elevation of AST and ALT values. In some cases, patients who have ingested excessive amounts of ethanol for several days before LFTs are drawn have normal AST and ALT levels. In others, beneficial intake results in mildly elevated AST and ALT values. Patients should be questioned carefully regarding recent ethanol use. If ethanol is a suspected cause, have the patient discontinue ethanol intake for 4 to 6 weeks to allow the hepatocytes to recover, then repeat LFTs. Whatever the results of the follow-up LFTs, the significant numbers of patients with NAFLD and chronic hepatitis B/C make attribution of test results to ethanol problematic. Similarly, the above factors and the co-occurrence of dyslipidemia with the metabolic syndrome or type 2 diabetes led the FDA to recently remove the requirements for baseline and follow up LFTs in statin users. See Chapter 19 for further discussion of the hepatotoxicity of statins.
• SERUM RENAL FUNCTION TESTS
In order to interpret serum tests of renal function accurately, understanding the general pathophysiology of nephron loss is important. In slow onset or chronic renal disease when nephrons are damaged, the remaining nephrons increase their filtration rates. This “hyperfiltration phenomenon” allows the kidneys to lose up to 50% of their function before changes are seen on common markers in the blood, e.g., serum creatinine. Therefore, serum levels of markers of renal function such as creatinine, BUN, and cystatin C are relatively insensitive to the early stages of renal damage, but are more accurate in monitoring the progression of chronic renal failure.
Serum Creatinine
Creatinine is a metabolic breakdown product of creatine, a substance found in muscle cells. Creatine exists as creatine phosphate, which is the primary source of phosphorous used in the regeneration of adenosine triphosphate (ATP) to transform chemical energy into muscle action. Creatinine is produced in muscle as a breakdown product of creatine and in healthy patients the production and excretion of creatinine are equal, which makes the serum creatinine a good marker of kidney function. While the normal value is listed at 0.7 to 1.5 mg/dL, a variety of issues can impact “normal” levels. The first is the assay method used. In 2003, results varied up to 41% between the various assays used. A decade later, the maximal variance is less than 15%. Differences in muscle mass will change the levels of creatinine. Women, who have less muscle mass than men, will have lower values for serum creatinine. African-Americans tend to have more muscle mass than other ethnicities and thus have higher creatinine serum levels. Therefore, an African-American male body builder may have a serum creatinine of 1.8 mg/dL that is actually normal for him, while a 75-year-old thin frail female with a serum creatinine of 1.0 mg/dL may have significant renal impairment, all due to differences in muscle mass. In addition, about 15% of creatinine found in urine is secreted through the tubules and drugs such as trimethoprim and cimetidine, which block that secretion, can falsely elevate serum creatinine levels. Finally, serum creatinine is slow to change in acute renal disease and may take several days to accurately reflect changes in actual renal function. In spite of these variances, serum creatinine is used to estimate the creatinine clearance and the glomerular filtration rate to monitor the progression of chronic renal disease.
Blood Urea Nitrogen
The BUN was the first serum test used to measure renal function. It actually measures the levels of serum urea, a breakdown product of amino acid metabolism by the liver via the arginine-ornithine cycle. The BUN has both advantages and disadvantages, in estimating renal function compared to the serum creatinine. Its major advantage is that a large percentage is reabsorbed at the same rate as sodium, chloride, and water and is sensitive to urine flow; therefore, it responds more quickly to acute change in glomerular filtration such as volume depletion and decreases in renal blood flow due to hypotension, blood loss, dehydration, or congestive heart failure (CHF). Its major disadvantage is that the serum levels of urea vary with the amount of protein intake. Therefore, patients on high protein diets, such as Atkins, or on hyperalimentation will have an elevated BUN, but a normal serum creatinine. Similarly, patients with a significant upper gastrointestinal bleed will have an elevated BUN and normal serum creatinine due to the excess protein absorbed from digested red blood cells and other serum proteins. Severe liver damage (cirrhosis), drastically slows the body’s capacity to metabolize amino acids to urea and leads to falsely lowered BUN levels. In patients with cirrhosis, a BUN level below the lower limit of normal can be a sign of end-stage liver disease.
To adjust for the other causes of elevated BUN, the BUN/serum creatinine ratio is used. If both values are in the normal range the ratio does not help. The normal ratio is between 10 and 20 to 1. Any value greater than 20 indicates the BUN is elevated due to poor perfusion (pre-renal azotemia), or large protein intake rather than any inherent damage or disease in the kidney.
