Male gonadal function
Spermatogenesis and its control
Spermatogenesis takes place in the seminiferous tubules, and requires normal functioning of both the Leydig and the Sertoli cells.
Leydig cells produce testosterone, the principal androgen, under the control of LH. Sertoli cells provide other testicular cells with nutrients, and also produce several regulatory proteins, of which inhibin, activin, Mullerian inhibitory hormone (MIH) and androgen-binding protein (ABP) are the best characterised. Sertoli cell function is regulated by FSH. Testosterone has crucial paracrine actions in the testes which are required for normal spermatogenesis and fertility.
The entire hypothalamic–pituitary–testicular axis (Figure 10.1) must function normally for spermatogenesis. GnRH from the hypothalamus stimulates the release of LH and FSH; its effect on LH release is more marked than that on FSH release. The secretion of GnRH, and thus of LH, occurs in pulses; the secretion of FSH is less markedly pulsatile. The amplitude and frequency of the pulses of LH release appear to be important in exerting effects on testosterone production.
The secretion of LH is under negative feedback control from plasma [free testosterone], and the release of FSH is inhibited by inhibin and stimulated by activin, both released by Sertoli cells. High testicular [testosterone] is ensured by the anatomical proximity of Leydig, Sertoli and spermatogenic cells, and by the local release of ABP. Inhibin is a dimeric glycopeptide comprising a 20 kDa α-subunit and a 15 kDa β-subunit. Two forms of β-subunit occur, thus two forms of inhibin (inhibin A and inhibin B) occur, although in males inhibin B is the most important form in plasma. Both FSH and testosterone are necessary for inhibin production in normal men. Activin is a dimer of inhibin β-subunits and has a stimulatory action on FSH release. MIH causes regression of the Mullerian ducts, and in the absence of MIH and testosterone the ducts differentiate into female internal genitalia.
Figure 10.1 The hypothalamic–pituitary–testicular axis. SHBG = sex hormone-binding globulin. Activin from Sertoli cells stimulates FSH release.
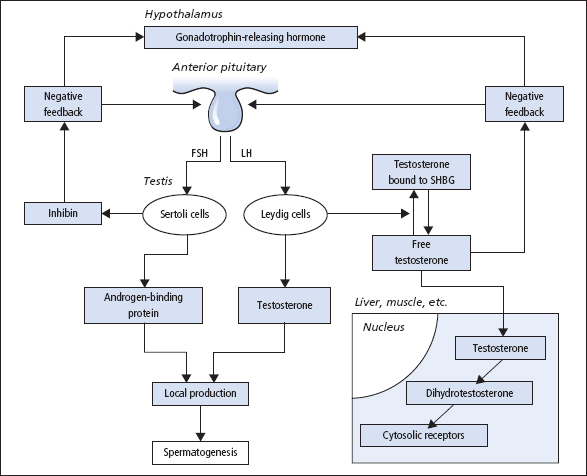
Transport, metabolism and actions of testosterone
In the circulation in males, about 60% of testosterone is strongly bound to sex hormone-binding globulin (SHBG), 38% is weakly bound to albumin while approximately 2% is unbound (free). These proportions vary somewhat and are dependent on the relative concentrations and affinities of albumin and SHBG. Since a large proportion of testosterone is bound to SHBG, the factors that alter the concentration or affinity of SHBG will have a significant effect on the circulating total testosterone concentration. Factors that modify the concentration of SHBG are shown in Table 10.1. There is continued debate concerning which components of circulating testosterone are capable of exerting bioactivity on target tissues. Classically androgens have been considered to exert their effect through interactions with a cytosolic receptor that then translocates to the nucleus and interacts with androgen-responsive genes. As such it was believed that only the small unbound ‘free’ fraction could enter cells and exert a biological effect. However, some consider that both the ‘free’ and ‘albumin-bound fraction’ of testosterone may be able to enter cells and thus be the ‘bioavailable testosterone’ fraction. Few laboratories measure the ‘free’ testosterone concentration, as this is technically demanding. Some laboratories may provide a measure of ‘bioavailable testosterone’. Most laboratories will offer a ‘free androgen index’ (FAI) which requires the measurement of [SHBG] and [total testosterone] and applying these in the formulae:
Table 10.1 Causes of abnormal SHBG in males and females
Increased SHBG | Decreased SHBG |
Anorexia nervosa | Obesity |
Hyperthyroidism | Hypothyroidism |
Hypogonadism (males) | Polycystic ovarian syndrome (females) |
Cirrhosis | Glucocorticoids |
Anti-convulstants | Androgens |
Oestrogens |
This effectively corrects for changes in SHBG but it does not take into account changes in the albumin-bound fraction. The FAI is unreliable in males and tends to overestimate the serum [free testosterone]; its use should be confined to females. More complicated mathematical formulae based on the law of mass action have been produced that can calculate [free testosterone] and estimate [bioavailable testosterone] from measured serum [total testosterone], [albumin] and [SHBG]. These formulae do not take into account changes in the affinity of SHBG and albumin but appear to work well for male subjects.
