Coagulase-positive: S. aureus
Coagulase-negative: S. epidermidis, S. hominis, S. saprophyticus, S. haemolyticus, etc.
Group (β-hemolytic) Streptococci (S. pyogenes, S. agalactiae, Groups C, F, and G streptococci)
Peptostreptococcus spp.
Lactobacillus spp.
Listeria monocytogenes
Propionibacterium spp. (P. acnes)
Clostridium spp. (C. perfringens, C. difficile, C. tetani)
Streptomyces spp.
Erysipelothrix rhusiopathiae
Nocardia spp. (N. asteroides)
Veillonella spp. (V. parvula)
Moraxella catarrhalis
Citrobacter spp. (C. freundii, C. koseri)
Enterobacter spp. (E. cloacae, E. aerogenes)
Escherichia coli
Klebsiella pneumoniae
Pasteurella multocida
Vibrio cholerae
Alcaligenes spp.
Burkholderia cepacia
Morganella morganii
Proteus spp. (P. mirabilis, P. vulgaris)
Pseudomonas spp. (P. aeruginosa, P. putida, P. fluorescens)
Salmonella spp. (S. typhi, S. paratyphi, S. enteritidis, S. typhimurium)
Serratia marcescens
Shigella spp. (S. dysenteriae, S. sonnei)
Stenotrophomonas maltophilia
Brucella spp.
Bordetella spp.
Campylobacter jejuni
Francisella tularensis
Helicobacter pylori
Legionella spp.
Besides providing a clue regarding the potential infecting organism, the Gram stain also helps to determine the presence of bacteria in biological specimens obtained from normally sterile body fluids (e.g., CSF, pleural fluid, synovial fluid, and urine directly from the bladder) and from specimens where infection is suspected (e.g., abscess fluid, wound swabs, sputum, and tissue); the number or relative quantity of infecting bacteria; the presence of WBCs; and the adequacy of the submitted specimen (e.g., large numbers of epithelial cells in a sputum or urine sample may signify contamination).1,2,4,6,7,9,11
Culture and Identification
The results from the Gram stain provide preliminary information regarding the potential infecting bacteria. In order for the bacteria to be definitively identified, the clinical specimen is also processed to facilitate bacterial growth in culture and then observed for growth characteristics (e.g., type of media, aerobic versus anaerobic, and shape and color of colonies) and reactions to biochemical testing. Under normal circumstances, the results of bacterial culture are typically available within 24–48 hours of specimen setup and processing.
In order for a bacteria to be grown successfully in culture, the specific nutritional and environmental growth requirements of the bacteria must be taken into consideration.2–4,8,12,13 There are several clinical microbiology textbooks and reference manuals available that can assist the microbiology laboratory with the selection of appropriate culture media and environmental conditions to facilitate the optimal growth of bacteria based on specimen type and suspected bacteria.2–4,8,12,13
Several types of primary culture media are available that enhance or optimize bacterial growth including nutritive media (blood or chocolate agar), differential media, selective media, and supplemental broth.1–3,8,12 The most commonly utilized bacterial growth media are listed in Table 17-3. Blood and chocolate agar plates are nutritive or enrichment media because they support the growth of many different types of aerobic and anaerobic bacteria. Blood agar is also considered to be differential media because it can distinguish between organisms based on certain growth characteristics, such as between the different streptococci based on hemolysis patterns. MacConkey (Mac), eosin methylene blue (EMB), colistin-nalidixic acid (CNA), and phenylethyl alcohol (PEA) agar plates are all selective media because they preferentially support the growth of specific organisms from a sample (e.g., Gram-negative or Gram-positive bacteria) through the use of antimicrobials, dyes, or alcohol incorporated into their media. Trypticase soy broth (TSB) and thioglycollate broth are considered supplemental media since they are used for subculturing bacteria detected on agar plates, or as back-up cultures to agar plates for the detection of small quantities of bacteria in biological specimens.
CNA = colistin-nalidixic acid; EMB = eosin methylene blue; Mac = MacConkey; PEA = phenylethyl alcohol; SBA = sheep blood agar; TSB = trypticase soy broth.
Once a clinical specimen is processed using the appropriate media, the plates must be incubated in the appropriate environment to support bacterial growth. The environmental factors that should be controlled during incubation include oxygen or carbon dioxide content, temperature, pH, and moisture content of the medium and atmosphere.1,12 The oxygen requirements for growth differ among organisms. Strict aerobic bacteria, such as Pseudomonas aeruginosa and Staphylococcus aureus, grow best in ambient air containing 21% oxygen and a small amount of carbon dioxide.1 Strict anaerobes, such as Bacteroides spp., are unable to grow in an oxygen-containing environment and require a controlled environment containing 5% to 10% carbon dioxide for optimal growth. Facultative anaerobes, such as Escherichia coli and some streptococci, can grow in the presence or absence of oxygen. Overall, most clinically-relevant bacteria grow best at 35°C to 37°C (the temperature of the human body), a pH of 6.5 to 7.5, and in an atmosphere rich in moisture, which is the reason why agar plates are sealed (to trap moisture).12
Bacteria grown successfully in culture appear as colonies on the agar plates. Identification of the bacteria are based on evaluation of colony characteristics (size, pigmentation, shape, and surface appearance); the assessment of culture media and environmental conditions that supported the growth of the bacteria; the changes that occurred to the culture media as a result of bacterial growth; the aroma of the bacteria; the Gram stain result of individual colonies; and metabolic properties.3,12 Biochemical tests are either enzyme-based, where the presence of a specific enzyme is measured (e.g., catalase, oxidase, indole, or urease tests), or based on the presence and measurement of metabolic pathways or byproducts (e.g., oxidative and fermentation tests or amino acid degradation).3,12 Examples of biochemical tests include the presence of catalase in the organism or the ability of a bacteria to ferment glucose. Most biochemical tests are performed using manual or automated commercial identification systems.12,14,15 Some of the commercial identification systems consist of multicompartment biochemical tests in a single microtiter tray so that several biochemical tests can be performed simultaneously.12,14,15 The information derived from the macroscopic examination of the bacteria and the results of biochemical tests are then combined to determine the specific identity of the bacteria.
Colonization, Contamination, or Infection
The growth of an organism from a submitted biologic specimen does not always indicate the presence of infection; it may represent the presence of bacterial contamination or colonization.9,11 Table 17-4 lists the anatomic sites, fluids, and tissues of the human body that are sterile and include the bloodstream, the CSF, internal organs and tissues, bone, synovial fluid, peritoneal fluid, pleural fluid, pericardial fluid, and urine taken directly from the bladder or kidney. Other body sites, particularly those with a connection to the outside environment, have microorganisms called normal flora that naturally colonize their surfaces. Normal bacterial flora can be found on the skin and in the respiratory, gastrointestinal, and genitourinary tracts; the bacteria that typically colonize these body sites are listed in Table 17-5.11,16 Typically, normal flora are harmless bacteria that rarely cause infection. They are often located in the same areas of the body as pathogenic bacteria and are thought to be protective by inhibiting the growth of pathogenic organisms through competition for nutrients and stimulating the production of cross-protective antibodies.11,16 However, normal flora bacteria may potentially become pathogenic and cause infection in patients with suppressed immune systems or after translocation to normally sterile body sites and tissues during trauma, intravascular line insertion, or surgical procedures, especially when the skin is not adequately cleansed in the latter situations. In addition, pathogenic bacteria may colonize body sites when they are present but do not invade host tissue or cause the signs and symptoms of infection that are listed in Table 17-6.
TABLE 17-4. Normally Sterile Body Sites |
Bloodstream |
CSF |
Pericardial fluid |
Pleural fluid |
Peritoneal fluid |
Synovial fluid |
Bone |
Urine (directly from the bladder or kidney) |
CSF = cerebrospinal fluid.
CRP = C-reactive protein; CT = computed tomography; ESR = erythrocyte sedimentation rate; MRI = magnetic resonance imaging; UTI = urinary tract infection; WBC = white blood cell.
Contamination occurs when an organism is accidentally introduced into a biologic specimen during specimen collection, transport, or processing. Bacteria that cause contamination typically originate from the skin of the patient (especially if not cleansed adequately before specimen acquisition), the clinician, or the laboratory technician, but may also come from the environment. A common biologic specimen contaminant is Staphylococcus epidermidis, which is an organism that can normally colonize the skin. In addition, the presence of normal vaginal or perirectal flora in the urine culture of a patient without evidence of a urinary tract infection (UTI) (absence of symptoms or WBCs) may also be indicative of contamination.
Infection occurs when an organism invades and damages host tissues eliciting a host response and symptoms consistent with an infectious process. When determining the presence of infection in an individual patient, several factors should be considered such as the clinical condition of the patient (e.g., fever and purulent discharge), the presence of laboratory signs of infection (e.g., high WBC count), the results of microbiologic stains and cultures, and the results from radiographic tests.9 Table 17-6 describes some of the local and systemic clinical signs and symptoms, laboratory findings, and radiographic findings that may be present in a patient with infection. The exact clinical, laboratory, and radiographic signs of infection vary based on the site of infection, the age of the patient, and the severity of illness of the patient. For example, a patient with pneumonia may have a fever, productive cough, shortness of breath, tachypnea, leukocytosis, and an infiltrate on chest x-ray, while a patient with a lower UTI will have symptoms such as urinary frequency, urgency, and dysuria. In addition, the typical signs and symptoms of infection may not be present in the elderly or in patients who are immunocompromised (e.g., neutropenic patients and patients with acquired immunodeficiency syndrome [AIDS]).
