Jennifer Schaadt Image-guided procedures use medical imaging to plan, perform, and evaluate surgical procedures and therapeutic interventions. Interventional techniques began in 1953 when Dr. Sven-Ivar Seldinger performed percutaneous vascular access using a hollow-core needle guidewire and catheter (Mauro, 2009). This development led to the specialties of interventional radiology (IR) and interventional cardiology and is the catalyst for today’s minimally invasive procedures. IR is the medical subspecialty of radiology using minimally invasive, imaging-guided procedures to diagnose and treat disease. The IR procedure scope includes 10 major service areas (Box 29-1), is therapeutic, and is considered a clinical discipline (Mauro, 2009). Interventional radiologists have expertise in diagnostic imaging, radiation safety, image-guided minimally invasive techniques, and patient evaluation and management associated with these procedures (Society of Interventional Radiology [SIR], 2010). This less invasive combination results in lower risks, less pain, and shorter recovery time compared with open surgery (SIR, 2010). Interventional radiologists complete a residency in diagnostic radiology, then a fellowship in vascular and interventional radiology. Further specialization includes interventional neuroradiology and pediatrics. Additionally, IR categorizes procedures into vascular or nonvascular categories. Although this chapter focuses on IR, vascular procedures are not exclusive to IR and vice versa. Depending on the institution, interventional radiologists, vascular surgeons, and interventional cardiologists also perform peripheral vascular work, endovascular aortic stenting, inferior vena cava (IVC) filter placement, arteriovenous (AV) fistulas, and other similar procedures. IR team members include nurses, physician extenders (e.g., nurse practitioners, physician assistants), radiology technologists (RTs), radiology assistants, and when required, anesthesia providers (American College of Radiology [ACR]. 2009). IR uses radiography, computed tomography (CT), ultrasound, magnetic resonance imaging (MRI), fluoroscopy, and nuclear medicine (NM). NM typically is a distinct hospital department and not discussed in this chapter. The most common imaging in the operating room (OR) is ultrasound and portable C-arm fluoroscopy. Ultrasound does not emit ionizing radiation, and is used for pelvis, prostate, testicle, abdomen, obstetrics, and pediatric studies. Other applications of ultrasound include transcranial Doppler, intravascular ultrasound (IVUS), and transesophageal echo (TEE). The traditional angiography system in the OR is a portable C-arm, used primarily as an adjunct to surgical procedures, producing limited imaging and quality views (Katzen et al, 2010). Relative to intraoperative CT and MRI, a few large academic centers have these in an OR. Potential applications are for trauma, neurosurgery (Research Highlight), and stroke treatment, but the cost and facility constraints limit its overall adoption. The traditional care path for imaging and surgery is diagnostic imaging, surgery, repeat imaging with discharge, or additional surgery. Advances in imaging and percutaneous techniques have changed this sequenced care path to an integrated single episode, theoretically meaning a shorter length of stay, less-invasive procedures, customized care at the point of diagnosis, quicker recovery time, and a single anesthesia encounter. This integrated care requires effective collaboration of multiple staff members and physicians on all levels and interdisciplinary cooperation surrounding the new technology. As the adaption matures and becomes conventional in more specialties, some procedure overlap occurs. Vascular medicine exemplifies this trend performing diagnostic, percutaneous interventions and open surgeries overlapping the traditional medical, cardiologic, radiologic, and surgical disciplines (Bashore et al, 2012). This paradigm continues relative to the new physician fellowships and specialties such as interventional nephrology, interventional pain, minimally invasive surgery-gastroenterology, image-guided therapy, advanced or interventional endoscopy, and endovascular neurosurgery. The specialty of radiology has its own vernacular, detailed here and in the text (Box 29-2). Terminology differences include the use of physician rather than surgeon or interventionalist because various specialists perform these procedures. IR uses the term procedure when referring to a case, rather than surgery. Many faculties use the term special procedures or specials lab versus IR. This terminology carries through in patient communication as well. Staff in the holding room area prepare and recover patients. Modality is a general term meaning imaging technology. Radiology does not use the term table; modalities have tables and table tops. The term scanner means either a CT scanner or an MRI scanner; likewise scanning refers to the process of image acquisition for a CT or MRI. Magnet is another term for MRI scanner. Fluoroscopy is an imaging technique displaying continuous X-ray imaging blood flow in vessels (angiography). A fluoroscope is the modality inherent to the IR suite or specials lab, as well as the cardiac catheterization lab, electrophysiology (EP) lab, neurointerventional labs, and hybrid ORs. A hybrid OR has a fixed angiography system (versus mobile C-arm) performing open surgical and catheter-based interventions (Figure 29-1). Hybrid ORs vary in equipment configuration; however, the universal element inherent to a hybrid OR is its