Androgens are thought to exert their action in target tissues through high-affinity cytosolic receptors that transport the androgens into the cell nucleus. In the nucleus, the androgens then interact with androgen receptors, which in turn modify the expression of androgen-responsive genes. In many tissues, testosterone is converted to the more biologically active compound 5α-dihydrotestosterone (5α-DHT) by 5α-reductase. It would seem that some actions of testosterone might be mediated through oestrogen receptors after local conversion of testosterone to oestrogen by the enzyme ‘aromatase’. It has thus become apparent that many of the actions of testosterone may be regulated in target tissues by both 5α-reductase and aromatase. In addition, since oestradiol and testosterone bind to SHBG with differing affinities, changes in the concentration of SHBG may modify the relative clearance rates of testosterone and oestradiol and thus alter the ratio of these hormones in plasma; this may in turn have a biological consequence.
Table 10.2 Causes of hypogonadism in the male
Primary hypogonadism (low testosterone, high LH, FSH) | |
Klinefelter’s syndrome | Testicular torsion |
Androgen resistance | Trauma |
Androgen synthesis defects | Iron overload |
Anorchia or cryptoorchidism | Drugs including alcohol |
Epididimo–orchitis (e.g. mumps) | |
Secondary hypogonadism (low testosterone, LH, FSH low or normal) | |
Kallman’s syndrome | Opiates, cocaine, anabolic steroids |
Hypothalamic–pituitary disease | Excessive exercise |
Isolated GnRH deficiency | Stress |
Panhypopituitarism | Weight loss |
Destructive pituitary tumour | Moderate illness (acute or chronic) |
Cushing’s syndrome | Iron overload |
Hyperprolactinaemia | Oestrogen-secreting tumours |
Investigation of infertility and male hypogonadism
Endocrine causes of subfertility are rare in men. Most infertile males are eugonadal, with oligospermia due to failure of the seminiferous tubules. In a eugonadal male with a normal sperm count, endocrine investigations are not required. Causes of male hypogonadism are given in Table 10.2.
If on two occasions the sperm count is less than 20 × 106/ml and/or motility is poor in more than 50% of the sperm, measurements of serum [LH], [FSH] and [testosterone] should be made to determine whether hypogonadism is caused by a primary defect in the testes or in the hypothalamic–pituitary region. Both forms lead to infertility (Figure 10.2). Azoospermia with a raised FSH suggests severe seminiferous tubular damage, while azoospermia with normal FSH and normal testicular volume indicates bilateral genital tract obstruction. Plasma [prolactin] should also be determined (p. 108), as hyperprolactinaemia can lead to diminished libido, hypogonadism and impotence.
Misleadingly high values for LH and FSH might be observed because of pulsatile release. Serum [total testosterone] results are affected by changes in serum [SHBG]. ‘The calculated serum [free testosterone]’ should be derived for all males who have abnormalities in serum [SHBG] or a low total testosterone. The calculation of the FAI provides an unreliable and often misleading estimate of free testosterone in males and its use should be avoided in male patients.
