First author
Publication year
Country
No. of UC patients
SIRa
Reference
Stewenius
1995
Sweden
471
2.1
PMID:7636371
Wandall
2000
Denmark
801
1.7
PMID:10766327
Palli
2000
Italy
689
1.8
PMID:10982757
Jess
2006
USA
378
1.1
PMID:16618397
Jess
2007
Denmark
1575
1.1
PMID:17206705
Söderlund
2009
Sweden
4125
2.7
PMID:19422077
Herrinton
2012
USA
10,895
1.6
PMID:22609382
Jess
2012
Denmark
32,911
1.1b
PMID:22522090
van den Heuvel
2016
The Netherlands
1644
0.7
PMID:27170593
Cheddani
2016
France
474
0.9
PMID:27481308
In a recently published prospective study of >19,000 patients with IBD in France, the standardized incidence ratio (SIR) of colorectal cancer (CRC) was 2.2 for all patients [7]. Patients with IBD and long-standing extensive colitis were found to have an increased risk for CRC with a SIR of 7.0 [7]. In contrast, CRC risk was lower among IBD patients receiving thiopurine therapy [7]. Thus, these two studies are consistent with a 1.6–2.2 times increased risk of developing CRC in patients with IBD. These numbers are in accordance with a meta-analysis of population-based cohort studies that was published in 2013 and found a SIR for CRC of 1.7 for patients with IBD (PMID: 23448792) [8]. Close examination of population-based cohort studies from several countries reveals a consistent but modest increased risk for CRC in the range 0.7–2.7 both for patients with UC (Table 10.1) and CD (Table 10.2).
Table 10.2
Reported risk of colorectal cancer in patients with Crohn’s disease (CD)
First author | Publication year | Country | No. of CD patients | SIRa | Reference |
---|---|---|---|---|---|
Palli | 2000 | Italy | 231 | 1.4 | PMID:10982757 |
Jess | 2004 | Denmark | 374 | 1.6 | PMID:14984375 |
Jess | 2006 | USA | 314 | 1.9 | PMID:16618397 |
Jess | 2007 | Denmark | 641 | 1.4 | PMID:17206705 |
Söderlund | 2009 | Sweden | 3482 | 2.1 | PMID:19422077 |
Herrinton | 2012 | USA | 5603 | 1.6 | PMID:22609382 |
Jess | 2012 | Denmark | 14,463 | 0.9b | PMID:22522090 |
Beaugerie | 2013 | France | 11,759 | 2.4 | PMID:23541909 |
van den Heuvel | 2016 | The Netherlands | 1157 | 2.0 | PMID:27170593 |
Cheddani | 2016 | France | 370 | 2.5 | PMID:27481308 |
There is strong evidence that the risk of CRC for IBD patients may have decreased considerably over the past 30 years [9, 10]. A large cohort study in Denmark with >47,000 patients with IBD over a 30-year period (1979–2008) showed that the overall risk of CRC among patients with IBD was comparable with that of the general population [10]. However, increased CRC risk was observed for subgroups of patients with UC such as patients diagnosed in childhood or adolescence, those with long duration of disease, and those with concomitant primary sclerosing cholangitis [10]. For patients with UC, the overall relative risk for CRC decreased from 1.34 in 1979–1988 to 0.57 in 1999–2008. For CD patients, the relative CRC risk was 0.85 and did not change over time. Therefore, the authors of this large Danish cohort study concluded that a diagnosis of UC or CD no longer appears to increase patients’ risk of CRC, presumably due to improved therapies for patients with IBD [10]. Similarly, a nationwide study in the Netherlands including 78 general hospitals concluded that the risk of developing CRC in IBD patients was very low [11]. Interestingly, reduced CRC incidence was observed in IBD patients treated with immunosuppressive therapy or by tumour necrosis factor α (TNF-α) blockade. [11]. Thus, one can conclude that the CRC risk in IBD has been overestimated in the past, presumably due to bias in patient inclusion and flaws in statistical analysis. A major pitfall appears to be the use of referral centre-based rather than population-based cohorts which tend to overestimate the risks by including patients with more severe disease (PMID:23448792). An approximately twofold increased risk of developing CRC in patients with IBD is a more reliable estimate, which only weakly supports the proposed link between chronic inflammation and cancer. In contrast, long-term immunosuppressive treatment of IBD patients is associated with an increased risk for overall cancer including haematologic and skin cancers (PMID:27170593), consistent with a key role of the immune system in preventing cancer. Thus, a diagnosis of IBD may be considered a risk biomarker for immunosuppression-associated cancers rather than for CRC only.
