Fig. 7.1
Arachidonic acid metabolism via cyclooxygenase (COX), lipoxygenase (LOX), and cytochrome P450 (CYP450) enzymes. Lipids highlighted include PGE2, LTB4, LTC4, LTD4, LTE4, 11,12-EET, and 14,15-EET for their diverse roles in inflammation-associated tumor pathogenesis
Prostaglandin E2
Prostaglandin E2 (PGE2) is a characteristically pro-inflammatory bioactive lipid synthesized from arachidonic acid initially by cyclooxygenase 1 (COX-1) and cyclooxygenase 2 (COX-2) and then by PGE synthase. Both PGE2 and COX-2 expression have been implicated in inflammation and cancer. PGE2 has been most notably characterized in colon cancer, where neutralizing PGE synthase and thus inhibiting PGE2 synthesis decreases colon tumor formation in genetically engineered murine models of colon cancer [90]. Further, levels of PGE2 positively correlate with cancer stem cell markers in colorectal cancer patient tumor samples. PGE2 has also been shown to be pro-metastatic, as administration of PGE2 results in increased tumor and liver metastasis in a genetically engineered model of colorectal cancer [91]. Mechanistically, PGE2 has been demonstrated to promote tumor growth through stimulation of angiogenesis and immune suppression in several cancers, including colon cancer [92]. PGE2 generated by tumor cells additionally stimulates myeloid-derived suppressor cells to inhibit natural killer cells, contributing to immune suppression [93]. In addition to the well characterized activity of PGE2 in colon cancer, the deletion of COX or PGE synthase in melanoma cells resulted in tumor rejection in immunocompetent mice [94]. However, in Rag1 knockout (KO) mice, melanoma tumor cells lacking PGE synthase form growing tumors [94]. As Rag1 KO mice are unable to generate mature T and B lymphocytes, this result highlights the relationship between PGE2, immune cells, and tumor progression. We analyze the characterization of PGE2 within this paradigm in regard to its relationship with omega-3 fatty acids and aspirin in the sections entitled “Omega-3 Fatty Acids and Derivatives” and “Inflammation and Cancer: Clinical Applications,” respectively.
Epoxyeicosatrienoic Acids
Epoxyeicosatrienoic acids (EETs) are locally acting lipid signaling molecules with a short half-life and exhibit both autocrine and paracrine activities. EETs are the products of arachidonic acid metabolism by cytochrome P450 enzymes. EETs are then further metabolized by soluble epoxide hydrolase (sEH) into dihydroxyeicosatrienoic acid (DHET). EETs have been vastly studied in various inflammatory diseases. While EETs are known to mediate proliferation, migration, and inflammation in human tissues, their molecular mechanisms in doing so remain poorly characterized [95]. EETs are known to play a role in coronary arteriole dilation [96], stimulate tissue and organ regeneration [97], promote wound healing [98], delay seizure onset [99], and participate in many other disease processes with an inflammatory component [100]. Interestingly, their role in specific inflammatory diseases appears to be somewhat complex. EETs have been shown to be cardioprotective, inhibit pathogenesis of diabetes, and exert renal and neuronal protection. EETs also promote tumor cell proliferation and regulate host antitumor immunity [101, 102]. Thus, their role in cancer continues to be an active area of study. It has been demonstrated that both exogenous systemic and endogenous endothelium-derived EETs promote not only tumor growth and angiogenesis in murine models but also metastasis and tumor dormancy escape [101]. The EETs pro-tumor activity in these models was in part due to increasing VEGF secretion from endothelium in the tumor microenvironment [101]. In fact, the pro-tumorigenic role of EETs has also been demonstrated in human breast tumor tissue. Increased levels of 14,15-EET correlate with greater malignancy potential in breast cancer patients [103]. While the role of EETs in cancer requires further elucidation, modulation of EET levels via sEH has been purposed as a possible new direction in cancer therapy [95, 104]. Specifically, the promotion of EET metabolism by the endogenous over-expression of sEH in transgenic mice has been shown to reduce tumor burden [101]. In summary, the role of EETs in cancer remains an interesting vantage point whereby to view inflammation and cancer, while also providing insight into possible new therapeutic targets.
