This chapter should be regarded as a warm-up. It will answer three questions about high-performance thin-layer chromatography (HPTLC): After a brief discussion of typical problems and questions frequently encountered by the herbal industry, we will learn why separation techniques are important and how thin-layer chromatography (TLC) stands out in this respect. If you already have experience with chromatography, you might skip the section “What Is Chromatography?” High-performance liquid chromatography (HPLC) and gas chromatography (GC) enthusiasts who have not done TLC in a while might find the section “What Is TLC?” particularly enlightening. All other readers are invited to enjoy the whole chapter. ♦ Analysis of Herbal Drugs What Are the Challenges? Dietary supplements, botanicals, nutraceuticals, herbal drugs, herbal medicinal products (HMP), natural health products (NHP), traditional Chinese medicine (TCM), and Ayurvedic drugs…it is difficult today to stay abreast of all those categories. Regulatory issues, safety concerns, popularity, economic value, and so forth, are complex questions affecting a huge market. New products are introduced daily, others are being banned, competition is fierce, and the pressure to keep costs low and maximize profit is always present. Marketing claims made in ads or on the label are often diffuse or cryptic, meaningless or too technical. A central question is common to all those products on the market: How is quality defined and ensured? Although that question seems rather simple, an answer is not easily found. Let us first consider pharmaceutical drugs: What does that mean for the analytical chemist? First of all, the analytical tools must be not only highly accurate and precise but also very sensitive. They should also allow separating the target compound from any impurity possibly present. Isolated compounds need to be identified and quantified. The exact quantity of the active ingredient in the finished dosage form must be certified. Numbers and acceptance criteria are used to carefully control all aspects of product quality (i.e., safety). When we now look into botanical drugs, the situation is quite different: Talking about quality is now a much more diffuse issue. When we limit our view on plant-derived products and leave out mineral and animal-related products, quality begins with the right species. Next, a traceable connection must be established between the raw material, a defined manufacturing process, and the quality of the finished product. It must be determined what and how much of it becomes part of the product. This is what the FDA regulation concerning current good manufacturing practices (CGMP) for the botanical industry is trying to deal with.1 Unfortunately, this solves the problem of quality only in theory. These are some of the reasons for it: In practice, new approaches have to be considered, which can address the complexity of the problem more appropriately. Instead of “zooming in,” which is necessary for impurity and trace analysis, the larger picture should also be seen. What Are We Dealing With? On one side there is the botanical raw material and on the other a more or less processed product. In between are all sorts of intermediates, and throughout is uncertainty regarding safety, efficacy, and chemical quality. Although it would seem at first glance that things should be largely under control as far as the plant material is concerned, one might expect the quality of finished products to be more difficult to control. When we approach the issue with the “pharmaceutical” quality in mind, everything changes: All this creates great challenges for the analyst, but quality control and quality assurance for botanicals are not impossible. It now a widely accepted fact that “happy and satisfied customers” alone are no longer a strong enough measure of quality. Chromatographic separation of suitable constituents for identification, in addition to their quantitative measurements, where necessary, should be performed. With that in mind, the following are some typical tasks for the botanical industry. Typical Analytical Tasks Identification of Raw Material and Products During chemical identification of a botanical sample, a so-called chromatographic fingerprint can be generated. The sample is extracted and then chromatographed. The result is a specific sequence of peaks or zones due to known or unknown components of the extract. The fingerprint of botanically authenticated raw material serves as a primary reference against which unknown material can be characterized. Identification can also be performed against chemical reference standards if the sample is expected to contain those. “Good” quality of a sample in the identification test means that the Box 1–1 Visual Comparison of desired plant species is present. Box 1–1 Visual Comparison of HPTLC Fingerprints for Identification Visual evaluation of a TLC plate (or an image of it) allows convenient comparison of many samples side by side. Similarities and differences can be clearly seen. Let us