Hypergonadotrophic and hypogonadotrophic hypogonadism
Causes of hypogonadism are listed in Table 10.2
Primary gonadal failure: hypergonadotrophic hypogonadism
The primary abnormality is in the testes, and serum [testosterone] is reduced while gonadotro phins are increased. This group of conditions includes congenital defects such as Klinefelter syndrome (usually 47XXY) and acquired lesions due to drugs, viruses or systemic diseases that affect testicular function. In some cases of abnormal spermatogenesis, FSH may be raised but LH and testosterone may remain normal.
Figure 10.2 The investigation of male infertility. *Prolactin should also be measured to exclude hyperprolactinaemia.
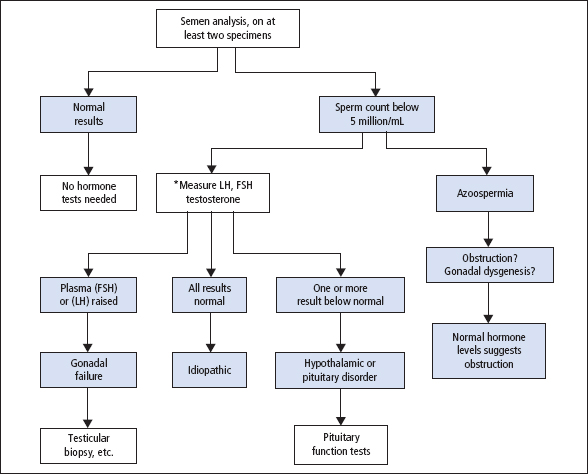
Hypothalamic–pituitary disease: hypogonadotrophic hypogonadism
The primary abnormality is in the hypothalamus or the pituitary; the deficiency may be part of a generalised failure of pituitary hormone production. Serum gonadotrophins and [testosterone] are often both reduced, but in some cases gonadotrophins may remain within the reference range. Low testosterone is a common finding in those who have moderate to severe chronic or acute illnesses.
hCG, injected daily for several days, can be used to help differentiate between hypergonadotrophic (primary) and hypogonadotrophic (secondary) hypogonadism. A failure to show a rise in serum [testosterone] suggests inadequate Leydig cell function whilst an exaggerated response suggests secondary hypogonadism.
Disorders of male sex differentiation
Many conditions have been described, all rare. In some, the gonads degenerate; in others, there is an enzyme defect affecting steroid synthesis. In a third group, there is androgen resistance at the end organ, and a fourth group consists of the true hermaphrodites.
The testicular feminisation syndrome is inherited as an X-linked recessive, caused by a mutation in a gene coding for the androgen (testosterone) receptor, in which genetic (XY) males develop female secondary sexual characters; serum [testosterone] is abnormally high. In many of the other conditions, plasma [testosterone] is low, both in childhood and in adult life.
Erectile dysfunction (impotence)
This is most commonly caused by diabetes, neurological disorders, cardiovascular disease medication such as β-blockers, alcohol abuse, thyroid disease, liver and renal disease and psychological problems. Testosterone deficiency and hyperprolactinaemia are uncommon causes of erectile dysfunction; such patients often complain of loss of libido. Patients with this condition should have glucose, lipids, thyroid function tests and renal function assessed in addition to testosterone and prolactin.
A common clinical problem is the patient with erectile dysfunction who has a slightly low testosterone and calculated free testosterone yet a ‘normal’ LH and FSH. A repeat blood sample should be taken between 8.30 and 11 am (since testosterone falls during the day) and if a low testosterone is confirmed an assessment of basal pituitary function should be carried out (Chapter 7). If no evidence of pituitary dysfunction is found some endocrinologists may still advocate a trial of testosterone replacement therapy; others argue that a fall in testosterone represents the normal pattern of ageing and would not give such therapy.
Andropause
In men, total and free testosterone concentrations tend to decline from about the age of 40. Whether this decline in testosterone is responsible for some of the functional changes that occur with age such as decreased muscle strength and decline in libido remains controversial. The long-term benefits and potential risks (e.g. prostate cancer) of testosterone treatment in older men are at present still being debated.