advanced imaging and reconstruction capabilities. These applications enable physicians to optimize their approach percutaneously, distinguish organ pathology, navigate instrument placement, ensure accurate device implantation and function, and convert to an open procedure if warranted. The image processing is done by a skilled RT or physician. Some of the advanced imaging techniques used in a hybrid OR include digital subtraction angiography (DSA), roadmapping, and rotational angiography. DSA is a computer-assisted fluoroscopy technique subtracting out the images of bone and soft tissue to view the vessels. DSA is useful in the diagnosis and treatment of peripheral arterial and venous occlusions, carotid artery stenosis, pulmonary embolisms, acute limb ischemia, renal arterial stenosis, and cerebral aneurysms and arteriovenous malformations (AVMs). Roadmapping is the superimposition of a live fluoroscopic image on a previously stored digitally subtracted angiogram. Roadmapping facilitates catheter and wire placement in complex and small neuroendovascular procedures such as aneurysm coiling, advanced stroke interventions, AVMs, and carotid stenting. Rotational angiography uses the rotation of the C-arm around the patient to reconstruct three-dimensional, cross-sectional CT-like images from standard two-dimensional angiography. Uses for rotational angiography include visualization of endovascular stent placement, complex surgeries of the aortic arch, transcatheter aortic valve replacement (TAVR), atrial fibrillation ablations, embolization techniques for tumor treatment, punctures, and drainages. Additional procedures performed in a hybrid OR requiring basic fluoroscopy include arteriograms or venograms, angioplasty and stenting, thrombectomies, arrhythmia device implantation, high-risk percutaneous coronary intervention (PCI) for unprotected left main coronary artery disease, and other complex and high-risk morphology. Combination interventional and surgical procedures performed in a hybrid OR include hybrid revascularization cardiac bypass graft (CABG), and PCI in the same setting, and congenital, structural and heart valve interventions. The location of a hybrid OR depends on the facility design, physicians, and procedures. New construction tends to locate a hybrid OR near the cardiac catheterization lab, IR, OR, or vascular lab. Such adjacencies bring economies of scale because these services use the same support space, imaging equipment, anesthesia, recovery, and supplies, as well as shared expertise (Schaadt and Landau, 2013). Facilities retrofitting typically convert an existing OR or interventional or catheterization lab into a hybrid room. Planning considerations regarding hybrid OR placement in a facility include procedure diversity, physicians, building constraints, and technology. In addition to imaging are numerous adjunctive technologies requiring advanced and expert planning prior to installing a hybrid OR (Landau, 2011). Hybrid ORs are complex work environments. There are no specific regulations or practices regarding skill sets and staffing patterns for a hybrid OR. Team representatives typically have a blended skill set of IR, surgery, and radiology. Although team members vary, an underlying key success factor is the interdisciplinary collaboration required to work in the room and optimize patient care. The personnel profile should include an open-minded attitude toward innovation, excellent communication skills, and a willingness to change traditional processes. Process change is perhaps the biggest obstacle to overcome because the standard workflow and processes inherent to each specialty must merge. There may be significant changes regarding the procedure approach, equipment, and environment depending on the core skill sets of staff. Moreover, in some cases the procedures are evolving, and best practice or standard workflow has not yet been established. When the processes, roles, and responsibilities become defined and standardized the benefits of a hybrid OR can be realized. The role of the nurse in radiology varies according to the site, modality, and department organization and the level of expertise. The practice analysis of radiology nursing (Center for Nursing Education and Testing [CNET], 2010) defined nine broad areas of nursing practice within both diagnostic IR roles. 1. Access patient and plan care. 2. Administer, monitor and evaluate therapeutic interventions. 4. Provide a supportive environment. 5. Provide a safe environment. 6. Manage emergency situations. 7. Participate in quality assurance improvement activities. 8. Participate in facility interdisciplinary activities including clinical, legal, and ethical issues. While the nurses’ focus has always been on patient safety and advocacy, today’s radiology nurse expands this focus across the entire continuum of radiology services, beginning with scheduling through follow-up postdischarge. The nursing process is intertwined with carefully designed, interdisciplinary protocols ensuring patient safety in a regulatory compliant and cost-effective environment (Kandarpa and Machan, 2011). There is no specific academic educational preparation for radiology nursing. Typical qualifications are prior experience in critical care nursing, emergency nursing, the cardiac catheterization lab, conscious sedation, advanced cardiac life support (ACLS), and pediatric advanced life support (PALS) (ACR and Society of Interventional Radiology [SIR], 2010.) Nursing practice should be in accordance with the American Nurses Association (ANA) and the Association