CHAPTER 8 INTERACTIONS WITH HERBAL AND NATURAL MEDICINES
INTERACTION MECHANISMS
PHARMACOKINETIC INTERACTIONS
Factors affecting absorption
Mucilaginous herbs
Although little research has been conducted to determine the effects of herbal medicines on the absorption of other medicines, one double-blind study found that guar gum slowed the absorption rate of digoxin, but did not alter the extent of absorption, whereas penicillin absorption was both slowed and reduced (Huupponen et al 1984). This brings into question the effects of other gums and highly mucilaginous herbal medicines, such as Ulmus fulvus (slippery elm), Althea officinalis (marshmallow) and Plantago ovata (psyllium). Poorly lipid soluble, the mucilaginous content forms an additional physical barrier to absorption, but whether this will have a clinically significant effect on rate and/or extent of absorption of other medicines is uncertain and remains to be tested.
Intrinsic drug transporters
Until recently, when an orally administered medicine exhibited poor absorption, it was generally assumed that this was because of either physicochemical problems or significant first-pass hepatic metabolism. Recently, it has been recognised that for many medicines poor oral bioavailability could be related to the influence of transporter proteins (Benet & Cummins 2001). Transporter proteins are associated with the transfer of some medicines from the intestinal lumen, through the biological barrier of the intestinal mucosa, into the systemic circulation and back again. Transporters fall into two main categories: carriers and pumps. Carriers are involved in three types of transport processes: facilitated diffusion, co-transport and counter-transport. Pumps are distinguished from carriers by the linkage of transport to an external source of energy. Examples of transporters in the intestine are P-glycoprotein (P-gp), members of the multi-drug-resistance-associated protein family (breast-cancer resistance protein, organic cation and anion transporters), and members of the organic anion polypeptide family (Wagner et al 2001). Of these, P-gp is the most studied (see Clinical note).
Herbal and natural medicines affecting P-gp
In 1999, clinical testing found that St John’s wort significantly reduces serum levels of digoxin after 10 days’ co-administration (Johne et al 1999). The suspected mechanism of interaction was chiefly liver enzyme induction; however, the magnitude of effect seen in this study, and in others, suggested that P-gp induction was involved (Hennessy et al 2002). More recently, several clinical tests have confirmed that St John’s wort has significant P-gp induction effects. One study found up to a 4.2-fold increase in P-gp expression compared with a placebo after 16 days’ administration. Similar
Clinical note — P-glycoprotein: an important drug transporter
P-glycoprotein was first studied in the context of cancer research, where its overexpression in tumour cells is associated with multi-drug resistance (Jodoin et al 2002). In cancer cells, P-gp is one of the transporters responsible for actively expelling chemotherapeutic drugs from cells, thereby decreasing intracellular concentrations and thus drug efficacy. As a result, identification of those substances that reduce P-gp expression and can be administered safely with chemotherapeutic agents is being investigated.
P-gp is found on the surface of hepatocytes, and epithelial cells of the renal tubules, the intestine, placenta and capillaries in the brain (Lin 2003). It plays an important role in the processes of absorption, distribution, metabolism and excretion of medicines. P-gp has a counter-transport activity, meaning it can transport medicines from the blood back into the gastrointestinal tract, thereby reducing bioavailability.
In humans, P-gp demonstrates genetic polymorphism, which may partly account for the inter-individual variability seen in drug absorption. One study conducted in 25 volunteers supports this idea and found that a greater than eightfold difference in expression of intestinal P-gp is possible (Lown et al 1997).
Some important P-gp substrates are:
results were seen in another study in which 14 days’ administration of St John’s wort resulted in a 1.4-fold increased expression of duodenal P-gp (Durr et al 2000). Induction of P-gp is attributed to pregnane X receptor activation by the hyperforin constituent (Lin 2003). Low hyperforin products are therefore less likely to induce P-gp.