Omega-3 Fatty Acids and Derivatives
Omega-3 fatty acids are essential dietary polyunsaturated fatty acids that are metabolized in the human body to other essential lipid metabolites, including prostaglandins, thromboxane, and leukotrienes. Omega-3 fatty acids and their derivatives have recently gained widespread public attention for their potential wide-ranging health benefits. Eicosapentaenoic acid (EPA) and docosahexaenoic acid (DHA) are omega-3 fatty acids that are frequently marketed as dietary supplements to promote heart health. The potential role of these fatty acids in cancer pathogenesis is also of interest, as diets rich in omega-3 fatty acids correlate with a reduction in cancer-related deaths [105]. The anti-inflammatory mechanism to activity of omega-3 fatty acids has been primarily attributed to its metabolites, EPA and DHA. These molecules saturate enzymes that classically metabolize arachidonic acid into pro-inflammatory molecules and instead generate lipid autacoids with more anti-inflammatory characteristics [106]. EPA and DHA can additionally activate peroxisome proliferator-activated receptors (PPARs), transcription factors with known anti-inflammatory effects [106], and, interestingly, antitumor activity [107, 108]. Dietary supplementation of omega-3 fatty acids has been associated with decreased risk of colorectal cancer mortality [109]. Of note, chronic inflammation, such as ulcerative colitis, is a risk factor for colorectal cancer pathogenesis [110].
Omega-3 fatty acid supplementation has also been demonstrated to decrease prostate cancer progression in murine models via a macrophage-mediated mechanism [111]. Dietary DHA in these models decreased tumor-associated macrophage viability, as well as pro-inflammatory cytokines and NFκβ-mediated gene expression. Induction of tumor cell apoptosis may mediate, at least in part, the antitumor activity of omega-3 fatty acids [112]. Studies have further indicated that omega-3 fatty acids are capable of inducing apoptosis in a range of solid cancers in vitro, ranging from gastrointestinal origin to neural tissue, and even hematological cancers [112]. While the underlying mechanism remains to be fully elucidated, it appears that omega-3 fatty acids play a role in inducing both the intrinsic and extrinsic apoptosis pathways [112]. In addition to inducing apoptotic programs, omega-3 fatty acids play an anti-tumorigenic role through increasing tumor cell susceptibility to cytotoxic therapies and are being investigated as adjuvant cancer therapies [113]. Omega-3 fatty acid supplementation was also found to enrich tumor cell membranes in unsaturated fatty acids, in turn making the cells more susceptible to destruction by free radicals [113].
An important process in tumor malignancy is epithelial-mesenchymal transition (EMT). This transformation is critical for tumor cell invasion into neighboring tissues and sets the foundation for tumor metastasis. DHA has proven to be efficacious in inhibiting epithelial-mesenchymal transition in colorectal cancer models [114]. The mechanism of DHA’s direct action in halting this process remains relatively unknown. As previously described, PGE2 and COX-2 are characteristically pro-tumorigenic. Recent studies in endometrial cancer suggest that omega-3 fatty acid supplementation exerts its antitumor activity through downregulating COX-2 expression and thus subsequently decreasing endogenous PGE2 levels [115]. This mechanism has also been demonstrated in a colon cancer model, in which EPA and DHA supplementation increased lipoxin A4 (LXA4), an anti-inflammatory endogenous lipid mediator [116]. While the intricate workings of omega-3 fatty acids still required further research, their potential in cancer therapeutics appears promising.
