Kidney Function Assessment by Creatinine-Based Estimation Equations
Chronic kidney disease (CKD) has been recognized as a common health care problem, and therefore the National Kidney Foundation (NKF) has published the Kidney Disease Outcomes Quality Initiative (KDOQI) Practice Guidelines for the early detection, evaluation, diagnosis, and treatment of this condition (http://www.kidney.org/professionals/kdoqi/guidelines.cfm). As recommended by this initiative, the degree of kidney dysfunction, as assessed by the estimation of the glomerular filtration rate (GFR), is essential for the diagnosis, classification, and staging of CKD; see elsewhere in this section (“Chronic Kidney Disease”). Among the various alternatives available for the assessment of GFR, the NKF, and the National Kidney Disease Education Program (NKDEP) recommend that renal function be estimated by creatinine-based GFR estimation equations, and these are the focus of this chapter.
EVALUATION OF GLOMERULAR FILTRATION RATE
Among the many physiologic roles of the renal system, GFR is considered the best indicator of overall kidney function and therefore its assessment has become an important clinical tool in the daily care of patients. GFR cannot be measured directly, but instead it can be assessed by the renal clearance of filtration markers.1 The total kidney GFR is the sum of the filtration rates of all single functional nephrons—that is, it is determined by the total number of functional nephrons. Because of its highly dynamic and adaptive nature, despite initial structural damage to the renal parenchyma (i.e., reduction in functional nephron number), an individual’s total GFR may not proportionally decrease because of the compensatory features of the remaining renal units, enabling the kidneys to maintain kidney function temporarily despite the loss of functional tissue. Moreover, the GFR may also be affected in the absence of parenchymal renal disease because of hemodynamic, pharmacologic factors, or both. The clinical assessment of GFR can aid the clinician in measuring the degree of renal dysfunction, progression of established kidney disease, or both; however, it is not informative in the determination of the cause of kidney disease, making it imperative to interpret the GFR in the context of the clinical setting.
The GFR can be determined from the renal clearance of a marker that achieves stable plasma concentration, is inert, and is freely filtered by the glomeruli but not reabsorbed, secreted, or metabolized.1,2 Such an ideal endogenous marker does not exist. Serum creatinine, one of the clinically useful analytes (others are serum urea and, more recently, serum cystatin C), has long been used by clinicians as a marker of renal function. However, it is important to emphasize that often the isolated use of serum creatinine concentration may not reflect the actual degree of kidney function of a particular subject. This is because multiple factors affect the concentration of serum creatinine (Table 1) and that the inverse relation between serum creatinine and GFR is nonlinear, particularly when patients have near-normal renal function (Fig. 1). An alternative to this approach is the measurement of creatinine clearance. This determination does not require highly trained personnel or expensive assays and can be performed by standard laboratories. However, this approach is limited by the difficulties in obtaining accurate urine collections and its potential misinterpretation because of the large biologic variability of creatinine metabolism in various clinical settings, including the unpredictable level of creatinine secretion at different levels of GFR. This method, however, is widely available and familiar to the health care community. On the other hand, more exact methods of GFR measurement, such as the clearance of exogenous markers such as inulin or renally excreted isotopes, are expensive and usually not readily available. More novel serum measurements of analytes, such as cystatin C, are under investigation and are not yet fully validated in all clinical settings; therefore, this approach remains a research tool at present.