St John’s wort is not the only natural substance found to influence P-gp. In vitro studies suggest that rosemary extract may have the opposite effect, inhibiting P-gp. Treating multi-drug-resistant mammary tumour cells with rosemary extract produced an increase in intracellular concentrations of doxorubicin and vinblastine (both P-gp substrates) (Plouzek et al 1999). The same effects were not seen in cells that lack P-gp expression, suggesting that rosemary extract inhibits P-gp activity. The isoflavone genistein has also been investigated, with some results suggesting inhibition of P-gp-mediated drug transport (Castro & Altenberg 1997). Other studies have found that different polyphenols, such as green tea polyphenols, resveratrol (a polyphenol from red wine), curcumin, caffeine, theanine and methoxyflavones from orange, may inhibit drug transport via P-gp (Jodoin et al 2002).
Grapefruit juice is well known to interact with a number of medicines, so it is not surprising that it has also been investigated for effects on P-gp. Currently, evidence is conflicting, as several studies have found that components in grapefruit juice inhibit P-gp (Eagling et al 1999, Ohnishi et al 2000, Soldner et al 1999, Spahn-Langguth & Langguth 2001), whereas a randomised, crossover clinical study found no significant effects (Becquemont et al 2001). A more recent in vitro study produced similar results with 5% normal concentration of grapefruit, orange and apple juices; however, inhibition of other transporter proteins was observed (Dresser et al 2002).
New research suggests that quercetin inhibits P-gp expression (Choi & Li 2005, Chung et al 2005, Kitagawa et al 2005, Wang et al 2004). Studies with experimental models have demonstrated that pretreatment with quercetin increases the bioavailability of the calcium-channel blocker, diltiazem (Choi & Li 2005), and of digoxin (Wang et al 2004). Intriguingly, one in vivo study found quercetin significantly decreased the oral bioavailability of cyclosporin, which is the opposite to what would be expected, suggesting that other mechanisms may also be involved (Hsiu et al 2002).
Although in vitro studies have also identified an inhibitory effect on P-gp for silymarin, the active constituent group in St Mary’s thistle (Chung et al 2005, Zhang & Morris 2003), recent clinical testing found no significant effect in vivo (Gurley et al 2006).
Table 8.1 (p 98) lists herbal and natural medicines that have suspected or known effects on P-gp, together with the research that gives evidence for these effects.
TABLE 8.1 Herbal and Natural Medicines with Suspected or Known Effects on P-Glycoprotein
Herbal/natural medicine | Effect | Evidence |
---|---|---|
St John’s wort | Induction | |
Grapefruit juice | Inhibition | |
Grapefruit juice | No effect | Clinical study (Becquemont et al 2001) |
Orange and apple juice | Inhibition | |
Rosemary extract | Inhibition | In vitro (Plouzek et al 1999) |
Genistein and diadzein | Inhibition | In vitro (Evans 2000, Castro & Altenberg 1997) |
Genistein and diadzein | Possible induction | In vitro (den Boer et al 1998) |
Resveratrol | Inhibition | In vitro (Jodoin et al 2002) |
Curcumin | Inhibition | In vitro (Anuchapreeda et al 2002, Romiti et al 1998) |
Quercetin | Inhibition | In vitro and in vivo (Choi & Li 2005, Chung et al 2005, Kitagawa et al 2005, Scambia et al 1994, Wang et al 2004) |
Green tea polyphenols, especially epigallocatechin gallate | Inhibition | In vitro (Jodoin et al 2002, Sadzuka et al 2000) |
Piperine (a major component of black pepper) | Inhibition | In vivo (Bhardwaj et al 2002) |
St Mary’s thistle | Inhibition | In vitro tests (Chung et al 2005, Zhang & Morris 2003) |
St Mary’s thistle | No effect | Human study (Gurley et al 2006) |
Factors affecting metabolism
Metabolism can occur before and during absorption, thereby limiting the amount of drug reaching the systemic circulation. In the intestinal lumen, digestive enzymes and bowel flora are capable of causing a wide range of metabolic reactions. The intestinal mucosa is also capable of metabolising drugs, with new research suggesting it is a major metabolic organ for some medicines (Doherty & Charman 2002).
For many medicines, metabolism chiefly occurs in the liver in two apparent phases, known as phase I or functionalisation reactions and phase II or conjugation reactions. Phase I reactions include oxidation, hydroxylation, dealkylation and reduction. Examples of Phase I reactions include conversion of an active drug to an inactive, less active, more active or toxic metabolite, and conversion of an inactive prodrug to an active metabolite. Phase II reactions include glucuronidation, sulfation, acetylation and methylation glutathionation, glycination and other amino acid conjugations (taurine, glutamine, carnitine, arginine), and usually result in the formation of inactive compounds that are water-soluble and easily excreted (Blower et al 2005).