The diagnosis of infection is usually suspected in a patient with a positive culture accompanied by clinical, laboratory, and radiographic findings suggestive of infection. In clinical practice, there are several situations that will warrant a thorough investigation to determine if the patient with a positive culture from a biologic specimen is truly infected. Since false-positive cultures can be associated with the use of additional laboratory tests, radiographic tests, unnecessary antibiotics, increased length of hospitalization and patient costs, every positive culture should warrant an evaluation for clinical significance.17 Certain bacteria have a propensity to commonly cause infection in particular body sites and fluids, as demonstrated in Table 17-7.4,16,18,19 This information can help the clinician determine if the bacteria isolated in the culture is a commonly encountered pathogen at the particular site of infection.16,18 For instance, the growth of Streptococcus pneumoniae from the sputum of a patient with signs and symptoms of community-acquired pneumonia (CAP) is a significant finding since S. pneumoniae is the most common cause of CAP and the patient is exhibiting symptoms of pneumonia. However, the growth of Staphylococcus epidermidis from a blood or wound culture from an asymptomatic patient should be evaluated for clinical significance since it may represent contamination of the submitted specimen.11 The information in Table 17-7 regarding the most common causative organism by infection site can also be used to select empiric antibiotic therapy before culture results are available by guiding the selection of an antibiotic regimen with activity against the most common causative bacteria at the suspected site of infection, as illustrated in Minicase 1.
MDR = multidrug resistant; MRSA = methicillin-resistant Staphylococcus aureus; MSSA = methicillin-susceptible Staphylococcus aureus.
Using Lab Test Results to Guide the Choice of an Empiric Antibiotic Regimen for Hospital-Acquired Pneumonia
MARIE A., A 68-YEAR-OLD FEMALE, was admitted to the University Hospital 4 days ago for management of a right cerebral vascular accident (CVA). Prior to this admission, she had been living at home with her husband and had been previously healthy without recent hospitalizations or antibiotic therapy within the past few years. Marie A. initially required ICU admission for management of her CVA and was recently transferred to the medical floor after stabilization. She continues to have L-sided hemiparesis, and has been deemed to be an aspiration risk by physical therapy/occupational therapy. On hospital day 4, Marie A. developed a temperature of 102.3°F, chills, tachypnea, a productive cough, and shortness of breath requiring supplemental oxygen via nasal cannula. Her physical exam is significant for an increased respiratory rate of 24 breaths/min and decreased breath sounds in the right middle lobe on lung exam. Her laboratory results reveal a total WBC count of 18,000 cells/mm3 (4800–10,800 cells/mm3) with 70% neutrophils (45% to 73%), 19% bands (3% to 5%), 7% lymphs (20% to 40%), and 4% monos (3% to 8%). Her chest x-ray displays right middle lobe consolidation consistent with pneumonia. The differential diagnosis includes bacterial pneumonia, so an expectorated sputum sample is obtained for Gram stain and culture. The Gram stain reveals >25 WBC/hpf, <10 epi/hpf, and many Gram-negative rods. The physician taking care of Marie A. asks you to recommend empiric antibiotic therapy to treat her pneumonia before the final culture results are available.
Question: What is the most likely causative organism of Marie A.’s pneumonia, and which empiric antibiotic therapy would you choose based on the Gram stain results?
Discussion: Marie A. most likely has early-onset (within 4 days of hospitalization) hospital-acquired pneumonia (HAP), where the most common causative organisms (Table 17-7) in early-onset HAP include Streptococcus pneumoniae; Haemophilus influenzae; Gram-negative bacteria such as Klebsiella pneumoniae, Escherichia coli, Enterobacter spp., Serratia marcescens, Proteus spp.; Staphylococcus aureus (methicillin-susceptible Staphylococcus aureus [MSSA]); and atypical bacteria such as Legionella pneumophila (especially in patients with diabetes mellitus, underlying lung disease, renal failure, or suppressed immune systems). Based on the Gram stain results demonstrating the presence of Gram-negative rods (Table 17-2), Marie A. most likely has HAP due to Klebsiella pneumoniae, Escherichia coli, Enterobacter spp., Serratia marcescens, or Proteus spp., which is not unexpected since Gram-negative bacteria are the most common cause of HAP overall. Based on the most recent Infectious Diseases Society of America (IDSA) guidelines for the management of HAP, Marie A. should receive empiric therapy with either ceftriaxone, ertapenem, or a fluoroquinolone (levofloxacin, ciprofloxacin, or moxifloxacin) based on her lack of risk factors for a multidrug-resistant (MDR) organism.19 The antibiotic regimen can be modified to more directed therapy, if possible, once the results of the culture and susceptibility are available.
Occasionally, patients with infection may have negative cultures, particularly in the setting of previous antibiotic use, improper culture collection methods, or the submission of inadequate specimens. In this setting, the clinical condition of the patient may establish the presence of infection despite negative cultures, where the suspected site of infection should help guide antibiotic therapy based on most likely causative organisms that cause infection at that site.9
Antimicrobial Susceptibility Testing
Once an organism has been cultured from a biologic specimen, further testing is performed in the microbiology laboratory to determine the antibiotic susceptibility of the infecting organism to help direct and streamline antimicrobial therapy. Due to the continual emergence of resistance in many organisms, bacterial susceptibility testing is imperative for determining the antimicrobial agents that could potentially be used for the treatment of the patient’s infection. There are a number of different methods that can be utilized to determine the antibiotic susceptibility of a particular organism, and these methods can either (1) directly measure the activity of an antibiotic against the organism or (2) detect the presence of a specific resistance mechanism in the organism, as described in Table 17-8.9,11,20–25 Microbiology labs often utilize several different methods for susceptibility testing in order to accurately determine the activity of antibiotics against many different types of bacteria (e.g., aerobic, anaerobic, and fastidious). The Clinical and Laboratory Standards Institute (CLSI) continuously updates and publishes standards and guidelines for the susceptibility testing of aerobic and anaerobic bacteria to assist microbiology labs in determining the specific antibiotics and test methods that should be utilized based on the particular organism or the particular clinical situation/infection.3,20,21,26–28
Etest® = epsilometer test; HLAR = high-level aminoglycoside resistance; MBC = minimum bactericidal concentration; MRSA = methicillin-resistant Staphylococcus aureus; NA = nucleic acid; SBT = serum bactericidal test; SGE = spiral gradient endpoint; VRE = vancomycin-resistant enterococci.
Methods That Directly Measure Antibiotic Activity
There are a number of different tests that measure the activity of an antibiotic against a particular organism. Quantitative tests measure the exact concentration of an antibiotic necessary for inhibiting the growth of the bacteria, and qualitative tests measure the comparative activity of antibiotics against the organism. It is important to note that the test results and interpretation of susceptibility from each of these methods will be reported by the laboratory based on the methodology that was utilized. The advantages and disadvantages of the different antimicrobial susceptibility testing methods are listed in Table 17-9.9,11,20,25,29,30
Etest® = epsilometer test; MBC = minimum bactericidal concentration; MIC = minimum inhibitory concentration; SGE = spiral gradient endpoint.
Dilution Methods (Macrodilution and Microdilution)
Several dilution methods exist that measure the activity of an antibiotic against a particular organism. Both broth dilution and agar dilution methods quantitatively measure the in vitro activity of antibiotics against a particular organism. Broth dilution can be performed using macrodilution or microdilution, where the main differences between the methods include the volume of broth utilized, the number of antibiotics that can be simultaneously tested, and the manner in which the test results are generated and reported. The agar dilution method differs in that it is performed using solid growth media.
Broth macrodilution. Broth macrodilution, or the tube-dilution method, is one of the oldest methods of antimicrobial susceptibility testing and is often considered the gold standard. This method is performed in test tubes in which twofold serial dilutions of the antibiotic being tested (with concentrations tested based on clinically-achievable serum or site concentrations of the antibiotic in mcg/mL) are placed in a liquid growth media (1 mL of broth or greater) to which a standard inoculum (5 × 105 cfu/mL) of the infecting bacteria is added.20,25–27,29 The tests tubes are incubated for 16–24 hours at 35°C and then examined macroscopically for the presence of turbidity or cloudiness, which is an indication of bacterial growth.11,20,25,27,29 The test tube containing the lowest antibiotic concentration that completely inhibits visible growth (the broth in the tube appears clear to the unaided eye) represents the minimum inhibitory concentration (MIC) in mcg/mL (Figure 17-1).20, 25,27,29
FIGURE 17-1. Broth macrodilution susceptibility testing for MIC and MBC. (Reprinted, with permission, from reference 9.)
The CLSI has established interpretive criteria for the MIC results of each antibiotic against each bacteria as Susceptible (S), Intermediate (I), and Resistant (R). The exact MICs that separate or define these three categories for an antibiotic are known as their MIC breakpoints.20,21,25 Minimum inhibitory concentrations have been categorized as S, I, and R to help predict the probable response of a patient’s infection to a particular antibiotic.9,21,25 Bacteria that are categorized as “susceptible” to a given antibiotic will, most likely, be eradicated during treatment of the infection since concentrations of the antibiotic represented by the MIC are easily achieved using standard doses of the antibiotic. “Intermediately” susceptible bacteria display higher MICs, where successful treatment may be achieved if higher than normal doses of an antibiotic are utilized or the antibiotic concentrates at the site of infection.25 In clinical practice, antibiotics displaying intermediate susceptibility against an organism are rarely used for treatment of the infection since clinical response is unpredictable. One of the only clinical scenarios where intermediately-susceptible antibiotics are used is when the organism displays resistance to all other agents tested. Lastly, organisms that are “resistant” to an antibiotic display extremely high MICs that exceed the normal achievable serum concentrations of the antibiotic, even if maximal doses are utilized, so that a poor clinical response would be expected.