for Radiologic and Imaging Nursing (ARIN) standards, and other established nursing guidelines. The ANA recognizes ARIN as a specialty with its own certifying exam, using the credentials certified radiology nurse (CRN). As always, nurses must practice within their scope of professional practice as per state licensing, national associations, regulatory agencies, and institutional policies and procedures. There is a clear delineation between the physician practice of diagnostic radiology and IR, but not necessarily for nursing. Depending on the institution, physician expertise, and case load, nurses may rotate or float from diagnostic radiology to IR. In other institutions, diagnostic and IR registered nurses (RNs) are separate departments, with different reporting structure, physician oversight, and dedicated staff. Nurses working within radiology may also work in the holding area. Because IR is procedure based, it requires a higher standard of patient care than general radiology. The scope of IR nursing practice is diverse and includes patient education, preprocedure planning, circulating, scrubbing, administering conscious sedation, patient recovery, and postprocedure discharge planning. The procedures performed encompass many different organ systems, various pathologies, and disease processes and are performed in different environments using a variety of imaging technology. As with traditional surgery, patient safety, procedural setup, instrumentation, medications, conscious sedation-monitoring, patient assessment, and complication management are IR nursing responsibilities. In IR the concomitant use of various imaging modalities creates a different environment and new dimension of patient care. The field of IR incorporates the same imaging technology (modalities) inherent to diagnostic radiology (e.g., radiography, CT, ultrasound, MRI, and fluoroscopy). IR is therapeutic, requiring a higher standard of care than diagnostic radiology. In many instances the modality establishes nursing practice. Hence, a high level understanding of each imaging technology, contrast agents, and radiation physics is important for the perioperative nurse. Radiography is the most common study ordered in hospitals. The correct terminology for radiologic images is radiography, films or images, not x-ray. Historically radiography studies required film. Today most radiography systems are filmless, known as computed radiography (CR) or digital radiography (DR). Instead of film, a photosensitive layer on a flat detector absorbs the energy, and converts it into photons captured for interpretation as a pixel. A computer converts the digital data into an image. X-rays can alter cellular activity resulting in damage to deoxyribonucleic acid (DNA). For this reason it is imperative to consider the benefit-versus-risk ratio when ordering medical imaging studies using ionizing radiation (Erkonen and Smith, 2009). A CT scan enables the clinician to review anatomic cross sections (slices) and three-dimensional images of the body using a combination of x-rays, x-ray detectors, and a computer. X-ray beams pass through sections of the patient as the patient traverses through the scanner. The detectors rotate, collecting a large amount of data reconstructed via computer to generate an image. CT studies are fast and rule out or determine the extent of any type of acute trauma, bleed, or injury as well as ongoing progression or resolution of a disease or condition. CT imaging assists with the planning and administering of radiation cancer treatments, surgical planning, guiding biopsies, and other minimally invasive procedures. CT contrast agents are either barium, for GI studies, or an iodine-based contrast injection given intravenously. Fluoroscopy is real-time continuous x-ray. Ionizing radiation passes through the patient collected by an intensifier or flat detector and digitally recorded and transmitted into moving images on a screen. Cardiac catheterization labs, electrophysiology (EP) labs, IR, and vascular and hybrid ORs use fluoroscopy for diagnostic and therapeutic procedures to determine catheter and device placement using iodine-based contrast agents. MRI is a radiology technique using magnets, radio waves, and a computer to produce images of body structures. The MRI scanner is a tube surrounded by a giant circular magnet with the patient placed on a movable table inserted into the magnet. The magnet creates a strong magnetic field aligning the protons of hydrogen atoms, which are then subject to radio waves. This spins the various protons of the specific body part being imaged producing a faint signal detected and amplified by coils. A computer receives and processes these signals generating an image. A magnetic resonance angiogram (MRA) is a type of MRI scan using a magnetic field and pulses of radio wave energy to image blood vessels. MRI scan times range anywhere from 30 minutes to several hours depending on the number of studies, requirement for contrast, and area of the body imaged. It is excellent for soft tissue anomalies and musculoskeletal studies as well as the abdomen, pelvis, brain, and spine and in other diagnostic applications (Research Highlight). The generation of a magnetic field requires a closed space, resulting in noise. Claustrophobic patients may require sedation. Contraindications for MRI include patients with implanted metal devices (e.g., pacemakers, defibrillators, plates, screws, and aneurysm clips or any foreign body [shrapnel]). Although MRI does not use ionizing radiation, it is crucial for those working in the