look at a few details. We want to learn how to “read” such a chromatogram. Tracks are generally compared one with another with regard to the number, relative position, color, and intensity of zones. A “good” chromatogram has zones that are horizontal and not distorted. Start and front lines are parallel. At the first glance, all samples in Fig 1–1 are very similar and represent the same species. The fingerprints of the raw materials (tracks 1 to 3), extracts (tracks 4 to 7), and finished products (tracks 9 to 14) are comparable but not identical. For example, all samples show a broad zone at the position of rutin (track 8) and two green zones just above. Closer inspection reveals that the “rutin zone” represents two overlapping zones. At low concentration (as in track 11), separation of the two zones is better seen. The middle portion of all tracks is similar, but tracks 3 and 14 show a strong white zone. This zone overlaps with a yellow zone on tracks 4, 6, 9 to 11, and 13. A red zone (chlorophyll) is seen close to the solvent front on tracks 1 to 3 and 9. The higher the resolution and specificity of the chromatogram, the clearer small differences between samples will become. It is highly unlikely that two plants and consequently two finished products are exactly alike. Therefore, an analytical method must be chosen that allows identification with certainty but tolerates minor variations. Identification can be considered one of the dominating applications of HPTLC. One of the most appealing features of HPTLC fingerprints is the visual impression Fig 1–1. A broad spectrum of constituents can be detected and described without the need to know the chemical nature of each chromatogram zone. Detection of Adulteration One of the common problems in quality control of botanicals is the purposeful exchange or accidental mix-up of plant species. It affects quality in any case, but adulteration/falsification becomes critical if the wrong species is toxic. A popular case is Stephania, which is easily confused with various aristolochic acid—containing plant species such as Aristolochia fangji Fig 1–2. The requirements for a suitable method are specificity (being able to distinguish right from wrong species) and sufficient sensitivity for detecting small amounts of adulterant. HPTLC is well suited for these tasks, correlating good quality with the 4 absence of adulterants. Figure 1–1 HPTLC fingerprint of various Ginkgo preparations.* Mobile phase (MP): ethyl acetate, acetic acid, formic acid, water (100:11:11:26); derivatization (D): NP reagent, UV366 nm; tracks 1 and 2, ginkgo leaf; track 3, ginkgo leaf, freeze dried; tracks 4 to 7, ginkgo leaf extract, powder; track 8, rutin; tracks 9 and 10, ginkgo leaf extract, capsule; tracks 11 to 13, ginkgo leaf extract, tablet; track 14, ginkgo tincture. See Box 1–1 for discussion. *Note: Unless otherwise stated, all images in the book represent HPTLC plates Si 60 F254 (Merck, Darmstadt, Germany). All plates have been cropped below the application position and above the front. Only the tracks of interest are shown. For a list of abbreviations, see page 215. Stability Tests Stability tests on herbal drug preparations and finished products are a rather new application of HPTLC. During such tests, it has to be shown that a material does not change over the declared shelf life when stored properly. On the other hand, if any changes occur, they should be detectable. In this test, good quality is indicated by no change over time. Figure 1–2 Detection of aristolochic acids as indication for adulteration. Mobile phase (MP), upper phase of toluene, ethyl acetate, water, formic acid (20:10:1:1); derivatization (D), tin (II) chloride reagent, UV 366 nm; 1 and 2, Aristolochia fangji, 1 and 10μ L; 3 and 4, aristolochic acids mix (Aldrich, St. Louis, MO), 10 and 50 ng; 5 and 6, Stephania tetrandra adulterated with 10% and 1% Aristolochia fangji, 10 μL; 7, pure Stephania tetrandra, 10μ L. See Box 1–2 for discussion. Box 1–2 Visual Comparison of HPTLC Fingerprints for Detection of Adulteration For detection of adulteration, typically one or more zones (markers) of known or unknown identity are selected. These markers must be specific for the adulterant, which means present in the adulterant and absent in the unadulterated sample. To increase the analytical certainty, a chemical reference substance is used for comparison. In the example presented in Fig 1–2, the reference is a mixture of five aristolochic acids, which can be identified as the main components and markers of Aristolochia fangji (tracks 1 and 2). Tracks 3 and 4 represent two different amounts of the reference. The fingerprint of Stephania tetrandra (track 7) is “empty” in this chromatographic system. This makes the markers selective for Aristolochia. If Stephania raw material is mixed with Aristolochia raw material (e.g., 10% or 1%), this adulteration can be clearly detected. The markers are present in the finger prints on tracks 5 and 6. Box 1–3 Densitometric Evaluation of HPTLC Chromatograms With the help of a