Minimum inhibitory concentration breakpoints for each antibiotic against each bacteria are based on a number of factors including achievable serum concentrations of the antibiotic after normal dosing; the inherent susceptibility of the organism to the antibiotic; the site of infection and ability of the antibiotic to obtain adequate concentrations at that site; pharmacodynamic analysis with Monte Carlo simulations to predict efficacy; and the results of clinical efficacy trials of the antibiotic against infections due to the specific organism.20,21,26,27,30 The safe and effective dose of each antibiotic is typically determined using pharmacokinetic, safety, and efficacy data gathered during preclinical stages of drug development. Since each antibiotic has its own unique pharmacokinetic profile and recommended dosage range, it is not surprising that each antibiotic achieves different serum concentrations after standard dosing. For example, intravenously-administered piperacillin/tazobactam achieves much higher serum concentrations and area under the serum concentration time curve (AUC) than intravenously-administered levofloxacin. Therefore, the MIC breakpoint for susceptibility for piperacillin/tazobactam against Enterobacteriaceae is higher (≤16 mcg/mL) than levofloxacin (≤2 mcg/mL). Another factor that is considered in the determination of MIC breakpoints is the inherent in vitro activity of the antibiotic against the organism. Some antibiotics are inherently more active against an organism than others; this is reflected by a lower MIC required to inhibit bacterial growth. The site of infection should also be considered, as this may predict the potential usefulness of the antibiotic depending on its ability to achieve adequate concentrations at the site of infection. An antibiotic might be very active against a particular organism in vitro, but may be ineffective in vivo due to poor penetration to the site of infection. In fact, there are a few clinical situations where the site of infection is directly incorporated into the MIC interpretation of an antibiotic, such as in the case of meningitis due to Streptococcus pneumoniae, where the interpretation of ceftriaxone and penicillin susceptibility should be determined utilizing meningitis breakpoints of both drugs. Monte Carlo analysis, using population pharmacokinetic data of the antibiotic and MIC distribution data from susceptibility studies of an organism, are also performed in MIC breakpoint determination to evaluate the percentage of time the particular antibiotic being evaluated will achieve adequate serum concentrations or particular pharmacodynamic indices for the treatment of that organism in a simulated population. Lastly, the results from clinical trials evaluating the efficacy of an individual antibiotic are also considered in MIC breakpoint determination where a correlation is made between the individual MIC value of the infecting organism and clinical efficacy or failure (e.g., what was the MIC of the organisms associated with clinical failure of the antibiotic?). In general, it is the responsibility of the clinician to determine if a drug listed as “susceptible” from an individual isolate susceptibility report is useful for the treatment of a particular infection based on the pharmacokinetic parameters (site penetration) and clinical efficacy studies of the antibiotic for that infection type.
An additional step can be added to the broth macrodilution test to determine the actual antibiotic concentration that kills 99.9% of the bacterial inoculum, which is also known as the minimum bactericidal concentration (MBC).20,22,24 Samples from all of the test tubes from the original broth macrodilution test that did not exhibit visible growth are subcultured on agar plates and incubated at 35°C for 18–24 hours (Figure 17-1).9,22 The plate representing the lowest antibiotic concentration that does not support the growth of any bacterial colonies is defined as the MBC. Because a higher concentration of an antibiotic may be necessary to kill the organism rather than just inhibit its growth, the MIC is always equal to or lower than the MBC. The determination of the MBC is not routinely performed in clinical practice, and is only considered useful in rare clinical circumstances such as in suspected treatment failure during the treatment of severe or life-threatening infections including endocarditis, meningitis, osteomyelitis, or sepsis in immunocompromised patients.9,20,22–24
Broth macrodilution is useful because an exact MIC (and, if needed, an MBC) of the infecting organism can be derived. The results of broth macrodilution are reported as the MIC of the antibiotic against the infecting organism with its corresponding interpretive category (S, I, and R). However, broth macrodilution is rarely utilized in microbiology laboratories since the methodology is resource and labor intensive, making it impractical for everyday use.
Broth microdilution. Broth microdilution susceptibility testing was developed to overcome some of the limitations of the broth macrodilution method and has become the most commonly used method for susceptibility testing of bacteria in microbiology labs.11,20,25,27,29 Instead of utilizing standard test tubes with twofold serial dilutions of antibiotics, this method utilizes manually- or commercially-prepared disposable microtiter cassettes or trays containing up to 96 wells that can simultaneously test the susceptibility of up to 12 antibiotics depending on the product used.11,20,25,27,29 Several examples of microtiter trays are shown in Figures 17-2 (a) and 17-2 (b). The wells in the broth microdilution trays contain a smaller volume of broth (0.05–0.1 mL) to support bacterial growth than broth macrodilution (1.0 mL or more). The microtiter tray is inoculated with a standardized inoculum of the infecting organism and incubated for 16–20 hours. The tray is then examined for bacterial growth by direct visualization utilizing light boxes or reflecting mirrors, or by automated, computer-assisted readers. The MIC represents the microdilution well containing the lowest antibiotic concentration that completely inhibits visible bacterial growth (e.g., did not produce turbidity). A number of companies commercially supply broth microdilution panels that contain broth with appropriate antibiotic concentrations according to guidelines for conventional broth dilution methods. Depending on the product or system, the results can either be read manually/semiautomated or automated. Some examples of the manual/semiautomated systems include BBL Sceptor (BD Microbiology Systems; no longer available), Sensititre Vizion® System (Trek Diagnostics Systems, Inc.), and MicroScan autoSCAN®-4 (Siemens Healthcare Diagnostics, Inc.). Examples of the automated systems include Vitek®-2 (bioMérieux Diagnostics, Inc.), MicroScan WalkAway® Plus System (Siemens Healthcare Diagnostics, Inc.), Sensititre ARIS® 2X and AIM® (Trek Diagnostics Systems, Inc.), and the Phoenix™ Automated Microbiology System (BD Microbiology Systems). The automated systems have also been engineered to aid with bacterial identification, and are able to provide more rapid susceptibility results (within 8 hours) due to shortened incubation times.30
FIGURE 17-2. (a). A broth microdilution susceptibility panel containing 98 reagent wells and a disposable tray inoculator. (Reprinted, with permission, from reference 25.) (b). Example of microtiter cassette used in automated systems that test for bacterial susceptibilities to various antimicrobials. (Reprinted, with permission, Vitek 2 systems card, bioMérieux, 2008.)
Because of the size constraints of microtiter cassettes, only a limited number of antibiotics and concentrations can be incorporated into the trays. Typically, drugs that have inherent activity against the class of bacteria being tested (e.g., Gram-positive versus Gram-negative) are included in the trays. For example, when determining the susceptibility of Enterobacteriaceae, it is impractical to include antibiotics in the microtiter trays that do not have activity against these organisms, such as penicillin, nafcillin, or vancomycin. The same holds true for susceptibility testing of Gram-positive organisms, where it would be impractical to test the susceptibility of piperacillin or ceftazidime against Staphylococcus aureus because these agents have limited antistaphylococcal activity. In addition, the trays are not large enough to incorporate the full range of antibiotic concentrations usually tested using broth macrodilution. Therefore, the concentrations incorporated into the wells for each antibiotic often reflect the CLSI interpretive category breakpoints of S, I, and R for the particular group of organisms. Most microbiology laboratories utilize CLSI guidelines and standards to guide appropriate testing and reporting of antimicrobial susceptibilities.
The test results of broth microdilution occasionally include an exact MIC but more often are expressed as an MIC range because of the limited antibiotic concentrations tested for each antibiotic. For example, if bacterial growth is not detected in the lowest concentration tested of a particular antibiotic using broth microdilution, the MIC would be reported as less than or equal to that concentration tested. The MIC could be much lower, but the exact MIC could not be determined because lower concentrations of the antibiotic were not tested due to the size constraints of the cassettes. As in broth macrodilution, the test results for broth microdilution are often reported to the clinician as the MIC (or MIC range) of the antibiotic against the infecting bacteria with its corresponding CLSI interpretive category (S, I, and R).
The advantages of broth microdilution include the ability to test the susceptibility of multiple antibiotics simultaneously; ease of use when commercially-prepared microtiter trays are utilized; rapid results with the automated methods; and decreased cost and labor.20,25,27,30 The disadvantages of broth microdilution include the lack of flexibility of antibiotics available in commercially-prepared microtiter cassettes; the limitation on the number of concentrations that can be tested for each antibiotic due to size constraints of the trays; and the reporting of an MIC range rather than the true MIC against an infecting organism.20,25,30
Agar dilution. Agar dilution is another quantitative susceptibility testing method that utilizes twofold serial dilutions of an antibiotic incorporated into agar growth medium, which is then placed into individual Petri dishes.20,25,29,30 The surface of each plate is inoculated with a droplet of standardized bacterial suspension (1 × 104 cfu/mL) and incubated for 18–20 hours at 35°C. The susceptibility of several different bacteria can be evaluated simultaneously on the plates. The MIC is represented by the plate with the lowest concentration of antibiotic that does not support visible growth of the bacteria. The advantages of agar dilution include the ability to simultaneously test the susceptibility of a number of different bacteria; the ability to perform susceptibility testing of fastidious organisms since the agar is able to adequately support their growth; and generation of an exact MIC of the infecting bacteria. However, agar dilution is not commonly utilized in most microbiology labs because it is resource and labor intensive. In addition, the antibiotic plates are not commercially available and need to be prepared before each test since they can be only stored for short periods of time.20,25,29,30
Disk Diffusion Method (Kirby-Bauer)
The disk diffusion method is a well-standardized and highly reproducible qualitative method of antimicrobial susceptibility testing that was developed by Kirby and Bauer in 1966, before broth microdilution, in response to the need for a more practical susceptibility test capable of measuring the susceptibility of multiple antibiotics simultaneously.20,25,28–30 Commercially-prepared, filter paper disks containing a fixed concentration of an antibiotic are placed on solid media agar plates inoculated with a standardized inoculum of the infecting organism (1–2 × 108 cfu/mL). The plates are large enough to accommodate up to 12 different antibiotic disks at the same time (see Figure 17-3). The plate is inverted to avoid moisture on the agar surface, and then incubated for 16–18 hours in ambient air at 35°C. During this incubation time, the antibiotic diffuses out of the disk into the surrounding media, with the highest concentration closest to the disk, as the bacteria multiply on the surface of the plates.20,25,29 The bacteria will only grow in areas on the plate where the concentrations of the antibiotic are too low to inhibit bacterial growth. At the end of incubation period, the plates are examined for the inhibition of bacterial growth by measuring the diameter (in millimeters) of the clear zone of inhibition surrounding each filter paper disk. In general, the larger the zone size, the more active the antibiotic is against the organism.