environment to adhere to the ferrous metal guidelines protecting the patient and staff from injury (Patient Safety). Ultrasound produces sectional images or slices in multiple planes much like CT and MRI. Ultrasound uses the interaction of high-frequency sound waves to form the image. As these sound waves strike different tissue densities, a transducer measures the sound waves and an image results by converting the echoes into electrical impulses. Ultrasound does not use ionizing radiation. Applications include obstetrics, vascular studies, and abdominal studies (kidney, liver, gallbladder, pancrease) and for visualization during fluid drainage or biopsy procedures. Color flow Doppler uses ultrasound technology to quantify the direction and velocity of blood flow in vascular and cardiac diagnostic and surgical applications. Radiation is present naturally in the environment and is manmade. The use of medical imaging began with the discovery of x-ray in 1895. An x-ray is a form of ionizing radiation present within the portion of the electromagnetic spectrum (Figure 29-2). The ions liberated may produce cell injury by damaging the DNA molecules through the ionizing effects on DNA or indirectly through the formation of free radicals. The categories of radiation effects are deterministic and stochastic effects (Holmes, 2011). A deterministic effect occurs when individuals receive greater than a threshold radiation dose, with the severity of the effect depending on the amount of radiation and tissue exposed (Table 29-1). Stochastic effects refer to the probability of suffering a disease due to the cumulative exposure of radiation over years, for example, radiation-induced cancers, such as leukemia. TABLE 29-1 Dose Threshold for Deterministic Effects Modified from International Commission on Radiation Protection: Recommendations of the International Commission on Radiological Protection (users edition), ICRP publication 103 (users edition), Ann. ICRP 37, 2007. The U.S. population’s total exposure to ionizing radiation has nearly doubled over the past two decades (National Council on Radiation Protection and Measurements [NCRP], 2009) as a result of increased exposure from CT, nuclear medicine, and interventional fluoroscopy. The NCRP (2009) estimates the increased incidence of radiation-induced skin injury cases will grow resulting in regulatory concerns regarding radiation exposure (Evidence for Practice). It is important for the perioperative nurse to understand the basics of radiation physics and radiation dose metrics to effectively communicate with colleagues and patients, as well as for documentation, monitoring, and quality assurance. Different units of measure describe radiation dose. The Health Physics Society Position Statement (2012) advocates the exclusive use of the international system of units (SI) for radiologic quantities, which are the gray (Gy), sievert (Sv) and millisievert (mSv). For conversion purposes: Dose refers to radiation to the tissues. A dosimeter measures the amount of x-rays or radiation absorbed in a given period. The terms used to describe dose are absorbed dose, equivalent dose, and effective dose (Holmes, 2011). The unit for absorbed dose is Gy and is a simplistic definition referring to the amount of energy deposited in a kilogram of matter. The Gy does not take into account the type of radiation or tissue damage. The equivalent dose unit is the Sv, and refers to the damage caused by the type of radiation on a specific tissue using a weighting factor. The effective dose (Sv) measures both the type of radiation and the biologic effect of radiation on all the exposed organs (Holmes, 2011). Radiation dose varies by modality, with CT and fluoroscopy higher than radiography. For example, the adult effective dose from a CT exam of the head is equivalent to the adult effective dose from roughly 100 chest x-rays. The adult effective dose from a CT exam of the abdomen is roughly equivalent to the adult effective dose from roughly 400 chest x-rays (Table 29-2). The portion of the x-ray passed through the patient is the primary source. However, x-rays scatter on contact when interacting with various materials, producing scatter radiation, known as secondary radiation. TABLE 29-2 Modified from U.S. Food and Drug Administration: Initiative to reduce unnecessary radiation exposure from medical imaging, available at www.fda.gov/Radiation-emittingProducts/RadiationSafety/RadiationDoseReduction/default.htm. Accessed March 5, 2012. Four metrics exist for radiation dose estimation in fluoroscopic procedures: peak skin dose, reference dose, dose area product [DAP]), and fluoroscopy time (Miller et al, 2012). Peak skin dose, measured in Gy, is the highest radiation dose at any portion of the patient’s skin during the procedure. This measurement includes radiation from the primary x-ray beam and scatter. The skin dose varies by procedure as well as other factors (Table 29-3). Reference dose measured in Gy is the approximation of the total radiation dose to the skin and does not incorporate scatter. All fluoroscopes sold in the United States must display the reference dose at the operator’s working position. The DAP is a measure for the entire amount of x-ray energy delivered to the patient by the beam and is expressed as Gy*cm2. The DAP uses a device in front of the x-ray tube to measure radiation entering the body (Figure 29-3). Fluoroscopy time is a surrogate measure for documenting approximate radiation dose. Fluoroscopy time measures the time the x-ray beam is on and does not incorporate information