Table 1 Factors Affecting Serum Creatinine Concentration
Factor | Effect on Serum Creatinine Level | Comment |
---|---|---|
Demographics | ||
Aging | Decreased | Caused by decline in muscle mass |
Female sex | Decreased | Reduced muscle mass |
Ethnicity* | ||
African American | Increased | Higher average muscle mass in African Americans |
Hispanic | Decreased | |
Asian | Decreased | |
Body Habitus | ||
Muscular | Increased | Increased muscle mass |
Muscle wasting, amputation, malnutrition | Decreased | Reduced muscle mass ± decreased protein intake |
Obesity | No change | No change in muscle mass |
Diet | ||
Vegetarian | Decreased | Decrease in creatinine generation |
Ingestion of cooked meats | Increased | Transient increase in creatinine generation |
Medications, Endogenous Substances | ||
Cimetidine, trimethoprim, probenecid, potassium-sparing diuretics | Increased | Reduced creatinine tubular secretion |
Ketoacids, ascorbic acid, glucose, some cephalosporins | Increased | Interference with alkaline picrate assay (Jaffé reaction) for creatinine |
Bilirubin, hemoglobin | Decreased | Interference with alkaline picrate assay (Jaffé reaction) for creatinine |
Flucytosine, praline, hemoglobin | Increased | Interference with enzymatic assays for creatinine |
Metamizole, methyldopa, ethamsylate | Decreased | Interference with enzymatic assays for creatinine |
* Whites used as reference group.
CREATININE-BASED ESTIMATION EQUATIONS
Numerous kidney function estimation equations, used to estimate creatinine clearance or GFR, have been published over the past few decades. However, the most commonly used formulas, the Cockcroft-Gault formula and the modification of diet in renal disease (MDRD) study equation, have been recommended by the KDOQI practice guidelines to be used for the estimation of GFR. These equations rely heavily on the reciprocal relation between serum creatinine and GFR but also incorporate demographic and anthropometric variables to complement the GFR estimation derived from the use of serum creatinine alone. It is important to emphasize that these mathematical models will reflect the clinical setting used to originate them.3
Cockcroft-Gault Formula
The Cockcroft-Gault formula was developed in 1976 with data from 249 men, primarily in an inpatient setting, with a wide range of renal function.4 It uses age, the inverse of serum creatinine, and lean body weight to estimate creatinine clearance in milliliters per minute (Box 1); it was not originally intended to be adjusted for body surface area (BSA). The inclusion of the weight factor is intended to adjust for muscle mass, a determinant of serum creatinine concentration. This implies that in clinical situations in which a change in weight is not the result of a similar change in muscle mass (e.g., edematous states, pregnancy, third spacing, overweight, obesity), the weight factor will adversely affect the performance of this formula. Because the original mathematical model was derived from data obtained predominantly in a male population, an arbitrary adjustment for female sex by a factor of 0.85 was incorporated. This equation has become popular because of its simple mathematical formulation and bedside applicability. It is important to note that this formula estimates creatinine clearance; this is known to overestimate GFR because of tubular secretion of creatinine, which is not adjustable.
Box 1 Creatinine-based Glomerular Filtration Rate (GFR) Estimation Equations
Cockcroft-Gault formula = ([140 − age] × weight)/(72 × SCr)*
Abbreviated MDRD equation = 186 × (SCr−1.154) × (age−0.203)†
Re-expressed MDRD equation‡ = 175 × (SCr−1.154) × (age−0.203)†
Note: Weight in kilograms, age in years.
MDRD, modification of diet in renal disease; SCr, serum creatinine level, expressed in mg/dL.
* Multiply by 0.85 if female; expressed in mL/min.
† Multiply by 0.742 if female, by 1.212 if African American; expressed in mL/min/1.73 m2.
‡ To be used with assays that have been calibrated to be traceable to an isotope dilution mass spectrometry (IDMS) reference method (gold standard), as specified by the National Institute of Standards and Technology (NIST).
