Fig. 25.1
(a) Protein Electrophoresis (PEL) and Immunofixation electrophoresis (IFE) of normal serum: the proteins in the gamma fraction on the PEL gel and the precipitated immunoglobulins on the IFE gel exhibit a smooth Gaussian distribution indicating a polyclonal distribution. (b) Protein Electrophoresis (PEL) and Immunoelectrophoresis (IFE) of a serum containing a monoclonal IgG Lambda: the proteins in the gamma fraction on the PEL gel and the precipitated immunoglobulins on the IFE gel exhibit a discrete band indicating a monoclonal protein. The upper most graph is a scan of the PEL gel, and the area under the monoclonal protein spike is used in conjunction with the serum total protein concentration to quantitate the amount of monoclonal immunoglobulin. (c) Protein Electrophoresis (PEL) and Immunoelectrophoresis (IFE) of patient with Primary Amyloidosis (AL): the proteins in the gamma fraction on the PEL gel and the precipitated immunoglobulins on the IFE gel exhibit only a small abnormality in the beta region reflecting the low plasma cell burden in this patient
Not all plasma cell proliferative disorders, however, produce a quantifiable or even detectable monoclonal protein. Nonsecretory multiple myeloma, for example, does not secrete an easily detectable monoclonal protein even though the bone marrow may be packed with malignant plasma cells containing cytoplasmic monoclonal immunoglobulin light chain. In AL patients with significant disease, it may also be difficult to detect a monoclonal protein and often impossible to quantitate. An AL patient with very small amounts of monoclonal free lambda light chain is illustrated in Fig. 25.1c. There is an almost imperceptible asymmetry in the lambda lane of the IFE, and there is clearly no way to monitor and quantitate the hematologic response of this patient.
The distribution of monoclonal gammopathies in our clinical practice is listed in Table 25.1. By far, the most common plasma cell proliferative disorder is monoclonal gammopathy of undetermined significance (MGUS). In the normal population older than 50 years, MGUS has a prevalence of 3 % [1]. The prevalence increases with age: between ages, 50 and 60 the prevalence is 1.7 % and in ages greater than 80 years, the prevalence is 6.6 %. This very common premalignant disorder has no clinical symptoms, but these patients have a 1 % per year progression to diseases such as multiple myeloma or AL [2]. The diagnosis of MGUS requires the absence of related organ or tissue impairment (e.g., no end organ damage or bone lesions), less than 10 % clonal bone marrow plasma cells, and less than 3 g/dL of serum monoclonal protein. The diagnosis of multiple myeloma requires greater than 10 % clonal bone marrow plasma cells as well as clinical symptoms such as hypercalcemia, renal disease, anemia, and/or bone lesions due to the clonal plasma cell proliferation. Multiple myeloma has an incidence of approximately 40 per million per year in Caucasians, and the incidence is 2–3-fold higher in African American populations [3]. There are approximately 13,000 new cases each year in the USA.
Table 25.1
Distribution of plasma cell proliferative disorders in clinical practice
Monoclonal gammopathy of undetermined significance | 61 % |
Multiple myeloma | 17 % |
Amyloidosis AL | 9 % |
Lymphoproliferative disease | 3 % |
Smoldering myeloma | 4 % |
Solitary or extramedullary plasmacytoma | 2 % |
Macroglobulinemia | 2 % |
Other | 2 % |
By contrast, AL is a relatively rare plasma cell proliferative disorder. It is one-fifth as common as multiple myeloma with approximately 2500 new patients diagnosed in the USA every year. Multiple myeloma and AL can occur together, with the myeloma commonly being diagnosed before or at the time AL is discovered [4, 5]. Like all forms of amyloid, diagnosis requires histologic identification of amyloid fibrils in tissue [6]. Because AL is a monoclonal gammopathy, the identification of a monoclonal protein is a useful initial screen for patients with AL. The 3 % prevalence of MGUS in the population over 50 years and the increased prevalence in African Americans, however, mean that some of these coincident findings will be unrelated. Over a 6-year period at Memorial Sloan-Kettering Cancer Center, 369 consecutive patients with systemic amyloidosis were evaluated for clonal plasma cell disease and 30 % of them were screened for hereditary transthyretin mutations (ATTRm). ATTRm was identified in 5.4 % (20/369) of the patients. Of the 20 patients with ATTRm, 50 % (10 of 20) had an associated monoclonal gammopathy. Ultimately, four of the six with a gammopathy and mutant TTR were diagnosed with ATTRm, while the other two had AL [7]. This study highlights a potential pitfall of relying on monoclonal protein screening alone. Immunohistochemical staining or some other direct characterization of the amyloid fibrils may be necessary to confirm the type of amyloidosis [8].