about dose rate or entrance ports. TABLE 29-3 Modified from Conference of Radiation Control Program Directors, Inc: Monitoring and tracking fluoroscopic dose, 2010, available at www.crcpd.org/Pubs/Handout-MonitoringAndTrackingFluoroDosePubE-10-8.pdf. Accessed July 3, 2012. For CT the best radiation dose descriptors are CT dose index volume (CTDIvol) and dose length product (DLP) (Halliburton et al, 2011). Current CT scan technology uses multiple slices and spiral rotations of the x-ray tube to acquire an image. CT dose index (CTDI) measures ionizing radiation exposure per slice of data acquisition. The CTDIvol represents the total absorbed dose in and outside the slices with a unit of milligray (mGy). The DLP factors in dose along the actual length of the body part being scanned; units are mGy*cm. As an example, the DLP for a CT scan of the head will be smaller than the DLP for a CT scan of the chest and abdomen because the surface area is smaller. Patient size is a key factor governing the dose of radiation. Obese patients, as well as those with larger chests, require higher radiation doses to adequately penetrate tissues to yield a quality image. Radiation exposure to staff occurs as a result of scatter (Figure 29-4). The amount of scatter radiation varies directly with patient size (i.e., the scatter produced from pediatric procedures is lower than adult procedures). Other determinates of scatter are the duration of radiation exposure, the field of view (FOV), the intensity of the x-ray beam, and the angulation of the x-ray beam. It is important to recognize that measures to control the patient radiation dose also control the dose received by the operator and staff (Klein et al, 2009). The “as low as reasonably achievable” (ALARA) radiation safety principle focuses on minimizing ionizing radiation doses. The three major principles inherent to ALARA are: These principles apply to ionizing radiation in general, but are more relevant to fluoroscopy than CT. Regarding time and distance, CT scans take seconds, with the distance between the CT’s x-ray source and the patient remaining relatively constant. The technologist is in a control room outside the CT scanner, shielded from radiation by lead-lined walls and doors. Exposure to fluoroscopy is longer (minutes) and varies by procedure. Distance is a primary method providing protection because radiation exposure follows the inverse square law. With fluoroscopy the distance between the patient and the x-ray tube varies. The team ensures the patient is positioned as far from the x-ray tube and as close to the image intensifier as possible for optimal image quality and minimal radiation dose (Mauro, 2009). Prolonged procedures using fluoroscopy should change the beam’s entry point to reduce repeated exposure to the same area. Staff members in the control room do not wear lead. Staff members in the procedure room during fluoroscopy wear lead in addition to radiation protection provided by table-side lead apron drapes, ceiling-suspended lead acrylic shields, and movable lead acrylic shields (Figure 29-5). Wrap-around lead aprons and thyroid shields with a minimum of 0.5 mm of lead must be worn by personnel in the room during fluoroscopy. Leaded eyeglasses provide protection against cataract development for the equipment operator and personnel adjacent to the operator. Personnel radiation monitoring is an important part of radiation safety. Personal radiation dosimeters are worn on the trunk (under the apron) and at the collar level (outside the apron) to measure exposure. These dosimeters should not be left on aprons because this would cause inaccurate readings when an apron is worn by another user. The Nuclear Regulatory Commission (NRC) states that no occupationally exposed personnel exceed 50 mSv per year (NRC, 2012). Dosimeter frequency monitoring depends on the institution’s policies and procedures and governing laws. Outside the hospital, regulatory and manufacturer initiatives exist to reduce radiation exposure. The U.S. Food and Drug Administration (FDA) requires that fluoroscopy equipment produce an audible warning sound after 5 minutes of fluoroscopy. Other radiation reduction techniques and applications include beam collimation (Figure 29-6), pulsed versus continuous fluoroscopy, digital fluoroscopy imaging techniques, and specific dose-reduction protocols for CT and fluoroscopy. The preferred modality for the pregnant patient does not use ionizing radiation (e.g., ultrasound and MRI). All efforts should be made to minimize the exposure, with consideration of risk versus benefit for each clinical scenario. There is potential for the embryo or fetal radiation exposure during diagnostic or therapeutic procedures for pregnant women undergoing x-ray, fluoroscopy, or CT scans. Risk depends on the gestational age at the time of exposure, fetal cellular repair mechanisms, and the absorbed radiation dose level (Brent, 2011). With CT, the fetal radiation dose varies with the proximity of the uterus to the scanning plane, patient thickness, depth of the fetus, and imaging techniques. Lead shielding is at the discretion of the practitioner if it will not interfere with the scan field, although the dose from external scattered radiation is minimal (Radiological Society of North America [RSNA], 2007). Depending on the institution, the perioperative nurse initiates patient contact to schedule the procedure and perform an assessment, either by telephone or at the hospital. The nurse provides clear verbal instructions regarding preprocedure lab work or special studies: the