Estimated Glomerular Filtration rate
Measuring the glomerular filtration rate (GFR) is difficult and cumbersome. An intravenous injection of exogenous substances such as inulin, or I-iothalamate intravenously, then collection of blood and urine samples must be done at specific times. Therefore, they are relegated primarily to research use. Clinically, we used to measure creatinine clearance, which required an accurate 24-hour urine collection and a serum creatinine. The major source of error was failure to collect all the urine. Today GFR is estimated using parameters for age, weight, gender, race, body surface area, and serum creatinine levels, depending on the method used. There is some controversy regarding whether to use actual, ideal or lean body weight. These methods are generally equivalent to a creatinine clearance estimate of GFR and only require a serum creatinine (Table 24.3). There are three methods to measure estimated glomerular filtration rates (eGFRs) that are commonly used. The Cockcroft-Gault equation uses age, weight, and serum creatinine, an adjustment factor for females in a simple mathematical formula. The modified diet in renal disease equation (MDRD) uses a complex formula involving serum creatinine and age with adjustments for both females and African-Americans and is based on a study using I-iothalamate GFR measurements. Finally, there is the chronic kidney disease epidemiology collaborative equation (CKD-EPI), which uses a complex formula involving gender, serum creatinine, and age. Most recently, a formula was developed substituting cystatin C for creatinine and one combining creatinine and cystatin C. Cystatin C is a protease inhibitor produced at steady state in all nucleated cells. It is filtered at the glomerulus, but is neither reabsorbed like urea nor secreted by tubules like creatinine. It is a newer test with less experience but is theoretically more accurate. While these three methods provide similar results, the standardization of creatinine assays has impacted estimates using older methodology such as Cockcroft-Gault. Since 2008, the standardized serum creatinine values are slightly lower than unstandardized values previously used. Because of these changes, today the Cockcroft-Gault equation, which was based on unstandardized serum creatinine values, tends to slightly overestimate the creatinine clearance. However, most of the pharmacokinetic drug dosing protocols for patients with impaired renal function use the Cockcroft-Gault methodology. Until protocols are developed for drug dosing using the MDRD and CKD-EPI, the older methodology should still be utilized recognizing that Cockcroft-Gault slightly overestimates creatinine clearance. Remember that pharmacokinetic dosing based on any renal parameter has always been an approximation requiring adjustments based on clinical judgment and other objective measures, rather than absolute calculation. Finally, for patients with normal or near-normal renal function, most laboratories do not report actual eGFR (calculated using the MDRD), but use eGFR >60 cc/min. Actual calculated eGFRs are only reported for eGFRs <60 cc/min, since that is the agreed upon definition of mildly to moderately decreased renal function.
TABLE 24.3 | Formulas for Calculating Estimated Glomerular Filtration Rates (eGFRs) |
• URINE RENAL FUNCTION TESTS
One of the disadvantages of serum RFTs is their relative lack of sensitivity to early and small changes in renal function. Urinalysis, on the other hand, is an accurate method to detect early and small changes in renal function. Protein and formed elements in the urine are the primary tools used to indicate early changes in renal function.
Urine Protein/Albumin
The glomerulus is normally impermeable to large protein molecules, such as albumin. Low molecular weight globulins, which are small enough to pass the glomerulus, are mostly reabsorbed in the tubules, so protein levels in the urine are normally very low (<100 mg/24 hours). Protein excretion from the kidneys can be measured in three ways, a 24-hour urine collection, an estimated 24-hour excretion using a 4-hour collection, and by urine test strip in a random sample. Random sample urine test strips for protein can be read without additional equipment, but only detect excretion rates of >300 mg/24 hours. Clinical proteinuria (albumin and globulins) is defined as >500 mg/day. Care is needed in evaluating individual random urine dipstick readings, because exercise, running, fever, infection, and trauma (e.g., dirt bike riding, football) all cause transient increases in urinary protein and albumin excretion. A single 1+ test strip reading should result in questioning about activities known to facilitate excretion. If an identifiable cause cannot be found, then the dipstick should be repeated later. Repeated 1+ protein test strip readings warrant a further workup for impaired renal function. Since albumin in the urine is a more sensitive indicator, it is relied on for early detection of renal damage in conditions known to have renal complications such as hypertension and diabetes mellitus. Albuminuria is measured in two primary ways: the urinary albumin excretion rate (AER), which uses a 4-hour urine collection to estimate 24-hour excretion and the urinary albumin to creatinine ratio (ACR), which uses a single random sample. Macroalbuminuria is defined as excretion rates of >300 mg/g/24 hours, while microalbuminuria is defined as excretion rates of 30 to 299 mg/24 hours. Because of its increased sensitivity, tests for smaller amounts of albumin (microalbuminuria) is preferred. Whenever urine is collected over a 4- or 24-hour period, accuracy due to missed urine collections can be a problem. Therefore, it is probably best to routinely use random urine samples. Random tests for microalbuminuria that measure ACR also use test strips, but require a special analyzer to accurately measure the sample. Because the ACR uses creatinine, it tends to underestimate albumin excretion in males, due to their larger muscle mass.