Leukotrienes
Similar to EETs, leukotrienes are another class of eicosanoid metabolites of arachidonic acid generated by members of the lipoxygenase enzyme family. Leukotrienes are traditionally viewed as pro-inflammatory molecules and have been highly studied in inflammatory lung diseases. Similar to the other lipid mediators, their role in inflammation is being actively elucidated. Leukotrienes are mainly synthesized by leukocytes, contributing to both innate and adaptive immunity responses [117]. In acute inflammatory settings, leukotriene B4 (LTB4) increases leukocyte trafficking, as well as pro-inflammatory cytokines such as IL-6 and TNFα. In fact, it has been demonstrated that cellular secretion of LTB4 is a crucial first step in potentiating inflammation-induced tumorigenesis in a lung cancer model [118]. The mechanism of LTB4 tumorigenic contribution was demonstrated to be mediated by signaling through binding of its receptor BLT1, characteristically expressed on peripheral blood leukocytes [118]. LC-MS-MS-based profiling demonstrated an increase in leukotrienes LTC4 and LTE4 correlates with tumor progression in aggressive murine lung cancer models [119]. Interestingly, in these models, resident alveolar macrophages demonstrated high expression of 5-lipoxygenase (5-LOX) and subsequent increases in LTB4, LTC4, and LTD4 secretion, as compared to infiltrating macrophages which did not produce leukotrienes [119]. This study provides insight into leukotrienes’ locally acting inflammatory mechanisms in a cancer setting. Interestingly, the deletion of 5-LOX in a murine lung cancer model stimulates primary tumor growth and liver metastasis through the regulation of T cells [120].
Recent evidence implicating 5-LOX as an inhibitor of tumor growth suggests that leukotrienes produced by this enzyme potentially enact anti-tumorigenic programs in addition to their well-characterized pro-tumorigenic activities. Inhibition of 5-LOX in murine models has been demonstrated to reduce polyp burden in intestinal mucosa, a known mechanistic step in the APC-driven adenoma-carcinoma sequence of colon cancer [121]. In this model, the inhibition of 5-LOX was further accompanied by a decrease in inflammatory infiltrate, including cytokines and immune cells [121]. Further, the inhibition of 5-LOX has been shown to selectively induce apoptosis in prostate cancer cells via decreased expression of c-Myc mRNA [122]. C-Myc is a commonly mutated gene in various cancers, allowing for unregulated cell proliferation. In pancreatic cancer models, 5-LOX knockout mice were shown to have decreased pancreatic lesions, precursors to pancreatic ductal adenocarcinoma [123]. Thus, while further studies on the role of leukotrienes in cancer are needed, it is apparent that their modulation could provide important insight into cancer pathogenesis and potentially open new therapeutic avenues.
Annexin A1
A protein of growing interest in inflammation and cancer is annexin A1 , an anti-inflammatory protein that inhibits acute inflammation by blocking phospholipase A2. This neutralization results in decreased eicosanoid production and inhibits leukocyte adhesion and infiltration. In breast cancer patients, high levels of annexin A1 have been associated with decreased overall survival and poor breast cancer-specific survival [124, 125]. Similarly, lung cancer patients have been shown to have a significant increase in annexin A1 levels in plasma [126]. Further, annexin A1 mRNA and protein levels are increased in lung tumor tissue as compared to adjacent, noncancerous tissue [126]. Thus, annexin A1, an anti-inflammatory protein, may prove to be an important biomarker for tumor progression.
C-Reactive Protein
C-reactive protein (CRP) is released by macrophages and enhances complement recognition and subsequent phagocytosis by macrophages. CRP is clinically used as a biomarker for various inflammatory diseases, including inflammatory bowel disease, pelvic inflammatory disease, arthritis, autoimmunity, and even heart disease. While C-reactive protein’s role in cancer has remained elusive, it has been shown to be a biomarker for various cancers. Elevated CRP levels are validated biomarkers in both Crohn’s disease and ulcerative colitis, both chronic inflammatory diseases of the colon. Elevated levels of CRP have also been associated with an increased risk of colorectal cancer development [127]. Moreover, increased levels of CRP correlate with decreased overall survival and more aggressive tumor recurrence and metastasis in oral squamous cell carcinomas [128]. CRP levels have also been associated with an increased risk of epithelial ovarian carcinoma and breast cancer [129–131]. Increased levels of CRP are correlated with decreased cancer-specific survival in pancreatic cancer patients [132]. While the relationship between CRP and cancer risk and progression remains unclear, research suggests that CRP is largely pro-tumorigenic and could serve as a clinical biomarker for several types of cancer.