time, date, and place to arrive on the day of the procedure; advance directives; insurance information; and nothing per mouth (NPO) status. Most patients are NPO after midnight, although angiography patients receiving contrast may continue water as prophylaxis against the dehydrating effects of contrast. Patients undergoing MRI have additional metal screenings. Same-day procedure patients must adhere to the prerequisites for same-day discharge. The determination of special needs (e.g., interpreters, claustrophobia, transportation) and the corrective or preventive actions are also components of the preprocedure phone call or visit. Most institutions use a checklist for the initial screening. The assessment and preparation for IR patients includes a complete medical history and physical, nursing assessment with care plan, informed consent, patient teaching, and the development of procedural sedation or analgesia planning. Due to the broad scope of IR, it is important to establish interdisciplinary patient preparation protocols. These include the requirement and completion of specific consultations (e.g., nephrology, oncology, anesthesia, orthopedics, gynecology, urology, etc.) as well as specific laboratory testing, urinalysis, and other required screenings and tests. Previous radiologic studies should be available for evaluating and planning procedure specifics. The physician performing the procedure determines protocol specifics including the access site, side and approach, imaging modality, contrast use, and device selection. Other considerations include specific medications, anesthesia plan, premedication for history of contrast reactions, antibiotic requirements, blood products, and use of closure devices. Assessment includes obtaining the patient’s height and weight, a brief history of the present illness, and past history, including renal dysfunction, coagulopathies, and allergies (including contrast medium allergy). The nurse verifies the patient’s current weight for medication dose calculation and to ensure compliance with the manufacturer’s modality table weight tolerance. For bariatric patients, the ARIN position statement on bariatric patient safety (2011) states preadmission planning for the bariatric patient includes careful screening for comorbidities and determining special equipment needs and history of past procedure sedation. Preadmission information includes height, weight, and body mass index (BMI) calculation. Depending on the patient’s body habitus, he or she may be within weight tolerance but the girth prohibits the scan. The average CT and MRI gantry size (bore opening) is 70 cm but does not factor in the table or instruments for a procedure. The nurse or technologist can use an appropriate diameter hula hoop to determine if a patient will fit in the scanner. The nurse performs and marks the initial distal pulse assessments’ presence or absence and quality for patients undergoing angiography before and after the procedure. An 18- or 20-gauge angiocath is standard for administrating medications, contrast, and hydration. Intravenous (IV) placement is usually antecubital but varies depending on the procedure, patient positioning, and vascular access. For neuroangiography procedures, the nursing neurologic assessment should include notation of difficulty with speech, visual disturbances, facial weakness, and any motor or sensory loss in the extremities, prior to the procedure start. This assessment provides a comparison during the procedure and recovery. Timing and selection of preprocedure antibiotics should be in accordance with regulatory agencies and facility and professional association guidelines. Please refer to Appendix A for standard labs. The referring physician and interventionalist collaborate to determine when and if to stop anticoagulants. Physician collaboration is essential regarding the use of aspirin-containing medication; nonsteroidal antiinflammatory drugs (NSAIDs) and antiplatelet medications. Some patients require bridging protocols (e.g., short-acting injected low-molecular-weight heparin) to wean oral anticoagulants while maintaining necessary anticoagulation until the procedure. The nurse provides clear instructions to the patients on continuing and discontinuing medications prior to the procedure. On admission the nurse measures the appropriate coagulation profiles prior to the start of the procedure according to hospital policy. All patients undergoing cardiac or pulmonary procedures and patients with a history of cardiopulmonary disease should have electrocardiograms (ECGs). The nurse gives specific dosing instruction to patients taking any hypertensive, antiarrhythmic, and cardiac medications prior to surgery. Patients with asthma should take their medications the day of the procedure and bring their inhalers. When appropriate, the anesthesia department should be consulted regarding airway management. The nurse should note any history or suspected problems with sleep apnea. Patients using continuous positive airway pressure (CPAP) or bilevel positive airway pressure (BiPAP) at home should bring their own machines due to the possibility of inducing sleep during sedation. Patients receiving sedation should undergo evaluation for obstructive sleep apnea (OSA) as per the American Society of Anesthesiologists’ guidelines to identify adverse sedation reaction risk (Minokadeh et al, 2011). Diabetic patients who take oral hypoglycemic medications should take their routine dose on the morning of the procedure. The nurse obtains blood glucose on arrival to the procedure room via a