Formed Elements
In addition to the presence of albumin in the urine, formed elements such as casts, red blood cells (RBCs), white blood cells (WBCs), and insoluble crystals can indicate kidney damage. Formed elements are seen with microscopic analysis of urine samples.
Hematuria A few RBCs can be found during microscopic urinalysis in patients without intrinsic renal damage. As with proteinuria and albuminuria, exercise, fever, and trauma can result in a few RBCs in the urine. However, even >2 to 3 RBCs/hpf in several samples can indicate renal damage. Significant hematuria may cause the urine to turn orange or pink depending on the number of RBCs. Similarly, urine residue containing RBCs may result in small red to dark reddish-brown stains on underwear. Also in women of childbearing age, RBCs from menstrual blood can be carried into the urine, usually accompanied by epithelial cells. Persistent hematuria requires further workup to rule out more serious problems such as neoplasm, infection, renal calculus, or trauma.
Pyuria WBCs in the urine are usually indicative of infection or inflammation in the urinary tract, occurring commonly in urinary tract infections. Pyuria is defined as >10 WBCs/hpf.
Casts Casts are cylindrical masses of glycoprotein formed in the tubules. The presence of casts in the urine is called cylindruria. Hyaline casts are common and carry no pathological significance. As casts form, they can surround RBCs, WBCs, and epithelial cells. The presence of WBC casts usually indicates infection or inflammation. RBC casts indicate significant damage to the kidney as do epithelial casts.
• MISCELLANEOUS DIAGNOSTIC TESTS
Medical imaging studies including ultrasound, CT scans, and MRI are used to detect masses and structural abnormalities. In addition, other substances found in the urine may indicate other systemic diseases, i.e., ketones in the urine may indicate diabetic ketoacidosis; bilirubin and its metabolites may indicate liver or biliary abnormalities.
• CAUSES OF ABNORMAL RENAL FUNCTION TESTS
When faced with lab test results that indicate potential renal damage, one must consider the problem to be potentially located anywhere in the urinary tract or it can be caused by other organ systems in the body. Decreased renal perfusion due to dehydration or CHF can cause temporary elevations in serum markers such as serum creatinine, BUN. Once hydrated or when CHF is treated, renal perfusion returns to normal along with the values of serum RFTS. Bleeding into the upper gastrointestinal tract can cause elevations in BUN levels and the BUN/creatinine ratio. Severe liver disease can cause abnormally low BUN values. Within the urinary tract, laboratory test abnormalities can be indicative of damage to the kidney itself, i.e., glomerulus, tubules, or interstitial tissue. Damage to these parts of the urinary tract can be caused by neoplasm, infection, autoimmune disease, and numerous medications and will result in abnormal serum and urine tests. In addition, problems with the ureters, bladder, and in males the prostate gland can result in abnormalities in both serum and urinary markers of renal function, but may require imaging to determine the location of damage. By themselves, RFTs usually cannot determine whether the renal injury is acute or chronic. Generally, that determination requires additional history, the clinical course of the event, and at times also requires other studies to determine the exact cause.
• KEY REFERENCES
1. Winger J, Michelfelder A. Diagnostic approach to the patient with jaundice. Prim Care. 2011;38:469-482.
2. Johnston DE. Special considerations in interpreting liver function tests. Am Fam Physician. 1999;59:2223-2230.
3. Thapa BR, Walia A. Liver function tests and their interpretation. Indian J Pediat. 2007;74:663-671.
4. Carey E, Carey WD. Non-invasive tests for liver disease, fibrosis and cirrhosis: is the liver biopsy obsolete? Cleve Clin J Med. 2010;77:519-527.