Inflammation and Cancer: Clinical Applications
Chronic inflammation is a known risk factor for various cancers. For example, pancreatitis is a known risk factor for pancreatic cancer, ulcerative colitis and Crohn’s disease are known risk factors for colon cancer, and Helicobacter pylori infections are known to increase the risk of stomach cancer. Other associations include cystitis and bladder cancer, Barrett’s esophagus and esophageal carcinoma, and bronchitis and lung cancer [16, 133]. However, the extent to which underlying inflammation contributes to tumor growth remains elusive. Inflammatory reactions appear to have a threshold for which they can either play a pro-tumorigenic role or anti-tumorigenic role. Thus, characterizing the point at which inflammation transitions from facilitating tumor inhibition to promoting disease progression has been the aim of many recent clinical studies. These studies aim to evaluate the “overall inflammatory score” in relation to specific cancers. Further, these clinical studies seek to highlight a potential threshold between acute and chronic inflammation that, when surpassed, could contribute to cancer pathogenesis.
In a recent study, in which the inflammatory score reflected the neutrophil to lymphocyte ratio, an increased neutrophil to lymphocyte ratio is an independent predictive marker or clinical outcomes in head and neck squamous cell carcinoma patients. This increased ratio was also associated with a decrease in overall survival and recurrence-free survival [134]. Another study found men who demonstrated either acute or chronic inflammation in prostate tissue with a negative prostate biopsy have a decreased risk in prostate cancer pathogenesis 2 years post-biopsy. However, 4 years post-biopsy, decreased risk in prostate cancer was found to be positively associated with acute inflammation [135]. In a similar study on prostate cancer, chronic inflammation, defined by the presence of lymphocytes, plasma cells, and macrophages, was associated with lower prostate tumor volume [136]. In a large-scale study evaluating the overall risk of cancer, a combination of CRP levels and leukocyte count was used as an inflammatory score. An increase in the inflammatory score correlated with an increase in prostate, lung, and colorectal cancer risk [137]. A correlation between decreased inflammation and increased cancer survival has also been shown in cervical cancer, in which a decreased platelet to lymphocyte ratio correlates with an increase in overall and disease-free survival in patients [138]. Recent studies further highlight the importance of lymphocyte to monocyte ratio in several cancers. Interestingly, decrease in lymphocyte to monocyte ratio was found to be consistent with decrease in overall survival in colorectal cancer, lung cancer, pancreatic cancer, and Hodgkin’s lymphoma [139]. An additional large-scale, retrospective study demonstrated that a greater ratio of lymphocytes to monocytes resulted in increased overall and disease-free survival in non-small cell lung cancer patients [140].
Thus, while the mechanisms underlying inflammation’s contribution to cancer progression remain an area of ongoing research, it is apparent that inflammation has an intimate connection with tumor pathogenesis. Therefore, it is no surprise that in efforts to prevent cancer progression, widely used anti-inflammatory medications have come into focus as prophylaxis. Aspirin and other nonsteroidal anti-inflammatory drugs (NSAIDs) have been the subject of small- and large-scale cancer patient studies. Beginning in the late 1980s, there has been a growing body of evidence implicating aspirin’s ability to decrease colorectal cancer risk [141, 142]. Although yet to be elucidated, aspirin’s antitumor mechanism appears to be its multifaceted inhibition of inflammation through COX acetylation. Aspirin acetylation of cyclooxygenase inhibits prostaglandin synthesis, thus decreasing the pro-inflammatory and pro-tumorigenic activities of PGE2 as well as decreasing platelet activation. It has been hypothesized that aspirin also inhibits immune cell activation [142]. Aspirin regiments have been associated with decreased risks of epithelial ovarian cancer and gastric cancer [143–145]. Aspirin has also been associated with decreased risk of breast cancer-related death following breast cancer diagnosis [146]. However, retrospective studies assessing aspirin’s anticancer benefits remain controversial. One review concluded that there was no statistically significant correlation between low-dose aspirin use and reduction in overall cancer risk and that aspirin’s chemopreventive activity was limited to colorectal cancer risk [147]. Similarly, other nonsteroidal anti-inflammatory drugs have proved to decrease risk of colorectal and prostate cancer [148–150]. The mechanism of NSAIDs within the tumor microenvironment has also been characterized as their ability to inhibit tumorigenic potential and tumor immune tolerance, as well as to enhance immunosurveillance [110]. Interestingly, this activity was found to be mediated in part through the reduction of PGE2 [110]. However, anti-inflammatories such as aspirin and COX-2 inhibitors have faced therapeutic challenges in the clinic, as they cause severe side effects such as kidney toxicity, stomach bleeding, and heart complications. Thus, more efforts to characterize the anti-tumorigenic mechanisms of aspirin and other NSAIDS are needed to develop novel viable clinical therapeutics that harness their broad anticancer activity.