Helicobacter pylori Infection and the Risk of Gastritis
Helicobacter pylori is a Gram-negative bacterium which colonizes the stomachs of about half of the world population. The prevalence of H. pylori infection varies widely according to geographic area [12]. Sequencing data from a worldwide collection of H. pylori strains suggest that anatomically modern humans were already infected with the bacterium before their migrations out of Africa [13]. A complete H. pylori genome was recently recovered from the stomach of a 5300-year-old mummy of an early European farmer (the Iceman), formally demonstrating that H. pylori has been a human pathogen for >5000 years [14]. Although the vast majority (>90%) of H. pylori infected people remain asymptomatic, H. pylori is considered the main causative agent behind gastritis and peptic ulcer. H. pylori (originally named Campylobacter) was first isolated from stomach biopsies by the Australian scientists J. Robin Warren and Barry Marshall [15]. H. pylori was reported to grow in close contact with the epithelium of the stomach, presumably near the neutral end of the pH gradient and protected by the overlying mucus [15]. Importantly, H. pylori was almost always detected in patients with active chronic gastritis, suggesting a causal relationship between H. pylori infection and inflammation. This hypothesis was strengthened by the analysis of biopsy specimens from 100 consecutive patients, revealing that the bacterium was present in almost all patients with active chronic gastritis, duodenal ulcers, or gastric ulcers [16]. Several studies have confirmed this association. Among 274 Italian patients with gastritis, H. pylori was found in 84%, while none of the 36 gastritis-free stomachs harboured the bacterium [17]. A case-control study in Finland with 33 patients with gastric ulcer and as many controls showed that H. pylori infection was significantly more frequent in the ulcer patients (57%) than in the non-ulcer cases (33%, p < 0.05) [18]. Notably, in the non-ulcer group, H. pylori infection was strongly associated with gastritis: 11 of 11 (100%) of the H. pylori infected patients had atrophic gastritis, as compared with 7 of 22 (32%) of the noninfected patients (p < 0.01) [18].
Helicobacter pylori Infection and Gastric Cancer Risk
Several studies have revealed that infection with H. pylori is associated with an increased risk of developing gastric cancer. For example, in a cohort of American men of Japanese ancestry living in Hawaii, 94% of patients with gastric carcinoma, but only 76% of the matched controls, had a positive test for H. pylori antibodies, implying an odds ratio of 6.0 [19]. As the level of antibody to H. pylori increased, there was a corresponding increase in the risk of gastric carcinoma [19]. Another American study in California with 109 patients with gastric adenocarcinoma reported 84% infection rate among patients and 61% for the matched controls [20]. Similar findings were made with patient cohorts in Japan and Norway [21] [22] [23] . Thus, although most H. pylori infected individuals remain healthy, there is a strong association between H. pylori infection and gastric cancer. Essentially, all patients with gastric cancer are colonized by H. pylori. Infection by H. pylori is now established as a risk factor for developing gastric cancer. In fact, H. pylori was classified as a carcinogen by the World Health Organization (WHO) in 1994 [24]. Thus, gastric carcinoma is considered a paradigm of infection-associated cancer.
Can H. pylori Infection or Gastritis Be Used as Biomarkers for Gastric Cancer Risk?
