Chapter 25 Lipid Transport
The plasma levels of the major lipids are not only higher than the normal blood glucose level of 100 mg/dl (Table 25.1), but they fluctuate over a wider range, depending on nutrition, lifestyle, and individual constitution. This is possible because none of the major tissues depends on lipids as its only energy source, although some tissues depend on glucose.
Table 25.1 “Normal” Concentrations of Plasma Lipids in the Adult, Determined in the Postabsorptive State 8 to 12 Hours after the Last Meal
Lipid | Normal Range (mg/dl) |
---|---|
Total lipid | 400–800 |
Triglycerides | 40–280 |
Total cholesterol | 120–280 |
LDL cholesterol | 65–200 |
HDL cholesterol | 30–90 |
Phospholipids | 125–275 |
Free fatty acids | 8–25 |
HDL, High-density lipoprotein; LDL, low-density lipoprotein.
Most Plasma Lipids Are Components of Lipoproteins
The general structure of a lipoprotein (Fig. 25.1) can be predicted from the solubility properties of the lipids. The hydrophobic triglycerides and cholesterol esters always avoid contact with water. They form the core of the lipoprotein. The amphipathic phospholipids prefer the water-lipid interface. They form a monolayer that covers the surface of the particle. The protein components, or apolipoproteins, are also amphipathic and reside on the surface of the particle. Large lipoprotein particles with a high volume/surface ratio have a high content of nonpolar lipids, and small particles contain mainly polar lipids and protein.
The plasma lipoproteins can be separated by electrophoresis, along with the other plasma proteins (see Chapter 15). In fasting serum or plasma, the two most prominent lipoprotein bands are in the α1 and β fractions. They are designated as α- and β-lipoproteins. A weaker band, the pre–β-lipoproteins, moves slightly ahead of the β-lipoproteins. The chylomicrons, found only after a fatty meal, do not move upon electrophoresis.
Density gradient centrifugation separates the lipoproteins according to their protein/lipid ratio. Nonpolar lipids have densities near 0.9 g/cm3. For lipoprotein particles, the densities increase from 0.95 g/cm3 in the most lipid-rich particles to well above 1.0 g/cm3 in the protein-rich types (Table 25.2). Based on their order of density and protein content, we can distinguish chylomicrons, very-low-density lipoprotein (VLDL), low-density lipoprotein (LDL), and high-density lipoprotein (HDL). The correspondence of these density classes to the electrophoretic separation pattern is shown in Figure 25.2.
Lipoproteins Have Characteristic Lipid and Protein Compositions
Table 25.2 lists the approximate compositions of the lipoprotein classes. In the fasting state, most of the plasma triglyceride is in VLDL, whereas 70% of the total cholesterol is in LDL. Therefore, elevations of plasma triglycerides usually are caused by increased VLDL, and elevations of cholesterol usually are caused by increased LDL.
Each lipoprotein class has its own characteristic apolipoproteins (Table 25.3). Chylomicrons contain apoB-48; LDL and VLDL contain apoB-100; and HDL contains the A-apolipoproteins. However, with the exception of the B-apolipoproteins, most apolipoproteins can be exchanged among the lipoprotein classes. The apolipoproteins
Dietary Lipids Are Transported by Chylomicrons
Approximately 100 g of dietary triglycerides is transported daily from the small intestine to other tissues. As discussed in Chapter 23, they are transported as constituents of chylomicrons. Chylomicrons are present only after a fatty meal. The fate of chylomicrons is shown in Figure 25.3. They are formed with apoB-48 and the A-apolipoproteins as their only apolipoproteins. ApoE and the C-apolipoproteins are acquired in the blood by transfer from HDL.
VLDL Is a Precursor of LDL
The liver synthesizes 25 to 50 g of triglycerides and smaller amounts of other lipids per day. These lipids are released as VLDL. Like the chylomicrons, VLDL is synthesized in the endoplasmic reticulum (ER) and Golgi apparatus and is released by exocytosis (Fig. 25.4). The liver sinusoids have a fenestrated endothelium that allows the passage of lipoproteins into the sinusoidal blood.
Like the chylomicrons, VLDL is metabolized by LPL, although VLDL triglycerides are hydrolyzed a bit more slowly than those in chylomicrons (see Fig. 25.4). Like chylomicrons, VLDL transfers C-apolipoproteins to HDL during triglyceride hydrolysis but retains most of its apoE.
About half of the VLDL remnants, especially larger specimens with multiple copies of apoE, are taken up by the liver. Smaller remnant particles appear initially as intermediate-density lipoprotein (IDL) and eventually are remodeled to LDL. This requires the hydrolysis of excess triglyceride and phospholipid by the hepatic lipase (HL) and the transfer of excess apolipoproteins to HDL (see Fig. 25.4).
LDL Is Removed by Receptor-Mediated Endocytosis
LDL has a well-defined structure. Its only apolipoprotein is a solitary apo B-100 molecule, and its lipid component includes a high proportion of cholesterol and cholesterol esters (see Table 25.2).