densitometer, the HPTLC chromatogram can be converted into an analog curve. Detected chromatogram zones are represented as peaks, which can be integrated. For identification, peak positions expressed as migration distance or RF-value are compared. For quantitation, peak areas or heights are evaluated. For a direct comparison of all integrated tracks, the three-dimensional (3D) plot can be viewed like a mirror image of the plate. The application position is on the left side, the first track is in front, and the last track is in the back of the plot. In the given example, the first and last five tracks represent 10 increasing levels of tetrandrine standard. The signals measured for standards (pink X) are fitted with a calibration function against which the signals for the tetrandrine peaks (blue +) found in the samples are evaluated. Quantitation of Marker Compounds The most commonly used measure of quality is related to the amount of certain marker compounds in a sample, even though “correct” quantities of the markers must not be confused with overall quality. Sufficient separation power of the chromatographic method to achieve baseline resolution for all components is usually desired. In many cases, quantitation is based on HPLC separation. It is often overlooked that with proper instrumentation and suitable methodology, quantitative determinations by HPTLC could give equal results at lower cost Fig 1–3. In Chapter 4, we will discuss these applications in greater detail. Now we want to look at chromatography as a general method. ♦ What Is Chromatography? One of the “scientific” definitions describes chromatography as “a separation technique based on the different affinities of the components of a mixture to two immiscible phases. One phase is stationary, the other mobile.” Unfortunately, this definition doesn’t help much unless you really have a good background in physical chemistry, know about all sorts of equilibriums, and enjoy mathematical equations explaining why certain processes take place and others do not. If you want to brush up your knowledge in this regard, check out Refs. 2–4. Otherwise, let’s start with the model given in Fig 1–4. A Simple Model of Chromatography We want to separate a large group of people into subgroups of similar individuals and have invited volunteers to participate in an outdoor experiment. When looking closely at the participants, we notice that they seem to be very diverse and difficult to group as far as their interests are concerned. However, we can think about something basic that almost everybody would be attracted to: free food and drinks. The rules of the experiment are easy: Figure 1–3 Quantitative determination of tetrandrine in Stephania tetrandra. (A) 3D plot of analog curves of samples (tracks 6 to 8) and standards (all other tracks). (B) Calibration function for tetrandrine. See Box 1–3 for discussion. Figure 1–4 “Chromatography.” Here is what may happen: Most of the people will accept the invitation and have a good time eating. Some had enough for breakfast and don’t want to stop at all. They will arrive at the finish line shortly after the start of the game. Others may not be very hungry and soon realize that most vendors offer the same food. After a quick stop they continue, and it does not take very long for them to reach the goal. The hungry participants of course will feast, but finally most of them can’t eat any more, and so they arrive at the finish line. Of course, there are always a few people not paying attention, like the diver in the lower left corner of Fig 1–4.They just remain at the start line. While everybody is having fun, the referee is creating a chart that looks like Fig 1–5. Shortly after the start (t0), a few boats will arrive. Their captains either forgot to stop or didn’t care. The time it took them (tM) is dependent on the velocity of the river. The boats that arrive later will have stopped at least briefly, and the longer their captains ate and drank, the more time it took them (tR1,tR2, etc.) to finish. Certainly, the individual boats of each group are not coming in at exactly the same time. Just think about what happens if 50 hungry people enter the typical hamburger joint at one time; there will be some haggling. But also on the river there is some trouble: too many boats are trying to get going simultaneously. Some are pushed to the banks; others are getting caught at the docks without wanting to stop. We can use the described event to explain chromatography in general. Only a few changes must be made and some new terms adopted. Chromatography: A More Scientific Approach The whole set described in the previous section is called the chromatographic system. It could be for instance an HPLC column. The river is the mobile phase. It has a constant flow rate maintained by a pump. The mobile phase requires a certain time to move from one end of the system to the other. This is tM, the mobile time. The clock starts when the sample is injected into the system. The docks can be viewed as the stationary phase to which the