Although it is well known that most people infected with H. pylori never develop gastric cancer, several investigators have attempted to use H. pylori infection and/or gastritis as predictors of gastric cancer development. In a prospective study, 1526 Japanese patients with various gastrointestinal diseases (duodenal ulcers, gastric ulcers, gastric hyperplasia, or non-ulcer dyspepsia) at the time of enrolment were followed. Gastric cancer developed in 36 of 1246 H. pylori infected patients (2.9%) but in none of the 280 uninfected patients (p < 0.001) [25]. Interestingly, gastric cancer did not develop in any of the 253 patients with H. pylori infection who received eradication therapy [25]. In a second prospective study in Japan, H. pylori antibodies and serum pepsinogen I and II (used a markers of gastric atrophy) were measured for a total of 9293 participants representing the healthy Japanese population [26]. A total of 43 persons developed gastric cancer during the follow-up period. A significantly higher incidence of gastric cancer was observed for individuals with an ‘atrophic’ pepsinogen status. In this study several patients who developed gastric cancer were negative for H. pylori antibodies [26]. The authors speculated that in the most advanced cases of gastric atrophy, H. pylori levels may dramatically decrease and H. pylori antibodies may actually disappear [26]. This hypothesis is supported by a Finnish study in which 47 men with advanced H. pylori-positive atrophic gastritis were followed for 10 years [27]. H. pylori antibodies disappeared spontaneously in almost one-fourth of patients with advanced gastritis (n = 11), and this was accompanied by no or only a mild improvement of the gastric mucosa [27]. If H. pylori antibodies and the bacterium itself may spontaneously disappear in some patients with advanced gastritis and if gastritis after the disappearance of H. pylori still predisposes to cancer, this weakens the validity of H. pylori detection as a biomarker for gastric cancer. In conclusion, H. pylori seropositivity or the associated gastritis may be used as biomarkers to predict development of gastric cancer. This may be particularly useful in populations with a high incidence of gastric cancer such as in Japan. However, there is a very high rate of false positives.
Cytokines as Biomarkers of Inflammation and Cancer Prognosis
Central to the consideration of inflammation-associated biomarkers is the important role of soluble cytokines and other signalling factors that serve to initiate and maintain inflammation. Some of these are released from the liver, whereas others originate either from leukocytes or tissue-resident cells of inflammatory lesions. In the following section, we will focus on some selected cytokines. We give a detailed account of their sources, targets, and the signalling pathways they activate, as this information is critical to understanding inflammatory biomarkers in cancer.
MyD88 and Interleukin-1 (IL-1) Family Members
IL-1 is elevated in various types of cancers, and it is known that patients with IL-1-producing tumours have poor prognosis [28]. This knowledge has called for strategies to target IL-1 that will be detailed below. However, before we explain the possible functions of IL-1 and put them into the context of other IL-1-like cytokine effects (IL-18 and IL-33), we shall first describe the mechanisms by which these cytokines affect the immune system and may act to affect tumour development. Signalling induced by most members of the IL-1 family of cytokines and also the Toll-like receptor (TLR) family converges on MyD88 (myeloid differentiation primary response gene 88), a central adapter of the IL-1 receptor/TLR superfamily [29]. This central position of MyD88 (Fig. 10.1) in inflammation has boosted a strong interest in assessing tumour development when MyD88 is inactivated, and starting this account of the IL-1 family can be better explained by first examining the signalling events downstream from MyD88 activation.


Fig. 10.1
Schematic representation of receptors for IL-1, IL-18, and IL-33. IL-1 receptor (IL1R1) forms a heterodimer with the IL-1 receptor accessory protein (IL-RAcP, aka IL1R3) upon binding of IL-1α or IL-1β, leading to intracellular recruitment of MyD88 and downstream signalling to NF-kB and p38MAP. Interleukin-1 receptor antagonist (IL1Ra, aka IL1F3) competes with IL-1 for binding to IL1R1. IL-18 receptor (IL-18Rα, aka IL1R5) forms heterodimer with IL-18Rβ (aka IL1R7) to mediate signalling after binding IL18. IL18-binding protein (IL18BP) acts as decoy to intercept IL-18. IL-33 receptor (IL-33Ra, aka ST2 or IL1R4) forms heterodimer with IL-RAcP upon binding of IL-33. Soluble IL33R/ST2 acts as decoy to intercept IL-33
MyD88
The majority of studies imply that MyD88 signalling promotes carcinogenesis in many cancer models. In chemical carcinogenesis models of skin and liver, tumour induction was inhibited in response to genetic lack of MyD88 [30, 31]. Reduced intestinal tumour growth was also observed in Myd88 −/− mice subjected to multiple injections of the carcinogen azoxymethane (AOM) in comparison with WT controls [32]. Furthermore, MyD88 signalling was shown to be required for AOM-enhanced colon carcinogenesis in Il10 −/− mice [33] and in the Apc Min/+ mouse model of spontaneous intestinal tumorigenesis; MyD88 signalling contributed to adenoma growth and progression [32].