fingerstick and consults the appropriate physician for insulin adjustment if the blood sugar is greater than 250 mg/dL. Additional screening of diabetic patients for metformin and medications containing metformin is critical (Box 29-3). These medications should be discontinued for 48 hours after the procedure in patients receiving contrast due to the risk of lactic acidosis. The ARIN clinical practice guideline (2006) recommends evaluating renal function before resuming metformin or metformin-containing medications. It is not necessary to discontinue metformin or drugs containing metformin prior to gadolinium-enhanced MRI studies using normal dosage regimens (0.1 to 0.3 mmol/kg of body weight). The ACR (2009) does recommend withholding metformin and drugs containing metformin with large doses of gadolinium, due to potential nephrotoxicity. Preexisting renal disease and hydration status increase the risk of nephrotoxicity. The nurse obtains serum creatinine and glomerular filtration rates prior to contrast administration in patients with history of kidney disease or surgery, family history of kidney failure, diabetes, paraproteinemia syndromes or diseases (e.g., multiple myeloma), collagen vascular disease (e.g., scleroderma, systemic lupus erythematosus), metformin-containing medications, chronic or high doses of NSAIDs, or regular use of nephrotoxic medications, such as aminoglycosides. Preprocedural considerations for renal disease patients include adequate hydration, coordination of contrast media volume, and type to preprocedure kidney function tests (Naidu et al, 2012). If risk exceeds benefit, the physician may substitute another imaging modality. The nurse determines and documents any prior exposure to, or history of, contrast media allergic reactions. Predisposing allergic risk factors include asthma, renal insufficiency, significant cardiac disease, anxiety, paraproteinemia, particularly multiple myeloma. Common pretreatment protocols for contrast allergies are steroids—32 mg methylprednisolone 12 hours before and 2 hours prior to the procedure or 50 mg prednisone orally 13 hours, 7 hours, and 1 hour prior to procedure; and IV diphenhydramine 25 to 50 mg as per the physician’s order. Beta-blockers may increase the risk of reaction to contrast media or increase the severity of a reaction. Thyroid disease may increase the risk of delayed hyperthyroidism, and contrast media may be a contraindication in patients with thyroid cancer. The physiologic and emotional status of the patient contributes to the incidence and severity of adverse reactions. Often the procedure diagnosis is the source of anxiety more so than the procedure. Patients may experience claustrophobia when undergoing CT or MRI, or any IR procedure in which sterile draping around the head, face, and neck occurs. Reassurance that sedation and analgesia will be available may help to allay fears. Other alternatives include complementary or alternative therapies, such as verbal relaxation techniques, music distraction, and therapeutic presence. The ACR and SIR (2011) Practice Guideline on Informed Consent for Image-Guided Procedures advocates obtaining consent for invasive diagnostic and therapeutic procedures requiring moderate sedation. The physician or other qualified personnel assisting the physician should explain the procedure, answer all questions, and arrange for appropriate documentation of informed consent. In the consent discussion, the physician should explain the likelihood and characteristics of radiation injury, particularly for procedures associated with higher levels of radiation (e.g., transjugular intrahepatic portosystemic shunt [TIPS], embolization, stroke therapy, biliary draining, visceral angioplasty, stent placement, vertebroplasty, and radiofrequency ablation [RFA]). The ACR guideline (2011) notes the omission of IV contrast consent is in accordance to state law, institutional and departmental policy, and surrounding community practice. Nursing diagnoses related to the care of patients undergoing IR procedures might include the following:
Interventional and Image-Guided Procedures
Interventional Radiology
Imaging and the Operating Room
Radiology Terminology
Hybrid OR
Location.
Personnel and Staffing Requirements for a Hybrid OR.
Radiology Nursing
Interventional Radiology Nursing
Imaging Overview
Radiography.
CT Scan.
Fluoroscopy.
MRI.
Ultrasound.
Radiation Physics
Ionizing Radiation.
Tissue
Total Acute Dose Threshold (Gy)
Time to Develop Effect
Detectable opacities
0.5-2
>1 year
Cataract formation
5.0
>1 year
Skin reddening
3-6
1-4 weeks
Temporary hair loss
4
2-3 weeks
Skin death and scarring
5-10
1-4 weeks
Temporary sterility
0.15
3-9 weeks
Permanent sterility
3.5-6
3 weeks
Mucosa lining loss
6
6-9 days
Reduced RBC production
0.5
1-2 months
Units of Measurement.
Average Adult Effective Dose (mSv)
Estimated Dose Equivalent (Number of Chest X-Rays)
Dental x-ray
0.005-0.01
0.25-0.5
Chest x-ray
0.02
1
CT scan
2-16
100-800
Nuclear medicine
0.2-41
10-2050
Fluoroscopy
5-70
250-3500
Fluoroscopic Dose Measurement.
Procedure
Approximate Skin Dose
TIPS
2168 mGy
Nephrostomy
258 mGy
Neuroembolization—head
1977 mGy
Neuroembolization—spine
3739 mGy
IVC filter placement
193 mGy
Biliary drainage
781 mGy
Hepatic embolization
1959 mGy
PCI
2 Gy
PTCA and CA
1407 mGy
CT Dose Measurement.
Radiation Dose Factors.
ALARA.
Protection and Regulation.
Pregnancy and Radiation.
Nursing Care of the Interventional Radiology Patient
Preliminary Evaluation
Assessment
Labs.
Cardiopulmonary.
Diabetes.
Renal Disease.
Contrast Allergies and Other Considerations.