components of the sample have a certain affinity. There is also an affinity to the mobile phase. While in the mobile phase, the sample component is migrating. If a component interacts with the stationary phase, it is retained relative to those components, which are not interacting and arrive at tM. The time differences are called net retention times (tRʹ). All molecules of a given sample component have the same affinity to the stationary phase and will be equally retained. With a suitable detector, the components arriving at the outlet of the column can be measured and recorded. The result is a chromatographic peak. Relative to the mobile time, each peak in the chromatogram can be assigned a capacity factor kʹ. This value is characteristic for a compound in a given chromatographic system and can be used for identification. More details on retention data are presented in Chapter 2. Unfortunately, such data are difficult to predict in general practice. That’s why qualitative chromatographic analysis is based on comparison to reference standards. If two compared samples are identical, they must have the same kʹ value, but the reverse relation is not true. That means even though two compounds are different, they may still have the same capacity factor. In this case, the chromatographic system is not selective. The trick is selecting an appropriate combination of stationary and mobile phase to achieve separation of a mixture. To increase certainty that two substances are really identical, they are usually chromatographed in two or more chromatographic systems of different selectivity in which they must also have the same retention behavior. The other option is to subject the separated compound either online or off-line to other analytical methods such as ultraviolet (UV), infrared (IR), mass spectrometry (MS), or nuclear magnetic resonance (NMR) for so-called positive identification. Figure 1–5 Retention in column chromatography (schematic): t0, start of chromatogram (injection); tM, mobile time (time for a nonretained molecule to pass the column); tR, retention time (time it takes a retained molecule to pass the column); tRʹ, net retention time (retention time less mobile time) tRʹ = tR–tM; kʹ, capacity factor (a measure of retention independent of flow rate and column dimension) Kʹ=tR–tM/tM. Classification of Chromatography The mobile phase of a chromatographic system can be a gas, a liquid, or a super-critical fluid. The stationary phase is either a solid or a liquid. These options allow convenient classification of the chromatographic process as listed in Table 1–1.
1
Introduction
Mobile phase | Stationary phase | Abbreviation |
---|---|---|
Gas | Solid | GSC |
Gas | Liquid | GLC, GC |
Liquid | Solid | LSC, LC |
Liquid | Liquid | LLC |
Supercritical fluid | Liquid (solid) | SFC |
For separation in GC, the sample must either be gaseous or at higher temperatures volatile enough to be evaporated. The only requirement for liquid chromatography (LC) is that the sample is at least partially soluble in the mobile phase.
Some more specific details about the chromatographic principle will be given in Chapter 2. At the moment, the question about the “affinities” of sample components to the stationary phase and to the mobile phase shall be briefly addressed. The underlying interactions (separation principles) at the molecular level can be used to classify chromatography into several types (Table 1–2). The chromatographic system must be selected depending on the chemical nature of the components of a mixture.
So far, we have only discussed chromatography in a column, which is packed with small particles of the stationary phase. But there are other technical solutions, as seen in Table 1–3.
Name | Typical interaction |
---|---|
Adsorption chromatography | Specific interaction of functional groups of solid surfaces with functionality of sample molecules based on H-bond, acid—base, dipole—dipole, dipoleinduced dipole interactions, dispersion forces, etc. |
Partition chromatography | Solubility of sample in stationary phase based on lipophilic character (chain length, branches, structure) or solvation including specific interaction (such as H-bonds) |
Ion-pair chromatography | Either dynamic ion exchangers are formed by incorporating counter ions into reversed phase or partitioning of neutral ion pairs is seen |
Ion chromatography | Electrostatic interaction of ionic sites on stationary phase with ionic samples |
Hydrophobic interaction chromatography | Altering the tertiary (quaternary) structure of large biomolecules affects their solubility and causes hydrophobic interaction with short carbon chains of stationary phase |
Affinity chromatography | Selective interaction between target and selector molecules (key—lock principle) traps specific target molecules |
Size-exclusion chromatography | No interaction! Reverse sieve effect: small molecules penetrate pores of stationary phase and are eluted later than large molecules, which are excluded from entering pores. SEC takes place between t0 and tM. |
♦ What Is TLC?