The fibrils in AL are derived from intact or fragmented monoclonal immunoglobulin light chains. These patients often have free monoclonal immunoglobulin light chains detected in the serum and/or urine. The FLCs are secreted from the plasma cells without being bound to heavy chain in intact immunoglobulin molecules and the monoclonal light chains or fragments are precursor components for the amyloid fibrils. Unlike patients with multiple myeloma, AL patients may have a very small population of clonal plasma cells in the bone marrow. The long-term continuous secretion of the amyloidogenic monoclonal FLC results in amyloid fibril deposition and eventual organ failure. The presence of small clonal plasma cell populations and the small amount of secreted monoclonal FLC may make it difficult to document the monoclonal gammopathy by serum and/or urine IFE [9].
Free Light Chain Quantitation
Bence Jones proteins are monoclonal FLC that are detected in concentrated urine using PEL and IFE. These urinary FLCs are low molecular weight peptides that are removed from serum by the kidneys and are either metabolized or excreted in the urine. An alternative strategy to detect and quantitate the Bence Jones proteins would be to directly quantitate the FLC in serum. Early approaches used the size difference between intact immunoglobulins and FLC to separate the molecules, and then quantitated the FLC with light chain immunoassays. These multistep procedures never migrated into clinical laboratories. Subsequent approaches were based on using antisera that were specific for immunoglobulin light chain epitopes that were obscured when the light chain peptides were bound to the heavy chain peptides (Fig. 25.2). The specificity for these “cryptic” light chain epitopes meant that FLC could be accurately quantitated even in the presence of the large concentrations of intact immunoglobulin found in serum. The introduction of automated assays for the quantitation of immunoglobulin FLC has given clinical laboratories a new tool for evaluating AL. The FLC assays have increased the diagnostic sensitivity for identification of light chain diseases such as AL, and in addition they have improved disease monitoring and prognosis. The FLC assay was described by Bradwell and colleagues in 2001 [10]. The method is an automated nephelometric assay that uses a commercially available reagent set of polyclonal antibodies to quantitate both κ FLC and λ FLC by immunonephelometry (FREELITE™: The Binding Site, San Diego, Calif.). The antibodies show no reactivity by IFE or by Western blots to light chains contained in intact immunoglobulin. Sensitive hemagglutination assays showed reactivity to the appropriate FLC at dilutions above 1:16,000 and no reactivity to light chains contained within intact Ig at antisera dilutions >1:2. The assay reagents therefore appear to have a minimum of a 10,000-fold difference in reactivity to FLC compared to light chain contained within intact Ig. This high specificity allows κ and λ FLC to be quantitated in the presence of a large excess of serum IgG, IgA, and IgM. Similar to the quantitation of elevated immunoglobulins, elevations in FLC concentrations do not indicate monoclonality. The relationship between κ and λ light chain secretion, however, is predictable in normal plasma cell populations. The ratio of κ to λ light chain synthesis is approximately 2:1. Significant deviations from this ratio suggest clonal light chain synthesis. If the ratio of κ to λ FLC (rFLC) is significantly greater than normal, it suggests clonal proliferation of a κ-producing plasma cell. If the ratio is below normal, it suggests clonal excess of λ FLC.