Gynaecomastia in males
Breast development occurring in males other than in the neonate or during puberty usually has a pathological cause. About 25% of cases of gynaecomastia are idiopathic but the principal endocrine causes are conditions that lead to an imbalance of oestrogen and androgens (Table 10.3). These include decreased androgen activity in hypogonadism and increased oestrogen production resulting from a variety of endocrine tumours; these tumours may synthesise oestrogens or secrete hCG, which then acts as a stimulus of oestrogen production. Thyrotoxicosis, hyperprolactinaemia, renal failure, liver failure and androgen resistance are other pathological causes that lead to an imbalance of oestrogens and androgens. Drugs account for about 20% of cases of gynaecomastia, and over 300 drugs have been reported as having the potential for producing the condition. Most drugs cause gynaecomastia by modifying the androgen/oestrogen ratio by direct or indirect mechanisms. In the elderly, mild gynaecomastia may commonly occur as a result of a decline in testosterone production.
Table 10.3 Some causes of gynaecomastia
Hypogonadism (primary or secondary) | Haemachromotosis |
Drugs – including cannabis, methadone, cimetadine, phenothiazines, oestrogens | Tumours – including testicular, adrenal, bronchus, liver, haematological, etc. |
Thyrotoxicosis | Chronic renal failure |
Cushing’s syndrome | Liver disease |
Congenital adrenal hyperplasia | Hyperprolactinaemia |
Over 300 drugs have been reported to cause gynaecomastia, and in many, the mechanism is uncertain.
Patients require full endocrine investigation, including measurement of serum oestrogens, androgens, gonadotrophins, prolactin, hCG and SHBG. Tests of liver, renal and thyroid function should also be performed and a full drug history taken.
Female gonadal function
Menstrual disorders and infertility
The changes that occur in normal menstrual cycles depend on cyclical variations in the output of FSH and LH, influenced by the output of GnRH (Figure 10.3). The effects of GnRH on LH and FSH release, in terms of the amounts secreted at different stages of the menstrual cycle, are strongly influenced by negative feedback control effects exerted by oestradiol-17β and progesterone.
The developing Graafian follicles in the ovaries respond to the cyclical stimulus of gonadotrophins by secreting two oestrogens, oestradiol and oestrone; these are metabolised to a third oestrogen, oestriol. After ovulation, the corpus luteum secretes progesterone as well as oestrogens. The changes in the uterus are determined by the ovarian steroid output at each stage. These changes are modified if pregnancy occurs.
Figure 10.3 The hypothalamicpituitary-ovarian axis. Activins, inhibins and progesterone also have a role in regulating the cycle.
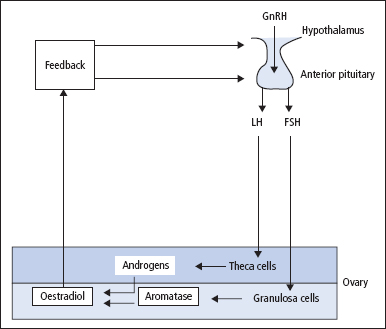
Figure 10.4 Cyclical changes in the plasma concentrations of the pituitary gonadotrophins and the principal ovarian steroid sex hormones in a normal 28-day menstrual cycle.