General Concepts in Comparison with Column Chromatography
In planar chromatography, a somewhat different approach is taken. Coming back to our experiment on the river, we make a change. A certain time after the start of the event, the referee makes up his mind and calls everything off. Each participant has to remain at his or her current position, and a map is drawn to represent the actual situation. “Nonretained” participants will mark a “front” line, and others will be distributed at staggered positions along the way. Figure 1–6 illustrates the result schematically.
In planar chromatography, the stationary phase is a flat (thin) layer coated onto an inert support (i.e., glass, aluminum, or polyester). That’s where the term thin-layer chromatography comes from. There are of course many similarities between TLC and HPLC, because both are forms of LC. In general, the same stationary and mobile phases can be used with both techniques. The same kinds of samples are dealt with, and the performance is comparable. Today, both chromatographic methods offer mature high-performance systems at several levels of sophistication.
A difference between the methods is the way of expressing retention. In column chromatography, the components of a sample require individual times to pass the column. In other words, separation is based on variable retention times and fixed distance. In planar chromatography, the sample components are allowed to migrate for a certain time. This way, separation is based on variable migration distance and fixed time. Instead of the capacity factor kʹ of column chromatography, planar chromatography uses the RF value to express relative retention.
Figure 1–6 Planar chromatogram (schematic): A, B, and C, various substances; MD, migration distance; zfʹ, distance of application position to solvent front; RF=MD/zfʹ
Another difference is associated with the mobile phase flow. In column chromatography, the mobile phase is maintained at a certain optimal velocity by means of gas pressure (GC) or pump action (HPLC). In TLC, capillary forces drive the mobile phase. In addition to stationary and mobile phases, TLC also takes advantage of a gas phase, which is usually present.
Figure 1–7 Setup of an HPLC system (schematic): 1, solvent reservoir/degasser (four solvents); 2, pump; 3a, injector; 3b, autosampler; 4, column; 5, detector; 6, waste.
The Practical Solution: Online versus Off-line Principle
Column chromatography (we will consider only HPLC in the following) operates in online mode. Figure 1–7 gives a schematic overview including only the key elements. A pump continuously feeds the mobile phase from a reservoir through the column and the detector to waste. Capillary tubing makes all connections. With the help of an injector (sample loop), the sample is injected either manually or by an autosampler into the flow, reaches the column, and is separated. The individual components eluting from the column are measured in the detector and then put into waste. Other devices such as gradient mixers, column oven, and fraction collector (for preparative work) can also be connected online. For detection, UV, refractive index (RI), evaporative light scattering (ELSD), diode-array, fluorescence, electrochemical, or mass spectra detectors are available. The control of the entire system and handling of the data are typically computer based.
A key feature of the online principle is that multiple samples are analyzed in sequence. That means when the last component of an injection has left the chromatographic system, it must be reset before the next sample is injected. Operation can only be interrupted between samples, not during a run. While in the system, the sample is completely protected from environmental factors.
The sequence for a typical quantitative analysis consists of several calibration standards (if necessary in duplicate), a blank run, and any number of unknowns. If many samples are analyzed over a long period of time, control standards are included at certain intervals. As long as no changes are made to the system and the results for the control standard and the blank are within specification, the samples can be analyzed against the same calibration curve. Online operation is well suited for full automation.
Thin-layer chromatography uses the off-line principle (Fig 1–8). The process is separated into individual steps. First, the sample is applied onto the plate. The plate is then developed, if necessary derivatized, documented, and evaluated. The main difference to the online principle is that all steps are separated in time and location. Instruments (sample applicator, developing chamber, immersion device, documentation system, densitometer) are available for automation of individual steps. Even software controlling/managing the entire process can be employed. However, the plate as the central piece of TLC has to be moved manually through all steps. This may be seen as inferior to the fully automated HPLC setup, but as we will see, it adds tremendous flexibility to the TLC operation, which is unsurpassed by any other method.