5. Lee WM. Drug-induced hepatotoxicity. N Engl J Med. 2003;349:474-485.
6. Navarro VJ, Senior JR. Drug-related hepatotoxicity. N Engl J Med. 2006;354:731-739.
7. Aboud G, Kaplowitz N. Drug-induced liver injury. Drug Saf. 2007;30:277-294.
8. Corsini A, Ganey P, Ju C, et al. Current challenges and controversies in drug-induced hepatic injury. Drug Saf. 2012;35:1099-1117.
9. Giboney PT. Mildly elevated liver transaminase levels in the asymptomatic patient. Am Fam Physician. 2005;71:1105-1110.
10. Vernon G, Baranova A, Younossi ZM. Systematic review: the epidemiology and natural history of non-alcoholic fatty liver disease and non-alcoholic steatohepatitis in adults. Aliment Pharmacol Ther. 2011;34:274-285.
11. Barsic N, Lerotic I, Smircic-Duvnjak L, et al. Overview and developments in non-invasive diagnosis of nonalcoholic fatty liver disease. World J Gastroenterol. 2012;18:3945-3954.
12. Ghany MG, Strader DB, Thomas, DL, Seef LB. Diagnosis, management, and treatment of hepatitis C. Hepatology. 2009;49:1335-1374.
13. Weinbaum CM, Williams I, Mast EE, et al. Recommendations for identification and public health management of persons with chronic hepatitis B virus infection. Morb Mortal Wkly Recomm Rep. 2008;57(RR08):1-20.
14. Killeen AA, Ashwood ER, Ventura CB, Styer P. Recent trends in performance and current state of creatining assays. Arch Pathol Lab Med. 2013;137:496-502.
15. Van Pottelbergh G, Van Heden L, Mathei C, Degryse J. Methods to evaluate renal function in elderly patients: a systematic literature review. Age Ageing. 2010;39:542-548.
16. Koyner JL. Assessment and diagnosis of renal dysfunction in the ICU. Chest. 2012;141:1584-1594.
17. Tesch GH. Review: serum and urine biomarkers in kidney disease: a pathophysiological perspective. Nephrology. 2010;15:609-616.
18. Shipak MG, Matsushita K, Arnlov J, et al. Cytostatin C versus creatinine in determining risk based on kidney function. N Engl J Med. 2013;369:932-943.
19. Stevens PE, Levin A. Evaluation and management of chronic kidney disease: synopsis of the kidney disease: improving global outcomes 2012 clinical practice guideline. Ann Intern Med. 2013;158:825-830.
20. Younes N, Cleary PA, Steffes MW, et al. Comparison of urinary albumin-creatinine ratio and albumin excretion rate in the diabetes control and complications trial/epidemiology of diabetes interventions and complications study. Clin J Am Soc Nephrol. 2010;5:1235-1242.
21. Rostoker G, Andrivet P, Pham I, Griuncelli M, Adnot S. Accuracy and limitations of equations for predicting glomerular filtration rate during follow-up of patients with non-diabetic nephropathies. BMC Nephrol. 2009;10:16-26.
22. Richardson M. Precision vs approximation: the trade off in assessing kidney function and drug dosing. Pharmacotherapy. 2010;30:758-761.
23. Nyman HA, Dowling TC, Hudson JQ, et al. Comparative evaluation of the Cockcrot-Gault equation and the modification of diet in renal disease study equation for drug dosing: an opinion of the nephrology practice and research network of the American College of Clinical Pharmacy. Pharmacotherapy. 2011;31:1130-1144.
24. Dowling TC, Matzke GR, Murphy JE, Burckart GJ. Evaluation of renal drug dosing, prescribing information and clinical pharmacist approaches. Pharmacotherapy. 2010;30:776-786.
25. Dowling TC, Wang E, Ferucci L, Sorkin JD. Glomerular filtration rate equations overestimate creatinine clearance in older individuals enrolled in the Baltimore longitutdinal study on aging: impact on renal drug dosing. Pharmacotherapy. 2013;33:912-921.