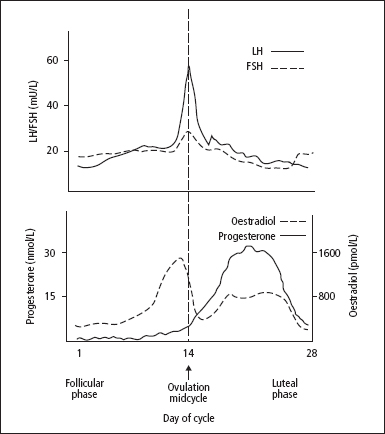
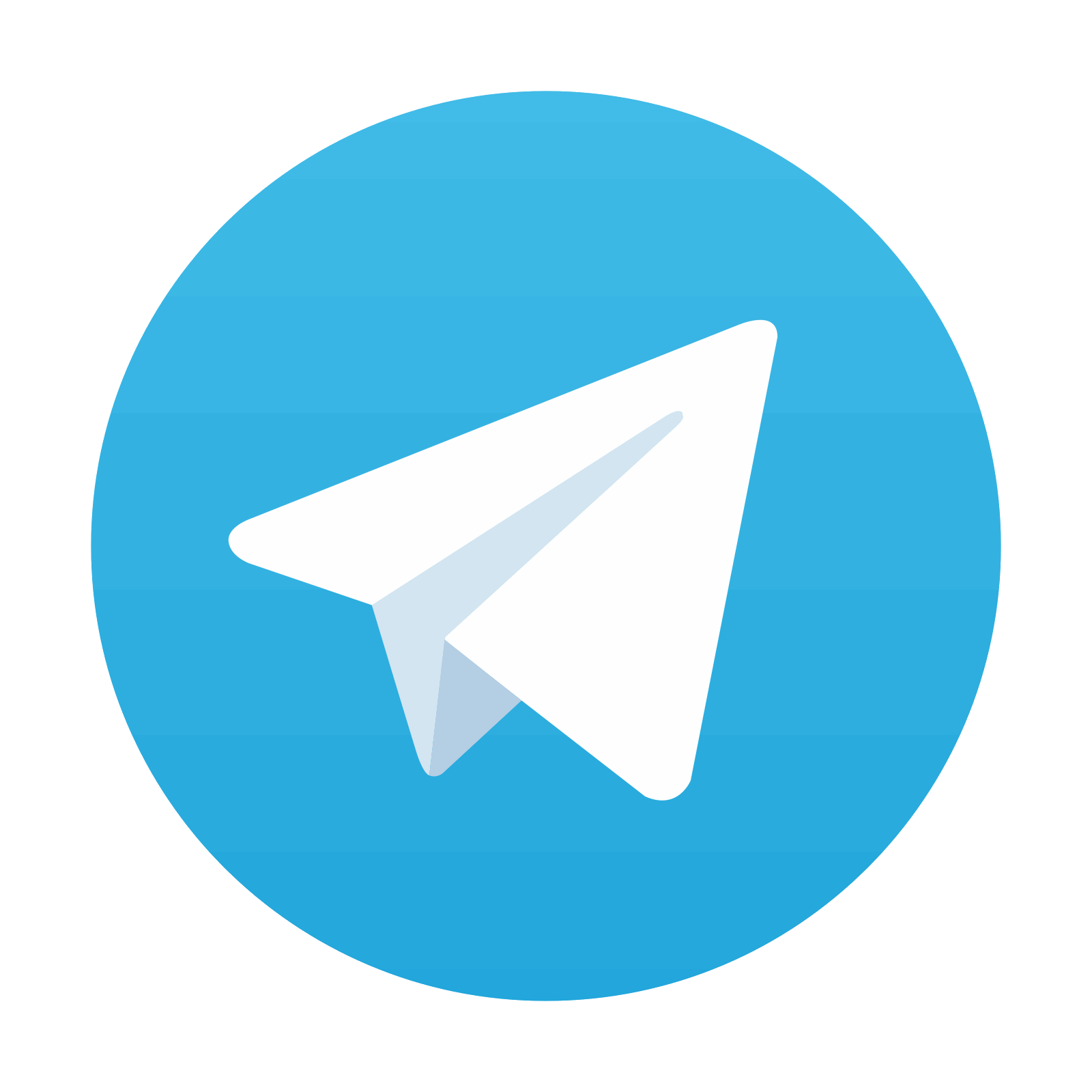
Stay updated, free articles. Join our Telegram channel

Full access? Get Clinical Tree
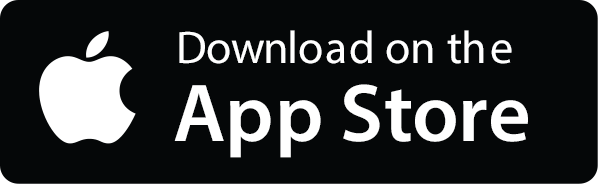
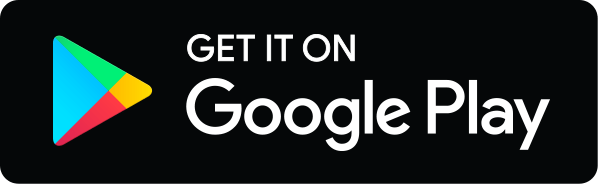