FIGURE 17-3. A disk diffusion test with an isolate of Escherichia coli from a urine culture. The diameters of all zones of inhibition are measured and those values translated to categories of susceptible, intermediate, or resistant using the latest tables published by the CLSI. (Reprinted, with permission, from reference 25.)
The diameter of the zone of inhibition is correlated to the MIC of the antibiotic from broth or agar dilution against the infecting organism using regression analysis.20,25,28–30 The CLSI has established interpretive criteria based on this relationship to categorize zone diameters as S, I, and R for each antibiotic against each organism.2,25,28,29 Subsequently, the results of the disk diffusion test are considered qualitative because the results only reveal the zone of inhibition and category of susceptibility of the antibiotic against the infecting organism rather than an MIC.
The disk diffusion susceptibility test allows the simultaneous testing of a number of antibiotics in a relatively easy and inexpensive manner, and also provides flexibility in determining the antibiotics that will be tested for susceptibility, providing a filter paper disk for that antibiotic is available. However, the major disadvantages of disk diffusion include the inability to generate an exact MIC and the difficulty in determining the susceptibility of fastidious or slow-growing organisms.
Antibiotic Concentration Gradient Methods
Epsilometer test. The Epsilometer test or Etest® (bioMérieux Diagnostics, Inc.) combines the benefits of broth microdilution with the ease of disk diffusion.11 The Etest® method simultaneously evaluates numerous concentrations of an antibiotic using a single plastic strip impregnated on one side with a known, predefined concentration gradient of an antibiotic. The other side of the Etest® strip is marked with a numeric scale that depicts the concentration of antibiotic at that location on the reverse side of the test strip.2,9,20,25 Like disk diffusion, the Etest® strip is applied onto a solid media agar plate that has been inoculated with a standardized concentration of the infecting bacteria. Several Etest® strips can be placed on the same agar plate providing simultaneous susceptibility testing of several antibiotics.9,20,25 During overnight incubation, bacteria multiply on the agar plates as the antibiotic diffuses out of the Etest® strip according to the concentration gradient. Bacterial growth will occur only in areas on the agar plate where drug concentrations are below those required to inhibit growth. An elliptical zone of growth inhibition will form around the Etest® strip, and the MIC is read as the drug concentration where the ellipse intersects the plastic strip (see Figures 17-4 and 17-5).2,20,25
FIGURE 17-4. Diagram of Etest® strip and gradient.
FIGURE 17-5. Etest® strips on agar showing inhibition of bacterial growth.
The results from the Etest® are reported as the MIC of the infecting bacteria with the corresponding CLSI susceptibility interpretation. The MIC results derived from the Etest® correlate well with the results obtained using other susceptibility testing methods.9,20,25,30 The advantages of the Etest® method include its ease of use, the ability to evaluate the susceptibility of several antibiotics simultaneously; the exact MIC of the infecting bacteria can be determined; and the laboratory can choose the antibiotics to be tested. However, the Etest® method is considerably more expensive than disk diffusion or broth microdilution methods, the results may be reader-dependent, and testing is limited to only those antibiotics for which an Etest® strip is commercially available.
The Etest® is currently used in some labs for the susceptibility testing of fastidious bacteria, such as Streptococcus pneumoniae, Haemophilus influenzae, and anaerobes, as well as for those bacteria in which a routine susceptibility test is not available and an MIC result is preferred.9,25,30
Spiral gradient endpoint test. The spiral gradient endpoint (SGE) test (Spiral Biotech, Inc., Bethesda, MD) is an antibiotic gradient diffusion test that utilizes agar plates containing a continuous radial concentration gradient of antibiotic in the agar from the center of the plate, where the concentration is the highest, to the edge of the plate, where the concentration is the lowest.9,30 The plates are not commercially available but can be made by individual labs with specialized equipment. The infecting bacteria is deposited onto the agar as a radial streak and incubated. Up to 15 bacteria can be tested using a single plate. The MIC is determined by measuring the radial distance between growth at the edge of the plate and where growth is inhibited toward the center of the plate. This measurement is used to compute the concentration of antibiotic at that particular location, which is the MIC.9,30 This method is relatively easy to perform and generates an exact MIC of the infecting bacteria. However, it is relatively expensive, can only test the susceptibility of one antibiotic per plate, and requires the use of specialized equipment. Therefore, it is not routinely used by most microbiology labs.
Specialized Susceptibility Tests
Additional tests may be performed in the microbiology lab to provide further information on the activity of an antibiotic against an organism. These specialty susceptibility tests may measure the bactericidal activity of the antibiotic (e.g., MBC testing, serum bactericidal tests [SBTs], and time-kill curves), or the activity of antimicrobials in combination against an infecting organism (e.g., synergy testing using the checkerboard technique or time-kill studies). These tests are not routinely performed in most microbiology labs due to both biological and technical difficulties, complexity in the interpretation of the results, and uncertain clinical applicability.20,22–24,31
Testing methods for determining bactericidal activity. Several methods measure the direct killing activity of an antibiotic against an organism and, if used, should only be performed for antibiotics that are generally considered to be bactericidal. As noted earlier, there are only a limited number of clinical circumstances where this information may be useful. The determination of bactericidal activity likely has the best clinical utility in the treatment of infections at anatomic sites where host defenses are minimal or absent such as endocarditis, meningitis, and osteomyelitis; as well as in the treatment of severe and life-threatening infections in immunocompromised patients.9,20,22–24,31 Testing methods that determine the bactericidal activity of an antibiotic include the MBC test, time-kill assays, and SBTs.20,22–24,31
The MBC is the lowest concentration of an antibacterial agent that kills 99.9% of the bacterial inoculum, which represents a ≥3 log reduction in the original inoculum.11,23 The methodology for determination of the MBC has been previously described in detail in the section on Broth Macrodilution since it is an extension of that test. CLSI has developed guidelines to standardize the methodology for MBC testing.31 If the MBC exceeds the achievable serum concentrations of the antibiotic, “tolerance” or treatment failure may be observed.20 Tolerance occurs when a normally bactericidal antibiotic can only inhibit the growth of bacteria based on MBC testing. Minimum bactericidal concentration testing is not routinely performed by most labs because it is labor intensive with limited clinical utility.20,22,31
Time-kill studies, also known as time-kill curves, measure the rate of bacterial killing over a specified period of time, which is in contrast to the MBC, which measures the bactericidal activity of the antibiotic at a single point in time following an incubation period.20,24,31 For time-kill studies, a standardized bacterial inoculum is inserted into test tubes containing broth with several different concentrations of an antibiotic (usually the MIC and multiples of the MIC in separate tubes). Samples of the antibiotic-broth solutions are obtained at predetermined time intervals to evaluate the number of viable bacterial colonies present over the 24-hour incubation period.11,20,24,31 The number of viable bacteria present at each time point are plotted over time to determine the rate and extent of bacterial killing of the antibiotic against the organism. A ≥3 log reduction in viable bacterial counts is representative of bactericidal activity.11,20,24,31 Because of the labor and resources involved, this test is not routinely performed in clinical microbiology labs, but is often used in the research setting.
The SBT or Schlichter’s test is similar to MIC and MBC testing, except the SBT testing method measures the bacterial killing activity of the patient’s serum against their own infecting organism after they have received a dose of an antibiotic.9,20,23,24,31–33 The methodology is very similar to determining the MIC using broth macrodilution, but dilutions of the patient’s serum are utilized instead of two-fold serial dilutions of an antibiotic.9,20,24,31–33 The patient’s serum is obtained at predefined intervals before and after a dose of an antibiotic, specifically at the time of expected peak concentration and at the time of expected trough concentration. The patient’s serum is then serially diluted and inoculated with a standardized concentration of the infecting organism. The SBT is the highest dilution of the patient’s serum that reduces the original standardized bacterial inoculum by ≥99.9%. The results of the SBT are reported as a titer, which represents the number of twofold serial dilutions of the patient’s serum that led to bacterial killing (e.g., SBT = 1:16), with a higher titer indicating better activity against the organism.20,22,24,31–33 The CLSI has developed methodology standards for performance of the SBT.31,33 However, this test is not routinely performed by most microbiology labs due to technical difficulties; limited data available regarding the clinical usefulness of SBTs in guiding therapy (only in endocarditis, osteomyelitis, and serious infections in febrile neutropenia); the inability of results to directly predict the response to therapy; and limited clinical applicability.22–24,31–33
Antimicrobial combination testing (synergy testing). In the treatment of bacterial infections, there are clinical situations where combination antimicrobial therapy will be utilized, with the decision to use combination therapy primarily based on the severity of infection, the causative organism, and/or the particular type of infection.11 The potential benefits of combination antibiotic therapy include (1) expanding the antimicrobial spectrum of activity, especially for empiric therapy for a life-threatening infection or for the treatment of polymicrobial infections; (2) producing synergistic bactericidal activity from the combination that is not achieved with each agent alone, such as the use of ampicillin and gentamicin for the treatment of enterococcal endocarditis; and (3) decreasing the emergence of resistant organisms, most notably in the treatment of tuberculosis (TB).19,24 Routine antimicrobial susceptibility tests measure the activity of one antibiotic against a particular organism. There are several tests, however, that evaluate the effects of combination antimicrobial therapy against an infecting organism, with the results being expressed as one of three types of activity11,20:
- Synergy: The activity of the antimicrobial agents in combination is significantly greater than the additive effects of each agent alone.