On the other hand, when combining AOM with the chemical irritant DSS, MyD88 was found to protect against the development of colitis-induced cancer [34], contrasting the findings obtained in response to AOM alone [32]. Moreover, MyD88 activation protects against the development of myeloproliferative neoplasia (MPN) [35]. A protective role of MyD88 against cancer may be explained by the fact that the immune system naturally protects against cancer and that certain types of inflammation prevent malignancies, while other types promote cancer [1, 36, 37].
These observations have nevertheless raised the following question: what are the signals that act upstream of MyD88? Central to the activation of IL-1 and IL-18 is their cleavage by caspase-1, generated by the inflammasome, a composite protein complex strongly involved in the regulation of inflammation and autoimmunity [38]. Indeed, inflammasome defects have been shown to increase tumour growth in several colitis-derived murine cancer models [39–41]. However, the biological effects of IL-1 and IL-18 differ in many respects. Despite the fact that both cytokines signal via MyD88, IL-1 is a strong driver of NF-kB signalling and the MAPK p38 pathway, whereas IL-18 signal transduction mainly involves the latter [42].
Interleukin-1
In carcinogenesis-driven experimental skin cancer, lack of IL-1β led to slower tumour growth, and, conversely, when IL-1 receptor antagonist was lacking, tumour growth was accelerated [43]. In the same vein, IL-1 contributes to the development of preneoplastic gastric lesions in the Helicobacter-driven model of intestinal neoplasia [44]. On the other hand, genetic lack of IL-1R does not alter outcome of the AOM/DSS-driven colitis model [34]. Several studies in mice have also documented protective functions of IL-1α and IL-1β against cancer [1, 45].
The effect of targeting IL-1 signalling in cancer treatment has been assessed in some clinical trials. A phase 2 clinical trial with patients with smouldering or indolent multiple myeloma indicated that blocking of IL-1 activity by recombinant IL-1 receptor antagonist (Anakinra) may result in prolongation of progression-free disease [46]. Moreover, a human antibody to IL-1α was tested in a phase I trial of patients that were refractory to antitumour therapies and losing weight and found to induce a significant increase in lean body mass [47].
Interleukin-18
IL-18 is a member of the IL-1 family of cytokines best known for its role in promoting IFN-γ production and Th1 polarization of T cells [48] and a cytokine that has also attracted interest in the field of immuno-oncology. In the Helicobacter-driven model of intestinal neoplasia, IL-18, in contrast to IL-1, appears to prevent the onset of gastric cancer [44], perhaps by mediating conversion of T cells to a regulatory phenotype [49]. Likewise, mice lacking either IL-18 or IL-18R were highly susceptible to tumour formation in a colitis-driven model of colorectal cancer [34]. Indeed, several studies have implicated IL-18 production as the main mediator that confers protection against colorectal tumour formation downstream of the Nlrp3 inflammasome [39]. In fact, in a model lacking caspase-1, substitution with bioactive IL-18 could reverse epithelial dysplasia [41]. IL-18 administration also mediated regression of melanoma and sarcoma [50], apparently mediated by IFN-γ [51] and perhaps involving the antiangiogenic effect of IFN-γ-responsive chemokines CXCL9 and CXCL10 [52]. It deserves mention that IL18-primed human NK cells develop a distinct helper phenotype that shows reduced cytotoxic function and instead, via production of IFN-γ, promote tumour-specific Th1 and CTL responses [53].
The experimental evidence of an antineoplastic role of IL-18 is in apparent contradiction to the elevated levels of IL-18 seen, for example, in human ovarian cancer [54]. However, it appears that while tumour cells have the capacity to synthesize high levels of IL-18, it is the full-length pro-IL-18 that has not been processed by capase-1, and accordingly, it has no biological activity [54]. Likewise, elevated levels of soluble IL-18 binding protein have the capacity to neutralize the effect of IL-18 [55, 56]. The preclinical efficacy of IL-18 has initiated clinical trials of IL-18 alone or in combination, showing that IL-18 has low toxicity in man but a limited therapeutic effect as a single agent [48].