Summary
Extensive evidence has established a link between inflammation and cancer, from the first observation of inflammation in tumors hundreds of years ago to current studies that have characterized the malleable and complex roles inflammatory cells and their mediators play in cancer progression. Experimental and clinical studies provide mechanisms that can be harnessed for future cancer therapy, with the aim to halt pro-tumorigenic inflammatory processes or harness the anti-tumorigenic pathways embedded in the human immune system. Many of the lipid mediators discussed above have potential as cancer biomarkers and exhibit a dual role in tumorigenesis, highlighting their diverse biological activity in various tumor microenvironments (Fig. 7.2). The frame through which to view inflammation and cancer is not one of strictly “pro-tumor” or “antitumor”; rather there exists a multitude of environmental influences that ultimately direct the role inflammation plays within the context of cancer. Future studies will be required to elucidate both the pro- and anti-tumorigenic roles inflammation plays, as well as to provide novel therapies that harness the immune system to inhibit or prevent cancer.


Fig. 7.2
Biomarkers in the tumor microenvironment can simultaneously promote (red) and inhibit (blue) tumor pathogenesis, highlighting the fluidity of the role of inflammation in cancer
References
1.
Trinchieri G. Cancer and inflammation: an old intuition with rapidly evolving new concepts. Annu Rev Immunol. 2012;30:677–706. doi:10.1146/annurev-immunol-020711-075008.PubMed
3.
Virchow R. Cellular pathology as based upon physiological and pathological histology: twenty lectures delivered in the pathological Institute of Berlin during the months of February, march, and April, 1858. New York: Robert M. De Witt; 1860.
4.
Coley WB II. Contribution to the knowledge of sarcoma. Ann Surg. 1891;14:199–220.PubMedPubMedCentral
5.
Dvorak HF. Tumors: wounds that do not heal. Similarities between tumor stroma generation and wound healing. N Engl J Med. 1986;315:1650–9. doi:10.1056/NEJM198612253152606.PubMed
6.
Coussens LM, et al. Inflammatory mast cells up-regulate angiogenesis during squamous epithelial carcinogenesis. Genes Dev. 1999;13:1382–97.PubMedPubMedCentral
7.
Coussens LM, Tinkle CL, Hanahan D, Werb Z. MMP-9 supplied by bone marrow-derived cells contributes to skin carcinogenesis. Cell. 2000;103:481–90.PubMedPubMedCentral
8.
Di Carlo E, et al. The intriguing role of polymorphonuclear neutrophils in antitumor reactions. Blood. 2001;97:339–45.PubMed
9.
Kitamura T, Qian BZ, Pollard JW. Immune cell promotion of metastasis. Nat Rev Immunol. 2015;15:73–86. doi:10.1038/nri3789.PubMedPubMedCentral
10.
Hanahan D, Weinberg RA. Hallmarks of cancer: the next generation. Cell. 2011;144:646–74. doi:10.1016/j.cell.2011.02.013.PubMed
11.
Mantovani A, Allavena P, Sica A, Balkwill F. Cancer-related inflammation. Nature. 2008;454:436–44. doi:10.1038/nature07205.PubMed
12.
Mantovani A, Caprioli V, Gritti P, Spreafico F. Human mature macrophages mediate antibody-dependent cellular cytotoxicity on tumour cells. Transplantation. 1977;24:291–3.PubMed
13.
Mantovani A, Bottazzi B, Colotta F, Sozzani S, Ruco L. The origin and function of tumor-associated macrophages. Immunol Today. 1992;13:265–70. doi:10.1016/0167-5699(92)90008-U.PubMed
14.