- Indifference: The activity of the antimicrobial agents in combination is similar to the additive effects of each agent alone.
- Antagonism: The activity of the antimicrobial agents in combination is less than the additive effects of each agent alone.
Therefore, before an antibiotic combination is utilized, it may be useful to determine the effects of the antimicrobial combination against the infecting organism, especially since some antibacterial combinations may produce suboptimal effects.
Synergy testing of an antimicrobial combination is performed using the checkerboard technique, the time-kill curve technique, the disk diffusion assay, or the Etest® method.11,20,24 The checkerboard and time-kill curve techniques are the tests used most often. The checkerboard technique is performed in macrodilution tubes or microtiter plates containing serial dilutions of the antibiotics alone and in combination. The tubes or plates are incubated for 24 hours with a standardized inoculum of the infecting bacteria. The effect of the antibiotic combination is determined by comparing the MICs of the agents when used in combination with the MICs of each agent alone. A synergistic combination displays lower MICs than when each agent is used alone. The time-kill curve method for combination therapy is similar to the time-kill curve method used to determine the rate of bacterial killing of a single agent, except that two antibiotics are added to the tubes in fixed concentrations. The effect of the antibiotic combination is determined by comparing the time-kill rates of combination therapy with the time-kill rates of each agent alone. A synergistic combination displays 100-fold or more greater killing activity than the most potent agent tested alone.11,20 In the clinical setting, synergy testing methods are not routinely performed due to their tedious, time-consuming methodologies; their expense; and their limited clinical applicability in predicting clinical outcome.11,20,24
Methods Detecting the Presence of Antibiotic Resistance Mechanisms
Detection of Beta-Lactamase Activity
To date, over 890 different beta-lactamase enzymes have been characterized.34 Beta-lactamase enzymes can be chromosomally-, plasmid-, or transposon-mediated, and may be produced constitutively or inducibly. These enzymes cause hydrolysis of the cyclic amide bond in the beta-lactam ring and, depending on the type of enzyme, may result in inactivation of one or numerous beta-lactam antibiotics. It is important to understand the consequences of detecting a particular beta-lactamase enzyme in an organism because certain enzymes produce resistance only to certain antimicrobials.20,23,34,35
There are a number of methods that detect the presence of a beta-lactamase enzyme depending on the organism and type of beta-lactamase enzyme suspected. Some tests directly detect the presence of beta-lactamase activity, while other beta-lactamase enzymes (such as the inducible or extended spectrum beta-lactamases) can be suspected based on resistance patterns and MICs derived from routine susceptibility tests.
The assays that directly detect beta-lactamase activity include the acidimetric, iodometric, and chromogenic tests. All of these tests directly measure the presence of beta-lactamase enzyme by observing a color change based on reactions to different substrates, and can be performed in a short period of time with results available within minutes to hours.2,22 The chromogenic test is the most common direct test utilized by microbiology laboratories due to its reliability in detecting beta-lactamase enzymes produced by many different bacteria.23 The chromogenic tests utilize chromogenic cephalosporins (nitrocefin, cefesone, or cefinase) incorporated into filter paper disks or strips that produce a colorimetric change if they are hydrolyzed by beta-lactamase enzymes present when a sample of the clinical specimen is inoculated onto the disk or strip. Test tube assays utilizing chromogenic cephalosporins can also be also utilized. A positive reaction using one of these direct beta-lactamase tests for Haemophilus influenzae, Moraxella catarrhalis, and Neisseria gonorrhoeae predicts resistance to only penicillin, ampicillin, and amoxicillin, but not to other beta-lactam antibiotics that are more stable to hydrolysis by beta-lactamase enzymes.2 A positive beta-lactamase test for Staphylococcus spp. predicts resistance to penicillin, ampicillin, amoxicillin, carbenicillin, ticarcillin, and piperacillin.
Extended-spectrum beta-lactamases (ESBLs) are plasmid-encoded, beta-lactamase enzymes that can hydrolyze penicillins, first-, second-, and third-generation cephalosporins, and aztreonam, and can be inhibited by beta-lactamase-inhibitors such as clavulanic acid.34,35 For some organisms, such as the Enterobacteriaceae and Pseudomonas spp., the production of ESBL enzyme may be inducible so that the detection of beta-lactamase enzyme cannot fully predict the antibiotic susceptibility (or resistance) of the organism.2,23,36 Therefore, direct beta-lactamase testing for these organisms is not recommended since it may produce misleading results. In the recent past, routine susceptibility tests using CLSI breakpoints did not always detect ESBL-producing organisms. The detection of ESBLs in these organisms was improved by the introduction of CLSI guidelines outlining the use of confirmatory tests, which involved MIC and disk diffusion screening breakpoints for particular antibiotics using beta-lactamase inhibitors.21,23,26–28,34–37 In addition, several automated systems, such as Vitek®-2 and the Phoenix™ System, contain ESBL detection tests that, when used with expert system software, are able to accurately detect ESBLs, including some ESBLs not detected by the CLSI confirmatory methods.37
AmpC beta-lactamases are chromosomally- or plasmid-mediated beta-lactamase enzymes that hydrolyze first-, second-, and third-generation cephalosporins and cephamycins, and also display resistance to currently available beta-lactamase-inhibitors such as clavulanic acid, sulbactam, and tazobactam. Many Gram-negative bacteria, such as Serratia marcescens, Pseudomonas aeruginosa, indole-positive Proteus spp., Acinetobacter spp., Citrobacter freundii spp., and Enterobacter spp. (often referred to as the SPICE or SPACE bacteria) contain chromosomally-mediated, inducible AmpC enzymes that, when hyperproduced, can also hydrolyze penicillins and aztreonam in addition to the cephalosporins and cephamycins listed above.34,37 AmpC hyperproduction can occur during the treatment of infection due to one of these organisms, especially when a strong inducer is used such as ceftazidime or clavulanic acid.34 Plasmid-mediated AmpC enzymes have been reported in Klebsiella spp., Proteus mirabilis, Citrobacter koseri, and Salmonella spp., and often display an antibiotic susceptibility profile similar to that of chromosomally-mediated AmpC hyperproducers.34,37 All SPICE and SPACE bacteria should be assumed to be AmpC producers, so that specific tests to detect AmpC production are not essential.37 Plasmid-mediated AmpC beta-lactamases can be detected by demonstrating cephamycin hydrolysis using the AmpC disk test, the Modified Hodge test, or the three-dimensional test.37
There are several types of carbapenemase enzymes that have been characterized (metallo-beta-lactamases, serine carbapenemases such as Klebsiella pneumoniae carbapenemase or KPC), which are able to hydrolyze carbapenems and other beta-lactam antibiotics.34,37 Carbapenemase enzymes may be chromosomally- (Stenotrophomonas maltophilia) or plasmid-mediated (KPCs, Pseudomonas aeruginosa, Acinetobacter spp.), with plasmid-mediated strains often displaying resistance to multiple other drug classes.37 The modified Hodge test can be used for carbapenemase detection on isolates with elevated carbapenem MICs; however, it cannot differentiate between carbapenemase types.23,37
In 2010, the CLSI lowered the cephalosporin and carbapenem breakpoints for Enterobacteriaceae in an attempt to better identify antibiotic agents with predictable efficacy against these bacteria with potentially multiple resistance mechanisms and eliminated the recommendation to perform specialized testing to detect ESBL-, AmpC-, or carbapenemase-mediated resistance. However, this recommendation has gained considerable criticism from many clinicians and microbiologists since detection of the exact mechanism of resistance is thought to be important for both treatment and epidemiologic purposes.37
High-Level Aminoglycoside Resistance
The aminoglycoside antibiotics have relatively poor activity against Enterococcus spp. due to poor intracellular uptake, so they cannot be utilized as monotherapy in the treatment of infections due to enterococci. However, they may be combined with ampicillin, penicillin, or vancomycin in order to achieve synergistic bactericidal activity, as in the treatment of enterococcal endocarditis or enterococcal osteomyelitis. Gentamicin and streptomycin are the two most commonly used aminoglycosides in this situation and are, therefore, the agents most commonly tested for synergistic activity. Supplemental testing can be performed to detect the presence of high-level aminoglycoside resistance (HLAR), which predicts the lack of synergism between gentamicin or streptomycin and cell-wall active agents against Enterococcus spp.2,23,30,36
The presence of HLAR can be evaluated using the agar dilution screening method utilizing agar plates containing high concentrations of gentamicin (500 mcg/mL) and streptomycin (2000 mcg/mL) or the broth dilution method where the well contains BHI broth containing high concentrations of gentamicin (500 mcg/mL) and streptomycin (1000 mcg/mL).2,23 The plates or wells are inoculated with a standardized suspension of the infecting Enterococcus spp. and incubated for 24 hours in ambient air.23 The growth of one or more Enterococcus spp. colonies on the agar plate or in the well demonstrates the presence of HLAR, and the corresponding aminoglycoside cannot be used with a cell-wall active agent to achieve synergistic bactericidal activity. High-level aminoglycoside resistance can also be detected utilizing a disk diffusion method where disks containing high concentrations of gentamicin (120 mcg) and streptomycin (300 mcg) are utilized.2,23 High-level aminoglycoside resistance to gentamicin also confers resistance to tobramycin, netilmicin, and amikacin, but not necessarily streptomycin, which should be tested independently.2,23 A modified test using kanamycin may be used to predict HLAR to amikacin for strains of Enterococcus faecalis; however, this test is not generally available in most labs.2,23 Testing for HLAR is usually performed only on enterococcal isolates from infections that require combination bactericidal activity, such as bacteremia, endocarditis, osteomyelitis, or meningitis.23,30