Interleukin-33
Interleukin-33 (IL-33, also known as IL1F11) is the most recently identified member of the IL-1 family of cytokines [57]. Its involvement in cancer development has not yet been reviewed, and it will therefore be dealt with in more detail. This is underscored by the fact that, like IL-1α, IL-33 has a complex biology based on the observation that it acts as a nuclear factor in many cell types, yet when released by damaged cells, it binds to a more conventional surface membrane receptor (IL-33R, also known as ST2 or IL-1R4) that resembles other members of the IL-1/Toll-like receptor (TIR) superfamily (Fig. 10.1). It is important to understand this dual function when interpreting results in cancer biology studies that modulate the function of IL-33 and its receptor.
The identification of IL-33 was initiated by the discovery that serum-stimulated fibroblasts expressed a molecule that partly resembled the IL-1 receptor [58]. This putative IL-1 family receptor member was designated ST2 and widely characterized as an orphan receptor until scientists working at Genentech identified IL-33 as its ligand [57]. In a genome-wide search and modelling for novel members of the IL-1 family, Schmitz et al. [57] revealed a sequence that was first characterized as a transcript induced in vessels in a model of vasospasm after subarachnoid haemorrhage [59]. This protein was later found to be abundantly expressed in the nuclei of endothelial cells in most healthy human tissues, as well as in some epithelia and in the fibroblastic reticular cells of lymphoid tissues [60, 61]. In lesions of inflammation, it is also expressed by activated fibroblasts, and the repertoire of epithelial cells that express IL-33 is expanded [62]. There are also important species differences [62], but as a general principle, IL-33 is expressed in intact cells as a nuclear protein thought to affect transcriptional behaviour.
The other aspect of IL-33 biology occurs when IL-33 is released by necrotic or otherwise damaged cells and acts as an active cytokine that binds to IL-33 receptor (IL-33, previously designed ST2) and initiates a signalling cascade that also involves signalling via MyD88, p38MAPK, and NF-kB (Fig. 10.1) [57]. Very interestingly, the signalling pathways activated by IL-33 show very strong similarities to those well characterized in response to IL-1. Indeed, despite numerous papers that analysed IL-33 signalling, only one publication reported a side-by-side comparison of the transcriptional response, concluding a virtually identical profile and instead describing mechanisms of regulation related to expression of IL-33R in relation to cell cycle [63]. Further complicating the biology of ST2 is the fact that alternative splicing of mRNA results in transcripts that also encode a shorter, soluble form of ST2 (sST2) that lacks the transmembrane region and is thought to act as a decoy receptor (Fig. 10.1).
Serum levels of IL-33 are reportedly elevated in breast, lung, and gastric cancer and are associated with tumour progression and metastasis [64–66]. On the other hand, multiple myeloma patients had an inverse association between IL-33 levels [67], whereas a study reporting on serum values from patients with hepatocellular carcinoma found no difference when compared to controls [68]. Nevertheless, elevated serum levels of soluble ST2 were identified as a negative prognostic factor in hepatocellular carcinoma [68]. Soluble ST2 is also a negative prognostic factor in oestrogen receptor-positive breast cancer [69]. At the level of tissue expression, increased numbers of IL-33-positive tumour cells were reported to correlate with poor prognosis in carcinoma of the tongue [70]. By contrast, IL-33 has been associated with prolonged patient survival in hepatocellular carcinoma, based on the degree of IL-33-expressing cells in relation to survival [71].