LDL that has been chemically modified by acetylating or oxidizing agents or by exposure to the cross-linking agent malondialdehyde (formed during lipid peroxidation; see Chapter 23) has a higher affinity for scavenger receptors than does virgin LDL. Therefore one likely function of these receptors is the removal of aberrant or aged lipoproteins that are no longer good ligands for the other lipoprotein receptors. The scavenger receptors bind not only lipoproteins but also other particles with negative surface charges, including some bacteria. Therefore they can participate in the defense against infections.
Cholesterol Regulates Its Own Metabolism
The concentration of free cholesterol in the cellular membranes is tightly regulated. To this effect, free (unesterified) cholesterol regulates the synthesis of three important proteins (Fig. 25.5):
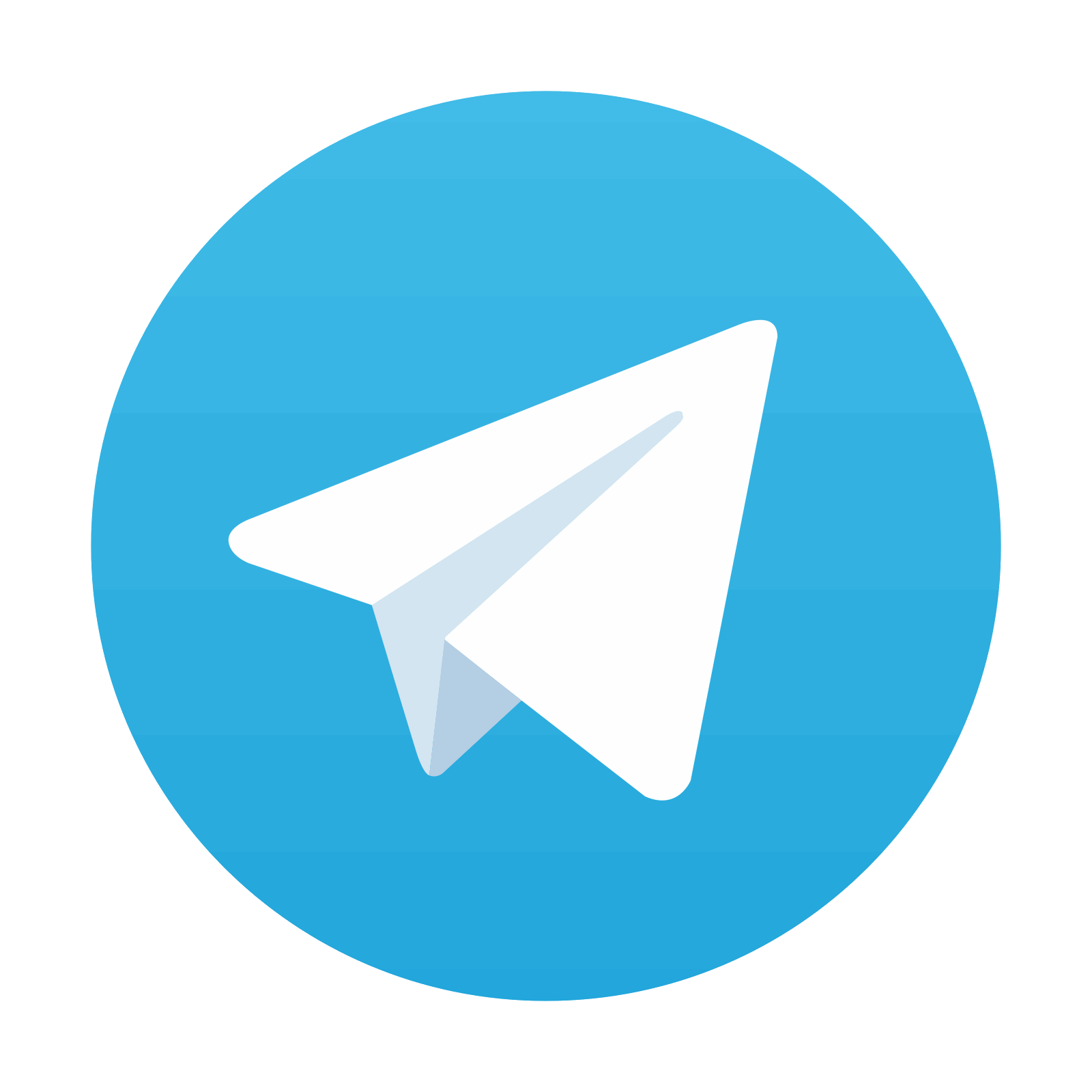
Stay updated, free articles. Join our Telegram channel

Full access? Get Clinical Tree
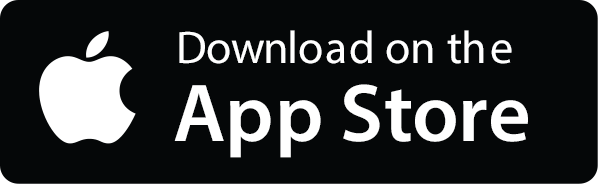
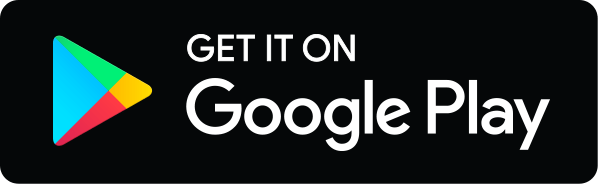