Consent.
Nursing Diagnosis
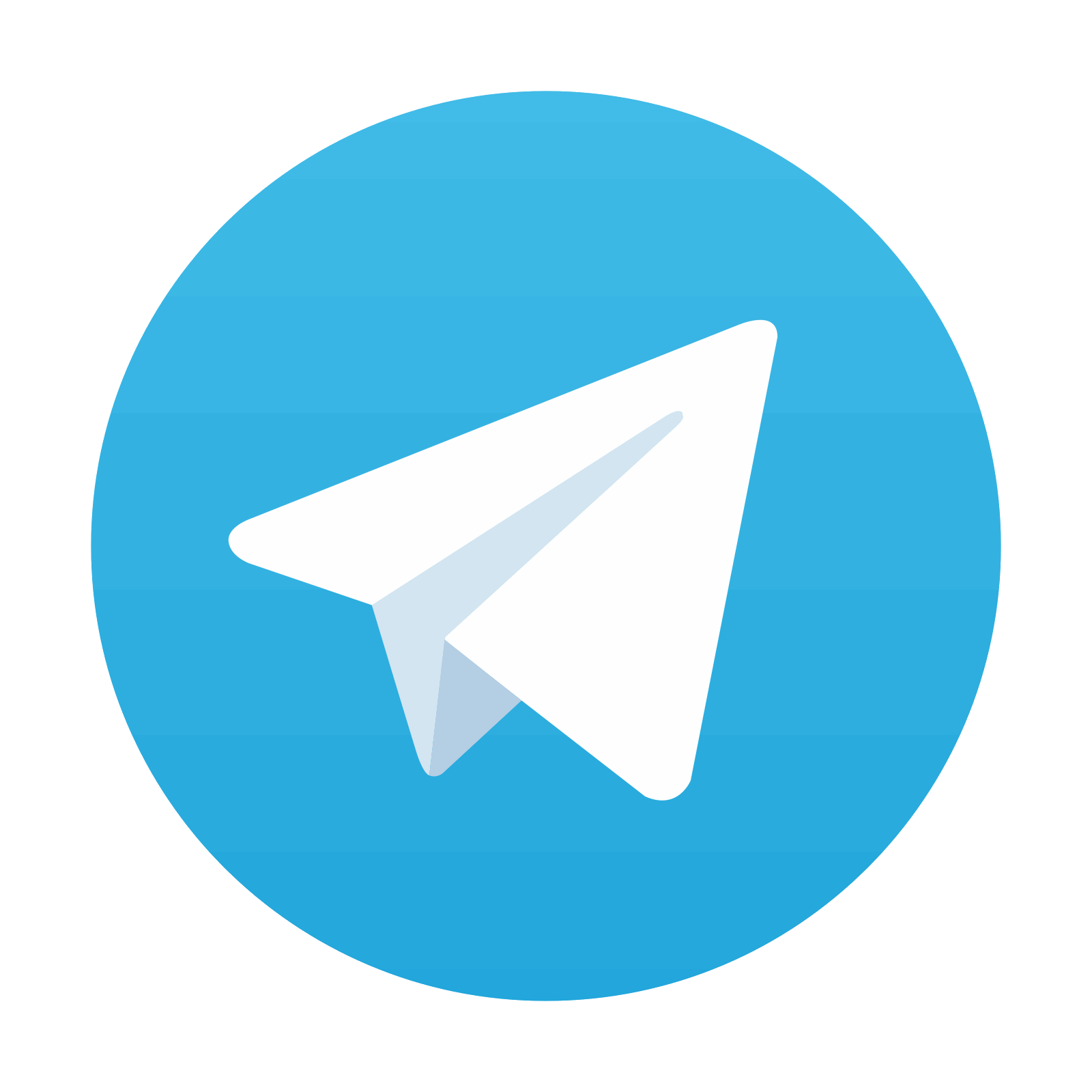
Stay updated, free articles. Join our Telegram channel

Full access? Get Clinical Tree
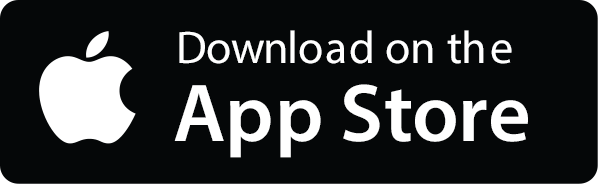
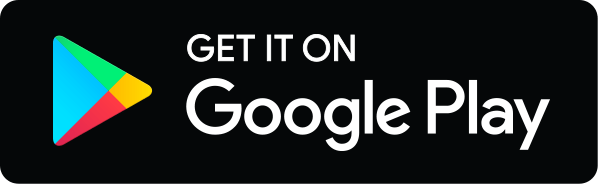