Figure 1–8 The off-line principle in TLC.
Advantages of TLC
In comparison with column chromatography, TLC offers several advantages:
- Visual results
- Simplicity
- Parallel analysis of samples
- Rapid results
- Flexibility
- Single use of plate
- Multiple detection
- Cost efficiency
Let us discuss these issues in more detail with applications in mind that are important for the analysis of botanicals. It depends of course on the point of view, but some of the presented features have consequences or aspects that could be seen as disadvantages as well.
At this point, we want to make it clear that discussing the advantages of TLC does not negate the strengths of other techniques. However, we would like to see that TLC is used as a rapid method capable of providing reliable information. TLC should be the first choice when it is the more suitable solution for a given analytical problem.
Visual Results
TLC is the only chromatographic method offering the option of presenting the result as an image. A chromatogram can be seen as a sequence of dark, colored, or fluorescing zones on the plate, and it can be easily documented as a picture (Fig 1–9A).
An HPTLC chromatogram may lack the great sharpness of an HPLC separation, which is due to the higher efficiency of HPLC, but information can usually be communicated much better visually than in the form of verbal description or long peak tables. Just imagine how long it would take you to describe to someone else what you see in Fig 1–9B. How would you compare the eight samples on the plate in the form of analog curves or—to get the color information as well-in the form of 3D plots from a diode-array detector?
Figure 1–9 HPTLC fingerprints of feverfew and adulterants. Mobile phase (MP), cyclohexane, ethyl acetate (1:1). (A) UV 254 nm. (B) Derivatization (D), anisaldehyde reagent, white light; track 1, parthenolide; tracks 2 to 5, feverfew; track 6, Mexican feverfew; track 7, chamomile; track 8, Roman chamomile. See Box 1–4 for discussion.
Box 1–4 Visual Evaluation of HPTLC Chromatograms: What Is Important?
Let us investigate the question about the identity of several feverfew samples. This plant is easily confused or adulterated with other species such as Mexican feverfew, common chamomile, or Roman chamomile. The HPTLC fingerprints of all samples are quite similar, and it requires an optimized chromatographic separation to find differences. The images in Fig 1–9 show the same plate in two detection modes. Prior to derivatization under UV 254 nm, some separated substances quench the fluorescence of the indicator built into the plate. Dark zones are seen on green background. Although this detection mode does not provide much information, it can clearly distinguish chamomile (track 7) and Roman chamomile (track 8) from the other samples, which are rather similar. After derivatization, more details are revealed, and greater similarity of the feverfew samples is seen. The reference substance parthenolide (track 1) shows a blue-colored zone. (Note that under UV 254 nm, this zone was not visible.) The feverfew samples (tracks 2 to 5) feature similar but not identical fingerprints. The distinguishing element is the presence of a blue band at the position of parthenolide. None of the other species shows it. Chamomile has a characteristic yellow zone in the upper third of the chromatogram, and
Roman chamomile has a distinguishing prominent violet zone. Mexican feverfew has two dark-blue bands but both are below the zone of parthenolide. If feverfew is mixed with Mexican feverfew, the presence of these blue zones would be clearly detectable.