Cytochromes
Cytochrome P450 is a generic term for a super-family of enzymes (haem containing mono-oxygenases) that have existed throughout nature since the beginning of life more than 3.5 billion years ago (Pirmohamed & Park 2003). The P450s are found chiefly in the liver, but also to a lesser extent in the intestines, kidneys, skin and lungs. These enzymes are responsible for foreign compound metabolism, which evolved about 400–500 million years ago to enable animals to detoxify chemicals in plants. The cytochrome P450 (CYP) enzymes are the most powerful in vivo oxidising agents and are able to catalyse the oxidative biotransformation of a wide range of chemically and biologically unrelated exogenous and endogenous substrates. They are named by a root symbol CYP, followed by a number for family, a letter for subfamily, and another number for the specific gene. For example, CYP3A4 would refer to a specific enzyme from the cytochrome P450 system, family 3, subfamily A and gene 4. Three main CYP families (CYP1, 2, 3) are responsible for metabolism of therapeutic drugs. The different P450 isoforms vary in their abundance within the liver. Of these, the cytochromes CYP2C9, CYP2D6 and CYP3A4 are the most abundant in the human body (Pirmohamed & Park 2003).
The CYP2C sub-family accounts for 15–20% of the total P450 content of the liver, and metabolises approximately 20% of all drugs (Pirmohamed & Park 2003). The main member of this sub-family is CYP2C9, which is responsible for the metabolism of a number of compounds, including warfarin, phenytoin and various NSAIDs. Cytochrome 2D6 is responsible for the metabolism of approximately 25% of therapeutically used drugs, although it accounts for only < 5% of the total P450 content. The CYP3A sub-family accounts for 30% of the total P450 content and is responsible for metabolism of about 50% of therapeutic drugs. Table 8.2 provides examples of common drugs and the cytochromes chiefly responsible for their metabolism. For a more complete list that is frequently updated, see Flockhart 2007.
TABLE 8.2 Selected Drugs that Act as Substrates for CYP Enzymes
CYP enzyme | Drugs |
---|---|
CYP1A2 | amitriptyline, caffeine, melatonin, naproxen, paracetamol, tacrine, theophylline, verapamil, R-warfarin |
CYP2B6 | amitriptyline, diazepam, methadone, midazolam, tamoxifen, temazepam, testosterone |
CYP2C9 | amitriptyline, celecoxib, diclofenac, fluoxetine, ibuprofen, tamoxifen, S-warfarin |
CYP2C19 | amitriptyline, imipramine, indomethacin, omeprazole, progesterone, propranolol, R-warfarin |
CYP2D6 | amitriptyline, beta blockers, codeine, fluoxetine, flecainide, haloperidol, nicotine, ondansetron, paroxetine, sertraline, tamoxifen, tramadol |
CYP3A4 | alprazolam, caffeine, codeine, cyclosporin, erythromycin, HIV antivirals (e.g. indinavir), lovastatin, midazolam, oestradiol, ondansetron, progesterone, simvastatin, tamoxifen, taxol, testosterone |
For example, one study identified that 30% of Ethiopians had multiple copies of the 2D6 gene (up to 13), resulting in ultra-rapid metabolism of CYP2D6 substrates (Aklillu et al 1996). As a result, standard doses of CYP2D6 substrates (e.g. beta-blockers, some opioids and tricyclic antidepressants) will not produce anticipated or adequate responses, and higher drug doses are necessary to produce therapeutic effects. It has also been estimated that 7% of Caucasians lack CYP2D6. These individuals will not experience the anticipated therapeutic effects of prodrugs that are CYP2D6 substrates, such as codeine, and will appear more sensitive to the side effects of CYP2D6 substrates (e.g. beta-blockers, some opioids and tricyclic antidepressants) (USFDA 2006).
Because of overlapping substrates, many drug interactions involve both P-gp and CYP3A4.