Fig. 25.2
Cartoon of immunoglobulin G showing the intact tetrapeptide as well as FLC. The hidden surfaces of the light chains are tightly bound to the heavy chains by noncovalent interactions. When no longer bound to heavy chain, these surfaces provide the specific targets for the FLC antisera (Courtesy of The Binding Site, Inc., reprinted with permission)
A reference range study was performed with sera from healthy donors aged 21–90 years [11] whose sera were screened by PEL and IFE to exclude samples with a monoclonal protein [e.g., unknown MGUS patients]. The κ FLC and λ FLC concentrations were assessed, and the rFLC was calculated. This reference range study revealed an apparent effect of age on the 2 FLC concentrations, but the rFLC showed no age dependence. Cystatin C (a marker of renal clearance) showed the same apparent age dependence as the 2 FLC concentrations. The increase in serum FLC concentrations with age is most likely due to reduced renal clearance, and the use of the rFLC ratio normalizes the effects of reduced clearance.
Concentrations of κ and λ FLC may be abnormal due to immune suppression, immune stimulation, reduced renal clearance, or monoclonal plasma cell proliferative diseases. Serum from patients with either polyclonal hypergammaglobulinemia or renal impairment for example, often have elevated κ FLC and λ FLC due to increased synthesis or reduced renal clearance, respectively. The rFLC, however, usually remains normal in these conditions [11]. A significantly abnormal rFLC should only be due to a plasma cell proliferative disorder that secretes excess clonal FLC and disturbs the normal balance between κ and λ secretion. An abnormally high ratio suggests expansion of a kappa-producing plasma cell clone, whereas an abnormally low ratio suggests expansion of a lambda-producing plasma cell clone. The κ and λ FLC reference ranges were defined as 95 % reference ranges, but a “diagnostic range” for the rFLC was defined by 100 % of the normal sample study (Table 25.2).
Table 25.2
FLC quantitation: serum FLC reference intervals and diagnostic range
95 % reference interval (normal range) | Diagnostic range | |
---|---|---|
Kappa FLC | 0.33–1.94 mg/dL | |
Lambda FLC | 0.57–2.63 mg/dL | |
FLC K/L ratio | 0.3–1.2 | 0.26–1.65 |
Recently, Barnidge et al. [12] described the application of a mass spectrometry (MS)-based platform routinely used by pharma for monoclonal therapeutic quality control, microLC-ESI-Q-TOF MS, for the rapid detection, quantification, and isotyping of monoclonal immunoglobulin in patient sera. Using reduced Ig-enriched serum, these authors utilized the mass distribution measurements to identify isotype and quantify the distribution of light chains. Figure 25.3 demonstrates the mass spectrum distribution in the presence and absence of a monoclonal protein. Limits of detection were significantly lower than existing serum measurements and the technique was able to detect residual M-protein in samples negative by PEL, IFE, and FLC. This methodology represents a major shift in the characterization of monoclonal immunoglobulins. Rather than using gel electrophoresis and anti-isotypic antibody reagents, the authors combine molecular mass and constant region fragment ions to create a more sensitive technique for detecting and monitoring monoclonal immunoglobulins.