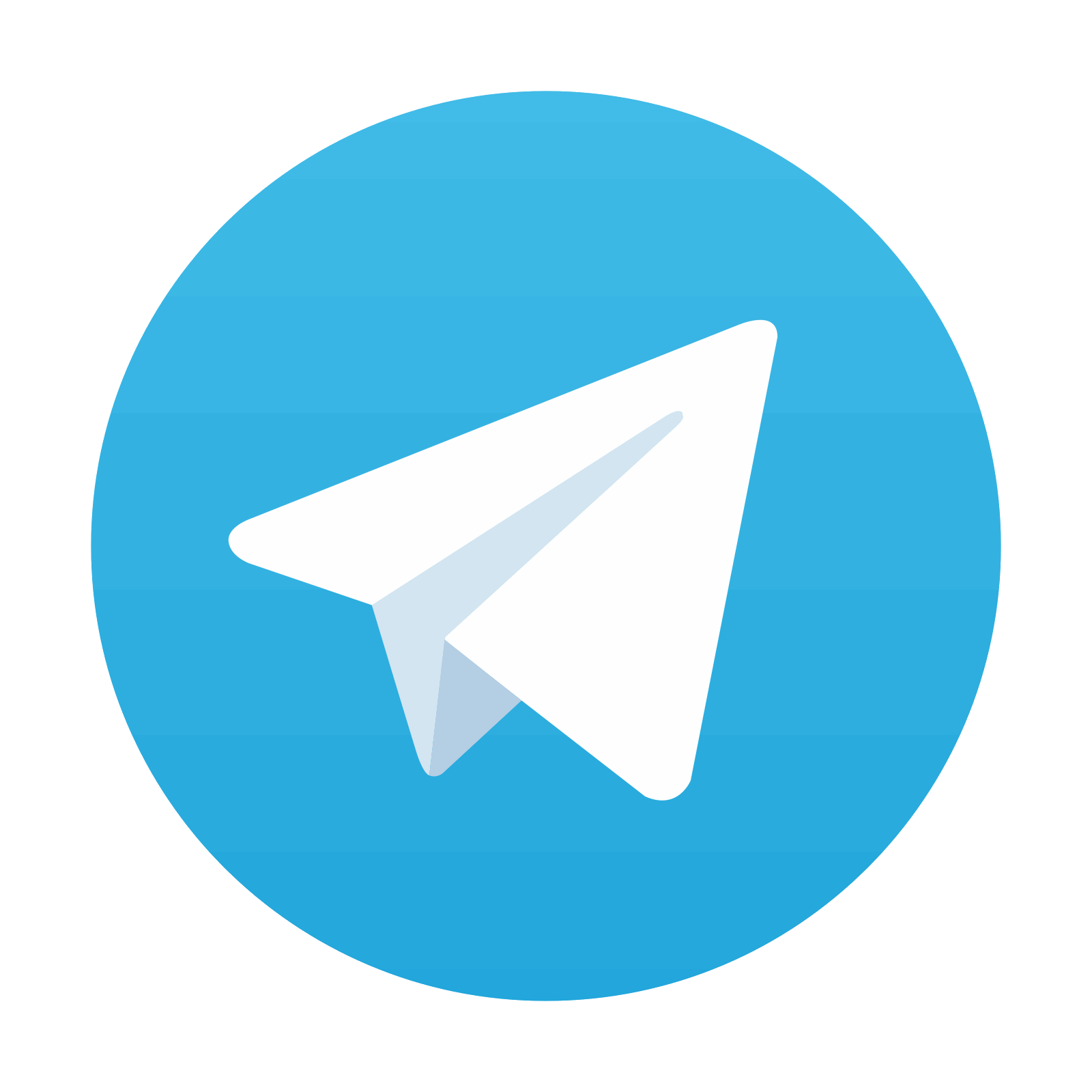
Stay updated, free articles. Join our Telegram channel

Full access? Get Clinical Tree
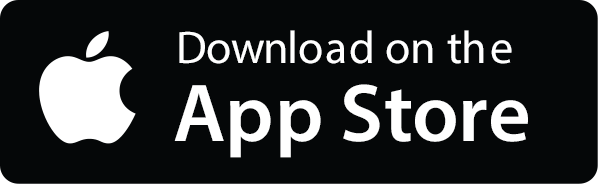
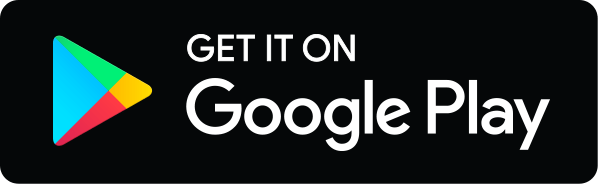