Enzyme inhibition
Although inhibition of a CYP enzyme is generally regarded as raising the serum levels of an active drug, this is not always the case. If the drug involved is a prodrug, then it is inactive in its administered form and must be converted to its active form, usually via metabolic processes. If metabolism is slowed, then the production of active metabolites will also be slowed. An example is codeine, which is primarily metabolised by CYP2D6 into its active analgesic metabolite morphine; therefore, CYP2D6 inhibition has the potential to slow or reduce its analgesic activity.
Inhibitors do not all have the same strength and can be classified as strong, moderate or weak, depending on their effect on drug clearance compared to normal values. For example, Flockhart describes a strong inhibitor as one that causes more than 80% decrease in clearance, a moderate inhibitor as one that causes a 50–80% decrease in drug clearance, and a weak inhibitor as one that causes a 20–50% decrease in drug clearance (Flockhart 2007).
To date, the most studied natural substance capable of significantly inhibiting CYP enzymes is grapefruit. The finding that grapefruit juice markedly increases the bioavailability of some orally administered medicines was based on an unexpected observation from an interaction study between the calcium-channel antagonist felodipine and ethanol. In the study, grapefruit juice was used to mask the taste of ethanol, but actually affected the results by reducing CYP3A4 by 62% and significantly raising felodipine levels (Bailey et al 1998). Since then, the constituents of grapefruit juice have been extensively studied and found to affect the transport and metabolism of many other medicines (Eagling et al 1999, Kane & Lipsky 2000).
Increasingly, research is being conducted to determine whether other commonly used herbal medicines have an affect on CYP activity in vivo. For example, one research group in the United States screened Citrus aurantium, Echinacea purpurea, milk thistle (Silybum marianum), and saw palmetto (Serenoa repens) extracts for effects on CYP1A2, CYP2D6, CYP2E1 and CYP3A4 activity in healthy subjects (Gurley et al 2004). Of the four herbs, only E. purpurea was found to have any effect on the CYP enzymes tested, with a minor influence on CYP1A2 and CYP3A4. Using the same method, extracts of the herbs goldenseal (Hydrastis canadensis), black cohosh (Cimicifugaracemosa), kava kava (Piper methysticum) and valerian (Valeriana officinalis) were tested for effects on CYP1A2, CYP2D6, CYP2E1 or CYP3A4/5 activity (Gurley et al 2005). Goldenseal strongly inhibited CYP2D6 and CYP3A4/5 activity in vivo, whereas kava kava inhibited CYP2E1, black cohosh weakly inhibited CYP2D6 and no effect was observed for valerian. In a separate study, testing Panax ginseng and Ginkgo biloba, no effect was observed on CYP activity; however, garlic oil inhibited CYP2E1 activity by 39% (Gurley et al 2002). There are many other examples to be found in the individual monographs of this book.
Enzyme induction
Clinical tests have confirmed that long-term administration of St John’s wort has significant CYP inducer activity, particularly CYP3A4 (Durr et al 2000, Roby et al 2000, Ruschitzka et al 2000, Wang et al 2001). It appears that the hyperforin component is a potent ligand for the pregnane X receptor, which regulates expression of CYP3A4 mono-oxygenase. In this way, hyperforin increases the availability of CYP3A4, resulting in enzyme induction (Moore et al 2000).
Lack of in vitro — in vivo correlation
It is interesting to observe an apparent lack of in vitro – in vivo correlation with some studies of CYP. For instance, in vitro investigations implicate milk thistle extract and/or silibinin as inhibitors of human CYP3A4, CYP2C9, CYP2D6 and CYP2E1; however, in vivo evidence for CYP-mediated interactions by milk thistle is less compelling (Gurley et al 2004). This may be owing to poor bioavailability of key constituents, large inter-individual differences in absorption of constituents, inter-product variability in the ratios of constituent, poor dissolution of dosage forms or other mechanisms.