Fig. 25.3
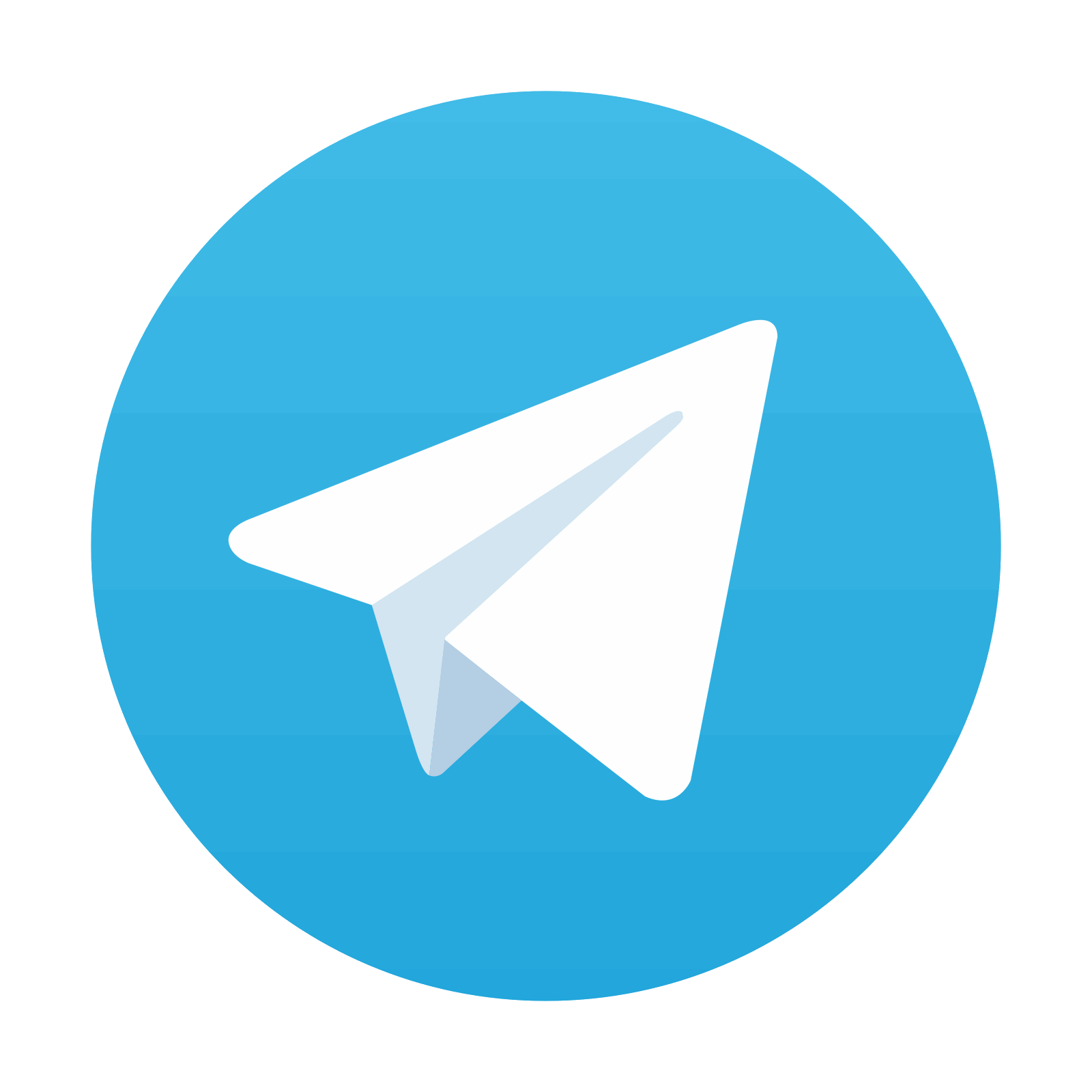
Mass spectra from (a) a normal serum sample and (b) normal serum spiked with 0.5 g/dL of the IgG kappa recombinant mAb adalimumab. The normal serum mass spectrum displays a broad range of unresolved peaks, whereas the normal serum spiked with 0.5 g/dL of adalimumab shows a clearly defined multiply charged protein ions. (c) Converted spectrum for the normal serum sample displaying a broad range of unresolved peaks. (d) Converted mass spectrum for the