INTERPRETING LIVER AND RENAL FUNCTION TESTS
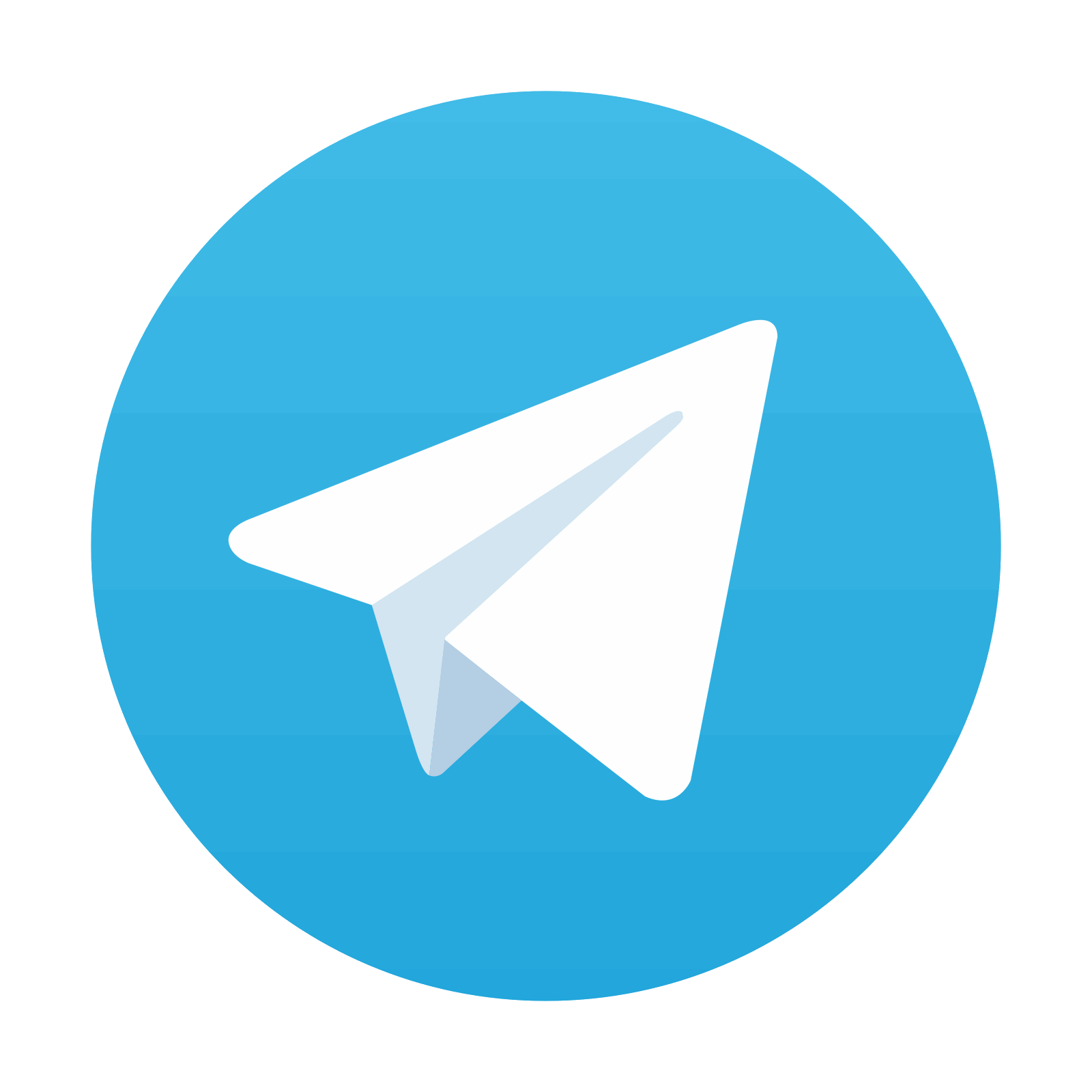
Stay updated, free articles. Join our Telegram channel

Full access? Get Clinical Tree
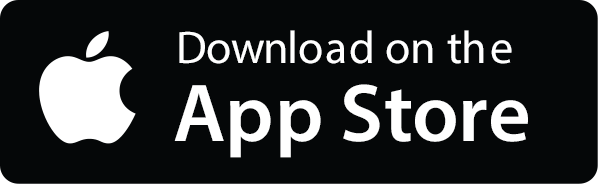
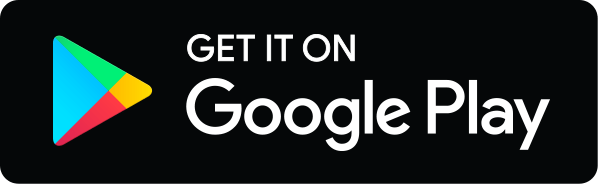