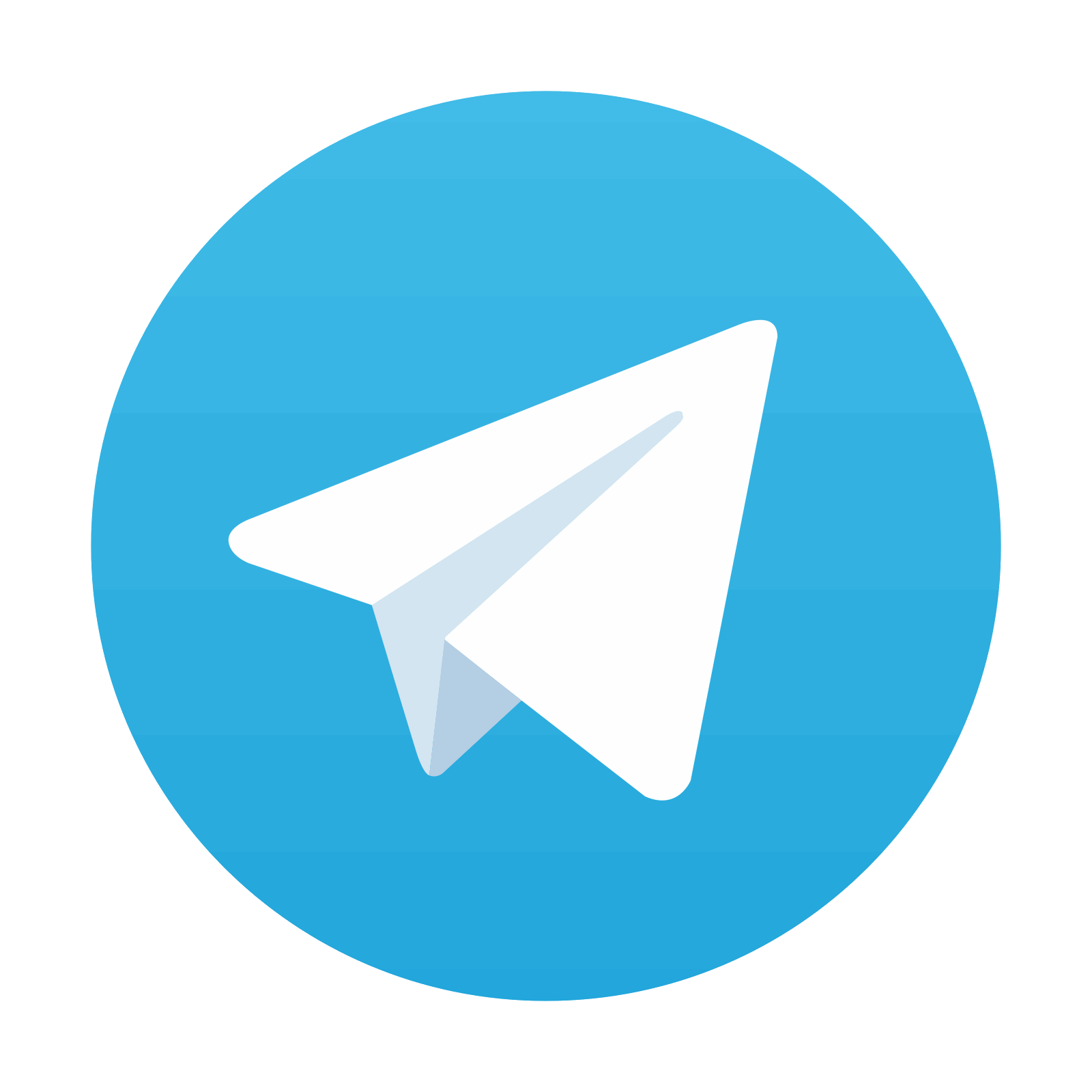
Stay updated, free articles. Join our Telegram channel

Full access? Get Clinical Tree
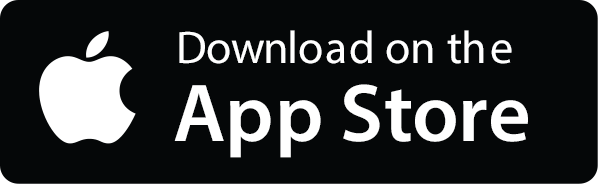
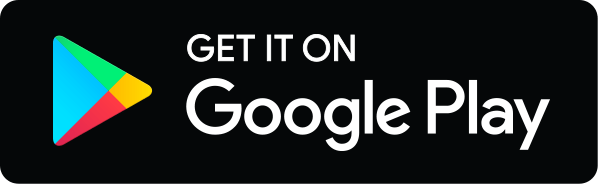