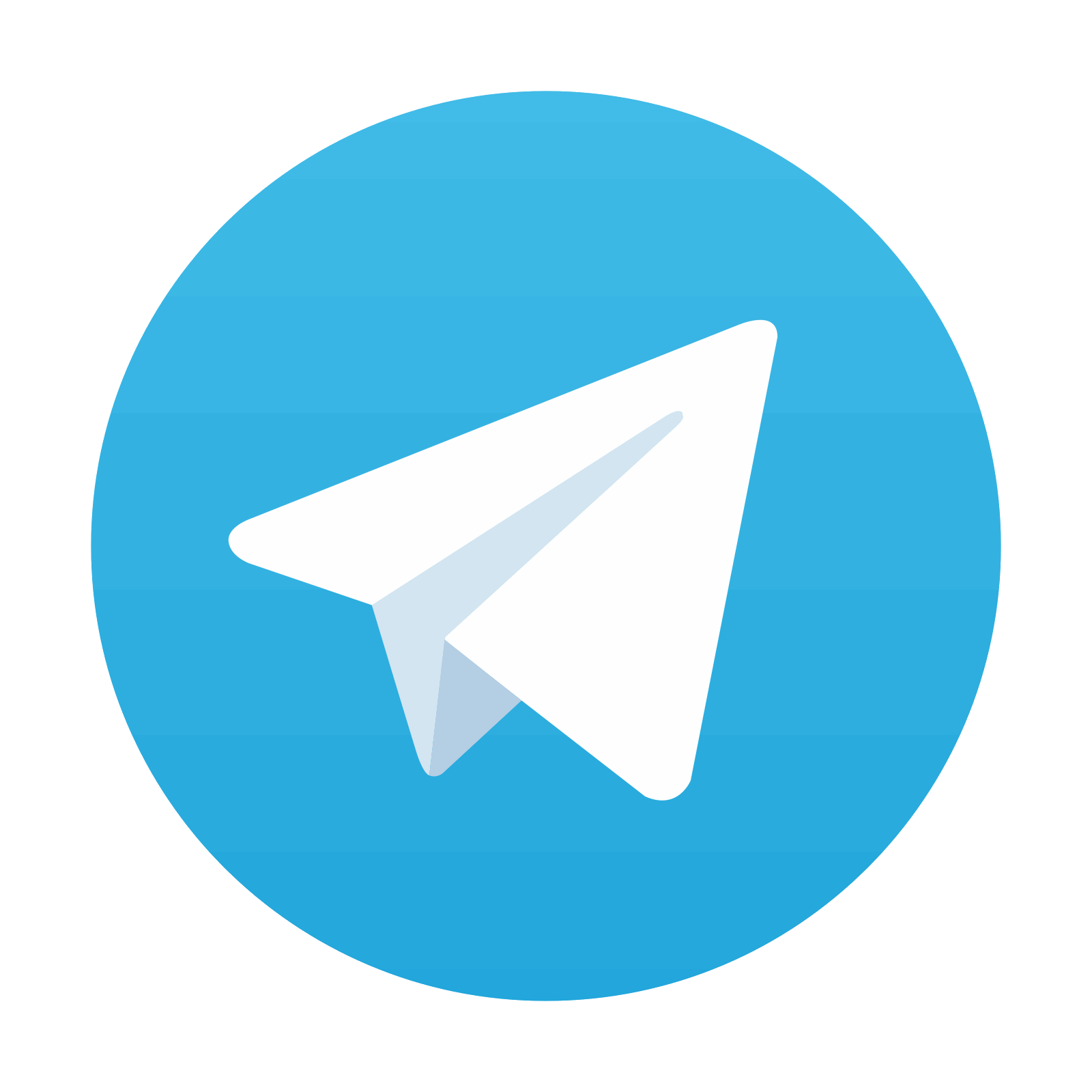
Stay updated, free articles. Join our Telegram channel

Full access? Get Clinical Tree
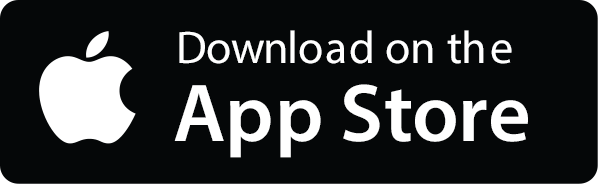
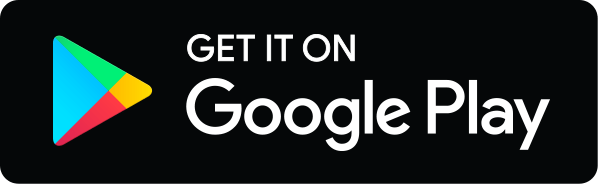