Tests for the Detection of MRSA, VISA, VRSA, and VRE
Several tests can quickly detect or confirm the presence of methicillin-resistant Staphylococcus aureus (MRSA) or vancomycin-resistant enterococci (VRE). For the detection or confirmation of MRSA, the cefoxitin disk diffusion test, oxacillin-salt agar screening tests, culture-based chromogenic media, rapid latex agglutination (LA) tests, or molecular methods utilizing real-time polymerase chain reaction (PCR) can be utilized.23,38–41 The cefoxitin disk diffusion test is performed using routine CLSI procedures, with modified interpretive criteria utilized to detect MRSA, where MRSA is reported for S. aureus strains with a zone size of ≤21 mm.23,28 This test has also been useful in detecting methicillin-resistance in coagulase-negative staphylococci.23
The oxacillin-salt agar screening tests have been widely used for the detection of MRSA, but they appear to lack sensitivity for the detection of strains that exhibit heteroresistance.23 A standard inoculum of S. aureus is inoculated onto an agar plate containing Mueller-Hinton agar (MHA) supplemented with 4% sodium chloride and 6 mcg/mL of oxacillin, and incubated in ambient air for 24 hours.2,23,36 The growth of more than one colony indicates MRSA, which also confers resistance to nafcillin, oxacillin, cloxacillin, dicloxacillin, and all cephalosporins excluding ceftaroline. However, this test is not recommended for the detection of methicillin-resistance in other Staphylococcus spp.23,36
Culture-based chromogenic media, some of which include MRSASelect (Bio-Rad Laboratories, Redmond, WA), Spectra MRSA (Remel Laboratories, Lenexa, KS), and CHROMagar (BD Sparks, MD), lead to the production of a characteristic pigment in the presence of MRSA.23 These tests are typically utilized for MRSA screening rather than diagnosis of infection, and are less sensitive and slower than molecular methods.23 There are numerous rapid commercial LA tests for the detection of MRSA (MRSA Screen Test [Denka-Seikin Co., Ltd., Tokyo, Japan], the PBP 2′ Test [Oxoid Limited, Basingstroke, UK], the Mastalex test [Mast Diagnostics, Booth, UK], and the Slidex MRSA Detection test [bioMérieux]) that utilize LA to directly detect the presence of penicillin-binding protein (PBP) 2a, the protein encoded by the mecA gene in MRSA.23,40 These tests can be performed within 10–15 minutes from bacterial colonies cultured on blood agar plates, decreasing the overall MRSA detection time by approximately 24 hours when compared to standard methods, and are highly sensitive and specific for the detection of MRSA.40
The GeneOhm™ MRSA Assay (BD, Franklin Lakes, NJ) the Xpert MRSA (Cepheid, Sunnyvale, CA), and the LightCycler MRSA Advanced test (Roche Diagnostics, Indianapolis, IN) are approved real-time PCR assays for the rapid, direct detection of nasal colonization by MRSA for the prevention and control of MRSA infection in healthcare institutions.23,38,39 These assays can detect the presence of MRSA directly from nasal swab specimens within 2 hours using real-time PCR that couples primers specific for mecA and the S. aureus-specific gene orfX (sensitivity 93%, specificity 96%).38,39 A PCR-based test also exists for the detection of Staphylococcus aureus and MRSA from blood cultures positive for Gram positive cocci in clusters (XPert MRSA/SA BC test, Cepheid, Sunnyvale, CA), with results typically available within 1 hour of culture positivity.41 The manufacturer has recently suspended availability of the product due to invalid results and is currently performing product improvements.
The CLSI reference broth microdilution method can accurately detect vancomycin intermediate Staphylococcus aureus (VISA, MIC 4–8 mcg/mL) and vancomycin-resistant Staphylococcus aureus (VRSA, MIC ≥16 mcg/mL).23 The use of BHI plates with 6 mcg/mL of vancomycin (VRE screening plates described below) can be considered for the detection of Staphylococcus aureus strains with an MIC of 8 mcg/mL, but is not useful for VISA strains with an MIC of 4 mcg/mL. Lastly, the disk diffusion test is unable to accurately detect VISA strains, but will detect VRSA strains mediated by vanA.23
Current automated susceptibility testing methods, including Vitek 2 and the Phoenix system, are now able to accurately detect the presence of VRE.23 However, VRE can also be detected utilizing the vancomycin agar screen test, which is most often performed on rectal swab specimens to detect carriers of VRE. A standard inoculum of the infecting Enterococcus spp. is inoculated onto an agar plate supplemented with brain heart infusion broth (BHI) containing vancomycin 6 mcg/mL and incubated in ambient air for 24 hours.2,23,36 The presence of any growth demonstrates the presence of VRE. This test is most useful for detecting acquired vancomycin resistance in E. faecalis and E. faecium, but is not useful for strains that display intrinsic resistance to vancomycin, such as E. gallinarum and E. casseliflavus. The vancomycin agar screening method test is also useful to detect the presence of a newly emerging resistant pathogen, namely vancomycin-intermediate Staphylococcus aureus (VISA).11,23,30,36
D Test for Detecting Inducible Clindamycin Resistance
Resistance to clindamycin in staphylococci and beta-hemolytic streptococci is typically mediated by expression of the erm gene conferring resistance to macrolides, lincosamides, streptogramin b, or MLSb-type resistance, which can be constitutive or inducible.20,23 Staphylococcal or beta-hemolytic streptococcal isolates that are macrolide resistant but clindamycin susceptible should be evaluated for inducible clindamycin resistance using the “D” test.20,23 The D test is a disk diffusion procedure where a 15-mcg erythromycin disk is placed 15–26 mm apart from a 2-mcg clindamycin disk on an agar plate inoculated with the infecting organism.20,23 If inducible clindamycin resistance is present in the organism, the clindamycin zone of inhibition will be flattened on the side nearest the erythromycin disk, demonstrating the letter “D” in appearance. Organisms that display a flattening of the clindamycin zone are D test positive and will be reported resistant to clindamycin in the final laboratory report.
Special Considerations for Fastidious or Anaerobic Bacteria
The susceptibility testing of fastidious bacteria (e.g., Haemophilus influenzae, N. gonorrhoeae, and Streptococcus pneumoniae) and anaerobes cannot be performed utilizing standard broth microdilution, disk diffusion, or automated susceptibility testing methods since these organisms require more complex growth media and environmental conditions to support bacterial growth.36,42–45 The cultivation of fastidious bacteria or anaerobes may require media with supplemental nutrients, prolonged incubation times, and/or incubation in atmospheres with higher CO2 concentrations.45 Microbiology reference texts and CLSI standards have been developed to outline specific methodologies (broth dilution, disk diffusion, and automated methods), quality control guidelines, and interpretive breakpoint criteria that should be utilized for the susceptibility testing of these bacteria.21,25–29,36,42–45
The clinical significance of anaerobes as a cause of infection is more widely appreciated, and the susceptibility of anaerobes to various anti-infective agents is no longer predictable.9,42–44,46,47 The handling and processing of biologic specimens for anaerobic culture and susceptibility testing are extremely crucial to the validity of the results since most anaerobic bacteria of clinical importance are intolerant to oxygen.2,9,42 Specimens should be collected in appropriate anaerobic transport systems (commercially-available vials or tubes) that contain specialized media and atmospheric conditions to support the growth of the anaerobic bacteria until the specimen is processed in the lab.2,42 Once collected, the specimens should be transported to the lab within minutes to hours of collection, set up for culture in anaerobic jars or chambers in the appropriate growth media, and incubated in anaerobic atmospheric conditions. The clinical specimens that provide the best yield for anaerobic culture include aspirated or tissue biopsy specimens.2,42,47
The identification of anaerobic bacteria by an individual hospital laboratory may be performed using one of three methods: (1) presumptive identification based on information from the primary growth plates including the Gram stain results, patterns of growth on selective or differential media, plate and cell morphology, and results of various rapid spot and disk tests; (2) definitive identification based on the results of individual biochemical tests that detect the presence of preformed enzymes found in certain anaerobes; and (3) rapid identification of anaerobes using commercially-available detection panels, which also rapidly detect the presence of preformed enzymes such as the BBL Crystal Anaerobe ID (Becton Dickinson and Company), the RapID-ANA II (Remel, Inc.), the Rapid Anaerobe Identification Panel (Siemens Healthcare Diagnostics, Inc.), or the Vitek®-2 ANC (bioMérieux Diagnostics, Inc.).2,43 Many hospital laboratories do not have the resources for commercially-available, anaerobic bacteria identification systems, and rely on the first two methods for presumptive identification of anaerobic bacteria. If necessary, clinical isolates can be sent to a reference lab for further testing.