Balkwill FR, Ward BG, Moodie E, Fiers W. Therapeutic potential of tumor necrosis factor-alpha and gamma-interferon in experimental human ovarian cancer. Cancer Res. 1987;47:4755–8.PubMed
15.
Balkwill F. Tumor necrosis factor or tumor promoting factor? Cytokine Growth Factor Rev. 2002;13:135–41.PubMed
16.
Coussens LM, Werb Z. Inflammation and cancer. Nature. 2002;420:860–7. doi:10.1038/nature01322.PubMedCentral
17.
Pikarsky E, et al. NF-kappaB functions as a tumour promoter in inflammation-associated cancer. Nature. 2004;431:461–6. doi:10.1038/nature02924.PubMed
18.
Luo JL, Maeda S, Hsu LC, Yagita H, Karin M. Inhibition of NF-kappaB in cancer cells converts inflammation- induced tumor growth mediated by TNFalpha to TRAIL-mediated tumor regression. Cancer Cell. 2004;6:297–305. doi:10.1016/j.ccr.2004.08.012.PubMed
19.
Elwood PC, et al. Aspirin in the treatment of cancer: reductions in metastatic spread and in mortality: a systematic review and meta-analyses of published studies. PLoS One. 2016;11:e0152402. doi:10.1371/journal.pone.0152402.PubMedPubMedCentral
20.
Qian BZ, Pollard JW. Macrophage diversity enhances tumor progression and metastasis. Cell. 2010;141:39–51. doi:10.1016/j.cell.2010.03.014.PubMedPubMedCentral
21.
Heuff G, et al. Enhanced tumour growth in the rat liver after selective elimination of Kupffer cells. Cancer Immunol Immunother. 1993;37:125–30.PubMed
22.
Oosterling SJ, et al. Macrophages direct tumour histology and clinical outcome in a colon cancer model. J Pathol. 2005;207:147–55. doi:10.1002/path.1830.PubMed
23.
Weber C, et al. Macrophage infiltration and alternative activation during wound healing promote MEK1-induced skin carcinogenesis. Cancer Res. 2016;76:805–17. doi:10.1158/0008-5472.CAN-14-3676.PubMedPubMedCentral
24.
Jaiswal S, et al. CD47 is upregulated on circulating hematopoietic stem cells and leukemia cells to avoid phagocytosis. Cell. 2009;138:271–85. doi:10.1016/j.cell.2009.05.046.PubMedPubMedCentral
25.
Gabrusiewicz K, et al. Glioblastoma-infiltrated innate immune cells resemble M0 macrophage phenotype. JCI Insight. 2016;1:e85841. doi:10.1172/jci.insight.85841.PubMedPubMedCentral
26.
Chow A, et al. Macrophage immunomodulation by breast cancer-derived exosomes requires toll-like receptor 2-mediated activation of NF-kappaB. Sci Rep. 2014;4:5750. doi:10.1038/srep05750.PubMedPubMedCentral
27.
Kitamura T, et al. CCL2-induced chemokine cascade promotes breast cancer metastasis by enhancing retention of metastasis-associated macrophages. J Exp Med. 2015;212:1043–59. doi:10.1084/jem.20141836.PubMedPubMedCentral
28.