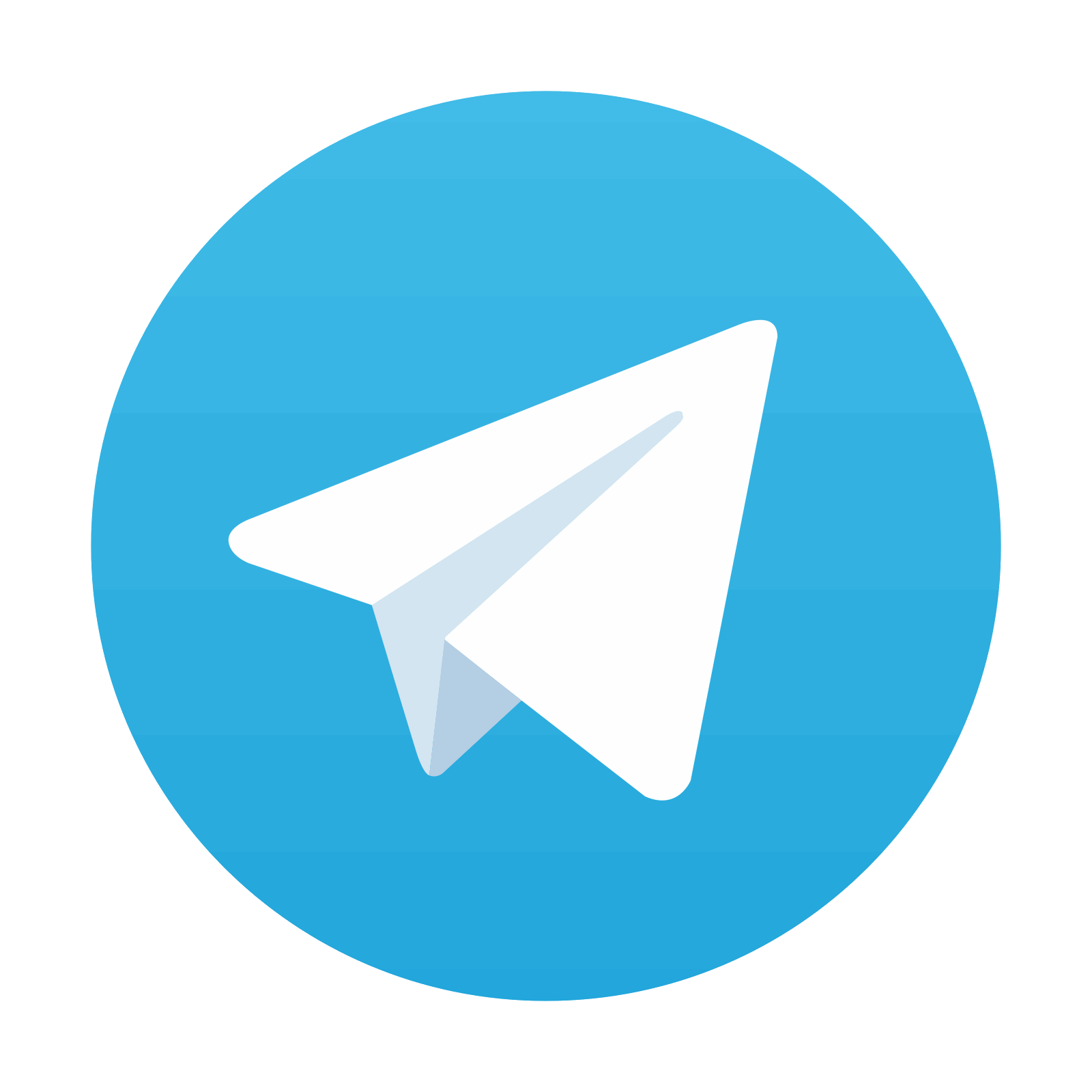
Stay updated, free articles. Join our Telegram channel

Full access? Get Clinical Tree
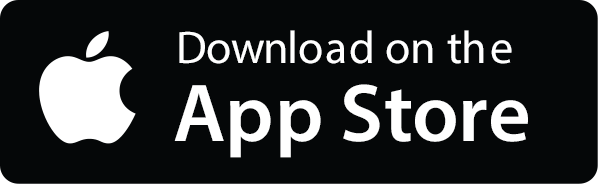
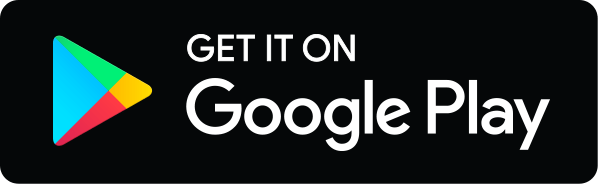