Most clinical microbiology labs do not currently offer routine susceptibility testing of anaerobic bacteria because of the uncommon occurrence of pure anaerobic infections, the uncertain role of anaerobes in mixed infections, the previous predictable susceptibility of anaerobic bacteria to antibiotics, the previous lack of standardization of antimicrobial susceptibility testing of anaerobes, and the technical difficulties in performing the tests.30,43,44,46,47 However, it is becoming apparent that routine antimicrobial susceptibility testing of anaerobic bacteria is necessary due to the increasing incidence of serious infections caused by anaerobic bacteria, the emerging resistance of anaerobic bacteria to multiple antibiotic agents, and the poor clinical outcomes observed when ineffective antibiotics are utilized for the treatment of infections due to anaerobes. 30,43,44,46,47
The susceptibility testing of anaerobic bacteria has undergone numerous methodological modifications and standardization over the past several years.9,44, 46,47 The CLSI has recently published a standard outlining when anaerobic susceptibility testing should be considered, which methods of susceptibility testing should be utilized, when and how surveillance susceptibility reporting should be performed, and which antibiotic agents should be tested for susceptibility.46
Susceptibility testing for anaerobes should be performed in patients with serious or life-threatening infections such as endocarditis, brain abscess, osteomyelitis, joint infection, refractory or recurrent bacteremia, and infection of prosthetic devices or vascular graft infections.9,43,44,46 Susceptibility testing should also be performed in patients with persistent or recurring anaerobic infections despite appropriate antibiotic therapy.9,44,46 Lastly, susceptibility testing of anaerobic bacteria should be periodically performed within geographic areas or individual institutions to monitor regional susceptibility patterns of anaerobic bacteria over time.43,44,46
The recommended anaerobic susceptibility testing methods include agar dilution and broth microdilution using supplemented Brucella broth, both of which can be reliably performed by most clinical microbiology labs.9,43,44,46,47 The agar dilution method is the gold standard reference method that can be utilized to test the susceptibility of any anaerobic bacteria, while the broth microdilution method has only been validated for antimicrobial susceptibility testing of Bacteroides fragilis group organisms.44,46 In contrast to agar dilution, the broth microdilution method can evaluate the susceptibility of multiple antibiotics simultaneously. Otherwise, the general methodology for each of these tests is similar to those described above for aerobic bacteria. Other methods that are utilized for susceptibility testing of anaerobes include agar dilution, broth microdilution, and the Etest®. Broth disk elution and disk diffusion are not recommended since their results do not correlate with the agar dilution reference method.43,44,46,47 Beta-lactamase testing of anaerobes can be performed according to CLSI guidelines using chromogenic disks.44,46
Since routine antimicrobial susceptibility of anaerobes is not performed by all hospital microbiology laboratories or for all anaerobic isolates, antibiotic therapy for infections due to anaerobes is usually selected empirically based on susceptibility reports published by reference labs.44 However, if susceptibility testing is performed on an individual anaerobic isolate, the results should be used to guide the anti-infective therapy for the patient.
Methods for Reporting Susceptibility Results
Individual Isolate Susceptibility Reports
When a bacterial isolate is recovered from a clinical specimen, the identification and susceptibility results are compiled in a report that is available electronically or via a hard copy and placed into the patient’s chart. The bacterial identification and antibiotic susceptibility report often contains the following information: the patient’s name, medical record number, the date and time of specimen collection, the source of specimen collection (e.g., blood, wound, urine, etc.), the bacteria that were identified (if any), and the list of antibiotics tested for susceptibility along with their MIC or disk diffusion results and CLSI interpretive category, as shown in Figure 17-6.20,48 In some hospitals, the susceptibility report may also contain information regarding the usual daily doses and costs of the antibiotics that were tested.
FIGURE 17-6. Example of microbiology laboratory report with bacterial identification and antibiotic susceptibility.
Once the culture and susceptibility results are available, this information should be utilized, if necessary, to change the patient’s empiric antibiotic regimen, which usually covers a broad-spectrum of bacteria, to a more directed antibiotic regimen targeted at the infecting bacteria and susceptibility. The directed antibiotic regimen should be chosen based on clinical and economic factors, some of which include the severity of infection, the site of infection, the activity of the antibiotic against the infecting organism, the proven efficacy of the antibiotic in the treatment of the particular infection, the overall spectrum of activity of the antibiotic (a narrow spectrum agent is preferred), the end-organ function of the patient, the presence of drug allergies, the route of administration required (oral versus parenteral), and the daily cost of the antibiotic, etc. The susceptibility report provides some of the information required for the antibiotic decision-making process, namely, the site of infection, the identification of the infecting organism(s), and the susceptibility of the infecting organism(s).
As seen in the sample susceptibility report in Figure 17-6, there may be a number of antibiotics to which the infecting organism is susceptible, often with differing MICs. It is not always advantageous to choose the antibiotic with the lowest MIC against a particular organism on a susceptibility report. As discussed earlier in this chapter, each antibiotic has different MIC breakpoints corresponding to S, I, and R for each bacteria based on a number of factors. Some drugs, such as the parenteral piperacillin-tazobactam, are assigned higher MIC breakpoint values for susceptibility since they achieve higher serum and/or site concentrations than other antibiotics. Because of this, a simple number comparison of the MIC between antibiotics should not be performed. The choice of antibiotic should be based on the knowledge of the MICs that are acceptable for a particular drug-bacteria combination, the site of infection, the penetration of the antibiotic to the site of infection, as well as the clinical and economic parameters listed above. In the sample report in Figure 17-6, oxacillin (nafcillin) or cefazolin would be an acceptable choice for the treatment of Staphylococcus aureus bacteremia in a patient without drug allergies since these agents are active against the infecting organism, have been demonstrated to be effective in the treatment of systemic staphylococcal infections, are relatively narrow-spectrum antibiotics, and are inexpensive. Minicase 2 is an example illustrating the use of a culture and susceptibility report in the antibiotic decision-making process.
Using Lab Test Results to Guide Choice of an Antibiotic Regimen for Urosepsis/Pyelonephritis
DIANA J., A 27-YEAR-OLD FEMALE, presents to the Urgent Visit Center with complaints of urinary frequency and urgency, pain on urination, and hematuria for the past 2 days. The patient also states that she recently developed a fever to 101.6°F and has experienced intractable nausea and vomiting for the past 24 hours. Upon presentation in clinic, she is febrile (102.3°F), hypotensive (90/60), and lethargic; physical exam reveals right costovertebral angle and suprapubic tenderness. A urine dipstick performed in clinic is leukocyte esterase positive, and a urine pregnancy test is negative. Because she is so ill-appearing, the clinic physician admits the patient to the hospital. Her past medical history is significant for recurrent UTIs, with three episodes over the past 6 months that have required antibiotic therapy including trimethoprim–sulfamethoxazole and ciprofloxacin. Diana J. reports no known drug allergies. Upon admission, a urinalysis, urine culture, and blood cultures are performed. The results of her urinalysis and cultures are at the right.
Question: What is an appropriate recommendation for antibiotic therapy for this patient?
Discussion: Diana J. is presenting with pyelonephritis and urosepsis (complicated UTI), making the acquisition of a urinalysis, urine culture, and blood culture useful in guiding antimicrobial treatment since her past UTIs and subsequent antibiotic treatment put her at risk for acquiring an infection with a resistant bacteria. Based on her presenting symptoms and the findings on her physical examination, Diana J. most likely has acute, pyelonephritis. Because she is hypotensive on admission and is experiencing significant nausea and vomiting, she should initially be treated with a parenteral antibiotic that displays activity against the infecting organism and has been proven clinically to display efficacy against complicated UTIs. Based on lack of antibiotic allergies and the results of her urine culture and susceptibility, Diana J. can be treated with parenteral cefazolin or ceftriaxone.
Urinalysis: Yellow, cloudy; pH 7.0, specific gravity 1.015, protein negative, RBC trace, WBC 50–100/hpf, leukocyte esterase positive, nitrite positive
Midstream Urine Culture/Susceptibility: >100,000 cfu/mL of Escherichia coli
ANTIBIOTIC TESTED | MIC RESULT | INTERPRETATION |
Ampicillin | >32 mcg/mL | R |
Ampicillin–sulbactam | 8 mcg/mL | S |
Cefazolin | 1 mcg/mL | S |
Ceftriaxone | 1 mcg/mL | S |
Imipenem | 1 mcg/mL | S |
Gentamicin | 0.5 mcg/mL | S |
Ciprofloxacin | 4 mcg/mL | R |
Trimethoprim–sulfamethoxazole | >80 mcg/mL | R |
Blood culture/susceptibility: | Escherichia coli |
ANTIBIOTIC TESTED | MIC RESULT | INTERPRETATION |
Ampicillin | >32 mcg/mL | R |
Ampicillin–sulbactam | 8 mcg/mL | S |
Cefazolin | 1 mcg/mL | S |
Ceftriaxone | 1 mcg/mL | S |
Imipenem | 1 mcg/mL | S |
Gentamicin | 0.5 mcg/mL | S |
Ciprofloxacin | 4 mcg/mL | R |
Trimethoprim–sulfamethoxazole | >80 mcg/mL | R |
R = resistant; S = susceptible.