Chavez-Galan L, Olleros ML, Vesin D, Garcia I. Much more than M1 and M2 macrophages, there are also CD169(+) and TCR(+) macrophages. Front Immunol. 2015;6:263. doi:10.3389/fimmu.2015.00263.PubMedPubMedCentral
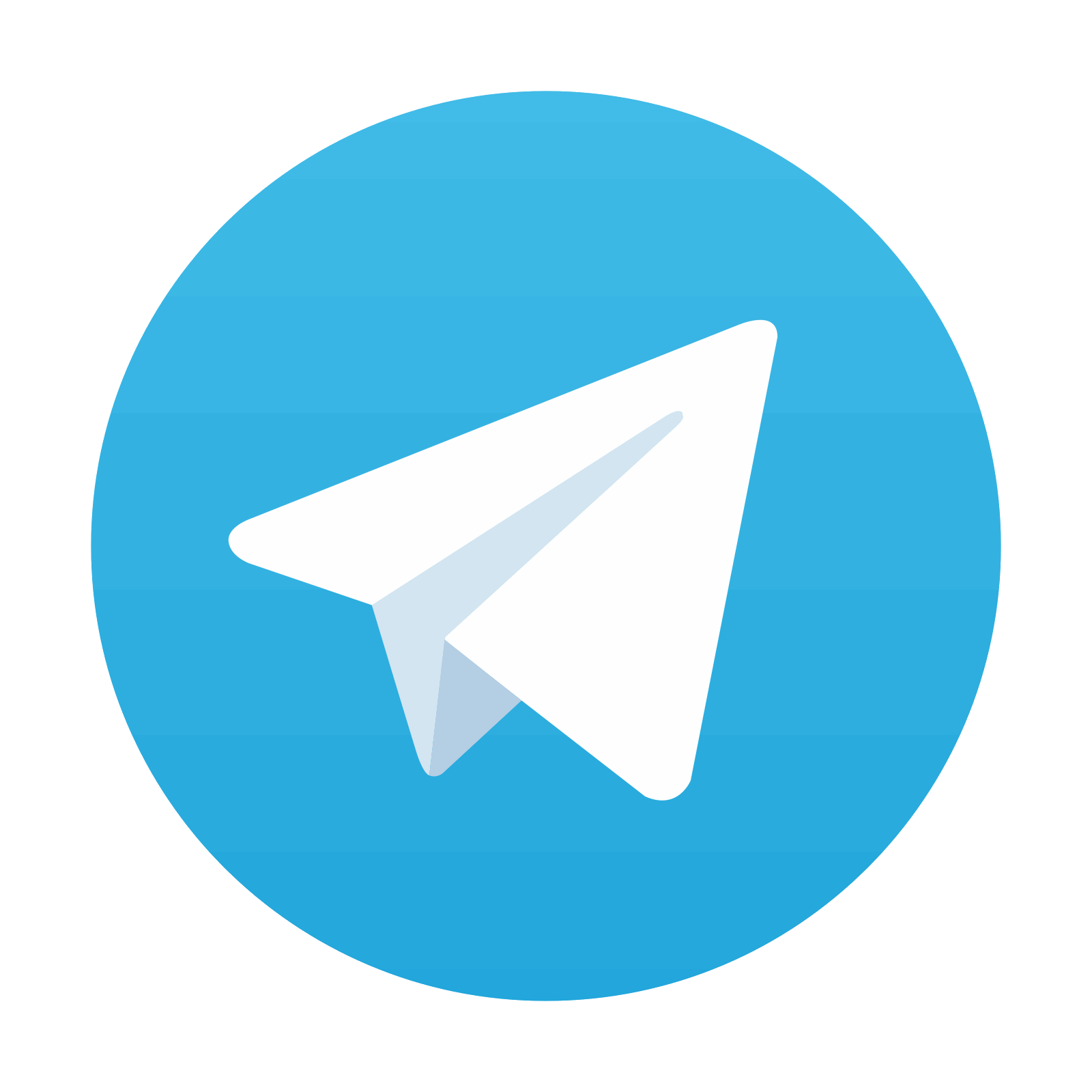
Stay updated, free articles. Join our Telegram channel

Full access? Get Clinical Tree
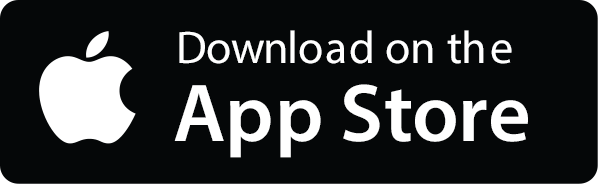
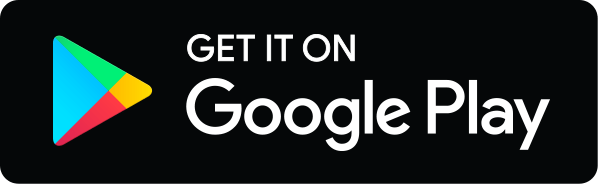