normal serum spiked with 0.5 g/dL of adalimumab showing a single peak at an average molecular mass of 23 412.19 Da. This mass is in excellent agreement with the calculated average molecular mass of 23 412.13 Da for the kappa light chain of adalimumab (reprinted with permission from Ref. [12])
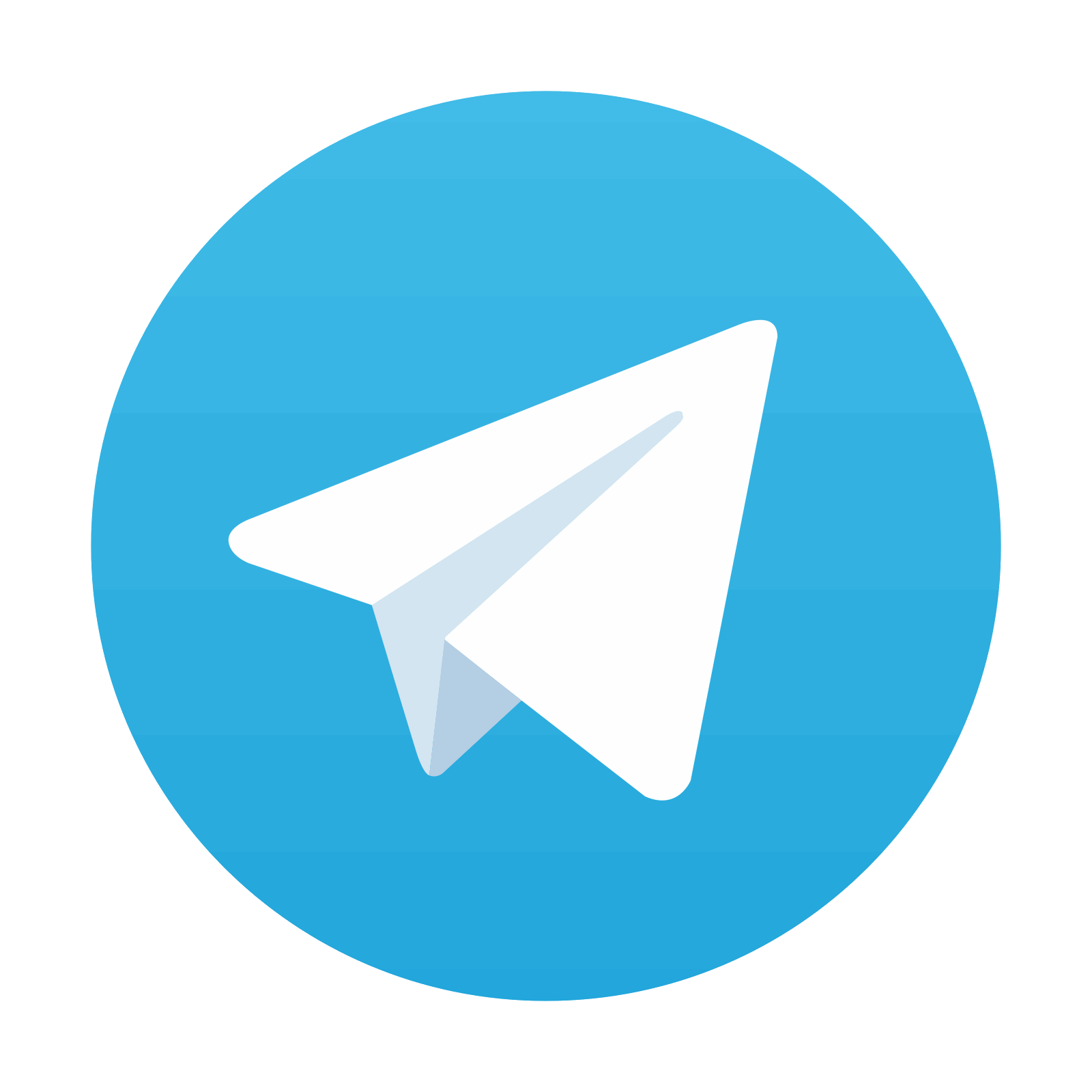
Stay updated, free articles. Join our Telegram channel

Full access? Get Clinical Tree
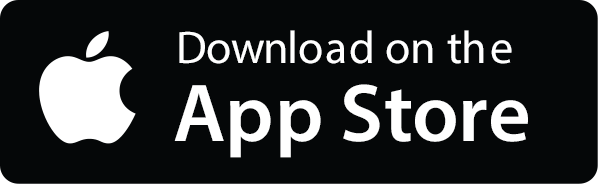
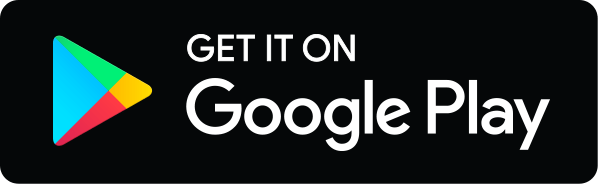