The decision regarding the antibiotics that will be reported on an individual susceptibility report for a bacterial isolate is typically based on input from a multidisciplinary committee (e.g., Antimicrobial Subcommittee, Infectious Diseases Subcommittee, Antimicrobial Stewardship Team) comprised of infectious diseases physicians, the infectious diseases pharmacists, the Infection Control Committee, and the microbiology laboratory of a given hospital.2 These decisions are often based on the antibiotics that are available on the hospital formulary, the level of control of antibiotic use that is desired, and the tests that are utilized by the microbiology laboratory for susceptibility testing. Tables that outline the antibiotics that should be routinely tested and reported for certain organisms can be found in the CLSI Performance Standards and Guidelines for susceptibility testing of bacteria.21,26–28
The methods utilized for reporting antibiotic susceptibility of bacteria for individual isolates include general reporting, selective reporting, and cascade reporting. General reporting involves reporting all antibiotics that were tested for susceptibility against the organism without any restrictions or analysis. Selective reporting strategies include information on the susceptibility of antibiotics available for routine use on the hospital formulary or only those antibiotics that are useful for the treatment of a particular organism or infection type. An example of selective reporting would be the exclusion of cefazolin from the susceptibility report of a CSF sample growing E. coli since cefazolin is not a suitable treatment option for meningitis. Cascade reporting strategies include information on the susceptibility of antibiotics that the hospital/committee considers to be first-line choices for the treatment of a particular organism or infection, with reporting of second-line agents if the first-line agents are inappropriate for the treatment of the particular infection, or if the first-line agents are inactive against the infecting organism. This process is utilized as a method to control the inappropriate use of broad-spectrum or expensive antibiotics.2,48 An example of cascade reporting is the reporting of the susceptibility result of amikacin against Pseudomonas aeruginosa only if the organism is resistant to gentamicin and/or tobramycin, which are less expensive aminoglycoside agents.
Hospital Susceptibility Reports (Hospital Antibiograms)
Many hospitals prepare and publish an annual cumulative report of the antimicrobial susceptibility profiles of the organisms that have been isolated from the patients within their hospital, healthcare system, or institution, called a cumulative antibiogram. The cumulative antibiogram usually reflects the antibiotic susceptibility patterns of isolates obtained from patients in the hospital who were admitted with an infection or developed an infection in the hospital (nosocomially-acquired); although, some hospitals with a large outpatient population may also include isolates from patients in the surrounding community, usually in a separate outpatient table. Antibiotic therapy must often be initiated at the suspicion of infection since many infectious diseases are often acute where a delay in treatment may result in significant morbidity or mortality (e.g., meningitis and pneumonia). Therefore, the cumulative antibiogram is a useful tool for selecting the most appropriate empiric antibiotic therapy based on the susceptibility of the organism that is most likely causing the patient’s infection (Table 17-7) while waiting for the culture and susceptibility results.48 During empiric therapy selection, an antibiotic is typically chosen based on the local susceptibility patterns of the most likely infecting organism, as described in Minicase 3. However, once the culture and susceptibility results of the infecting bacteria are known, antibiotic therapy should be streamlined, if necessary, to an agent with more targeted activity against the organism. In order for the cumulative antibiogram to be clinically useful, the susceptibility data from patient isolates should be appropriately collected, analyzed, and reported according to the CLSI guidelines, which are outlined in Table 17-10.48
Using Lab Test Results to Guide Choice of an Antibiotic Regimen for Bacteremia
DAVID M. IS A 45-YEAR-OLD MALE who sustained multiple traumatic injuries after a motorcycle accident. He has required multiple surgeries over the past 10 days for fracture stabilization. In the last 12 hours, he has spiked a temperature to 39°C and has developed shaking chills. His other vital signs are stable, and his physical exam does not demonstrate any significant findings. Urinalysis, urine culture, and blood cultures are performed to determine the potential etiology for his new fever. In addition, a chest x-ray is performed, which did not demonstrate any pulmonary infiltrates. The lab calls the surgical floor later that day to report that the blood cultures are positive for Gram-negative rods. The patient is allergic to penicillin (nonurticarial rash), and the hospital antibiogram is pictured in Figure 17-7.
Question: What empiric antibiotic regimen should be used to treat David M.’s Gram-negative rod bacteremia?
Discussion: Nosocomial Gram-negative bacteremia is a potentially life-threatening infection, which requires aggressive antibiotic therapy for treatment. The choice of whether to use monotherapy or combination therapy while waiting for culture and susceptibility results in this setting will often depend on the clinical condition of the patient and local susceptibility patterns. Combination antibiotic therapy might provide some antibacterial synergy, as well as provide coverage against a wide range of potential infecting bacteria. Based on the hospital antibiogram in Figure 17-7, it is desirable to choose antibiotics that retain good activity (>85% susceptible) against Gram-negative bacteria isolated at the institution, namely, Pseudomonas aeruginosa, E. coli, Klebsiella pneumoniae, Serratia marcescens, and Enterobacter cloacae, as well as choose agents that have demonstrated efficacy in the treatment of bacteremia. Since David M. is clinically stable and displays only a rash to penicillin therapy, some useful therapeutic options include imipenem, meropenem, ceftazidime, cefepime, or ciprofloxacin monotherapy. If he begins to clinically deteriorate, an aminoglycoside, such as tobramycin, or a fluoroquinolone, may be added to the carbapenem or cephalosporin while waiting for the results of the culture and susceptibility. The antibiotic regimen can be modified to more directed therapy, if possible, once the results of the culture and susceptibility are available.
FIGURE 17-7. Example of a hospital antibiogram.
The cumulative antibiogram contains information on the percent of isolated organisms that were susceptible to antibiotics tested over the time frame of the antibiogram, as illustrated in Figure 17-7.48 The information is derived by dividing the number of organisms susceptible to a particular antibiotic by the total number of single-patient isolates collected and reported (with duplicate patient isolates removed). The calculations can be performed either manually or using automated systems that have been programmed using appropriate definitions to remove duplicate patient isolates. The data published in the cumulative antibiogram should be based on input from infectious diseases physicians, infectious disease pharmacists, the Infection Control Committee, the Pharmacy and Therapeutics Committee, and the microbiology laboratory of a given hospital or healthcare system. Cumulative antibiograms may contain separate data tables for reporting the susceptibility of Gram-positive, Gram-negative, and anaerobic bacteria; as well as separate data tables reporting the antibiotic susceptibility patterns of organisms isolated from patients in key patient care units (e.g., Burn Unit, Medical ICU, Pediatric Unit, Med-Surg Unit, outpatient clinic, nursing home, etc.), with particular infection types (e.g., susceptibility of bloodstream isolates or urinary tract isolates), with specific medical conditions (e.g., cystic fibrosis, transplant patients, etc.), or by organism (e.g., susceptibility of Staphylococcus aureus). For some organisms, the cumulative antibiogram will only contain information regarding the presence of bacterial resistance mechanisms, particularly when routine susceptibility testing is difficult to perform, such as in the case of Haemophilus influenzae where the percentage of isolates that produce beta-lactamase enzyme during the time period of the cumulative antibiogram will be reported. Other information may be incorporated into a cumulative antibiogram including antibiotic dosing guidelines, recommended empiric antibiotic choices based on infection type, antibiotic cost data, etc.48
Surveillance Susceptibility Testing of Large Numbers of Isolates
Surveillance susceptibility testing is a useful method to monitor the susceptibility of bacteria to antimicrobial agents over time, and can be performed in an individual hospital or within a geographic location (e.g., regionally, nationally, and internationally).20 Surveillance studies typically report the overall susceptibility of the bacteria to particular anti-microbial agents using CLSI breakpoints, along with other susceptibility parameters such as the MIC50 and the MIC90. To determine the MIC50 or MIC90, the MIC values from the bacteria studied are arranged in ascending order where the MIC50 is the MIC value representing 50% of the bacterial population (the MIC value of the isolate that represents 50% of the bacterial population studied) and the MIC90 is the MIC that represents 90% of the bacterial population (the MIC value of the isolate that represents 90% of the bacterial population studied). The MIC90 value is usually higher than the MIC50 value. This information is useful for detecting the emergence of subclinical antibiotic resistance where the MIC50 and MIC90 of a particular agent may be increasing over time but are still below the MIC susceptibility breakpoint.
Additional Considerations When Interpreting Susceptibility Results
The successful treatment of a patient’s infection involves an understanding of the interactions among the patient, the infecting organism, and the antibiotic. It is important to note that antimicrobial susceptibility testing only measures one of these factors, namely, the activity of the antibiotic against the infecting organism in a laboratory setting. The current methodologies for antibiotic susceptibility testing are unable to reproduce the interaction between the antibiotic and the bacteria at the site of infection where a multitude of host factors (e.g., immune system function, concomitant disease states and drugs) and drug factors (e.g., pharmacokinetic parameters including concentration of free drug at the site of infection and protein binding) play an integral role.
FUNGI
Fungi are classified as one of the six kingdoms of life. There are approximately 500 named species of fungi that are known to cause infection in humans and other vertebrate animals.49 Approximately 50 fungal species are associated with infections in healthy subjects and the majority of fungal infections occur in immunocompromised or debilitated patients by organisms that are part of the normal human flora. However, an increasing number of serious and life-threatening opportunistic infections are being caused by ubiquitous environmental molds.
One of the most challenging and frustrating aspects of diagnostic medical mycology is the terminology, taxonomy, classification, and nomenclature of fungi. For example, the correct name for a species of fungi is that which was published earliest and met the requirements in the International Code of Botanical Nomenclature (http://www.bgbm.org/iapt/nomenclature/code/default.htm). All subsequent names are considered synonyms; however, exceptions do exist, particularly when a later name is more commonly used than the earlier name or if research requires a species to be transferred to a different genus. Changes have occurred between kingdoms as well. For example, members of the genus Pneumocystis, which were originally placed in the kingdom Protozoa, are now reclassified as fungi and placed in the phylum Ascomycota. The common human pathogen from this genus is Pneumocystis jirovecii (formerly Pneumocystis carinii). Because of these issues, the reader is referred to the latest editions of standard microbiology textbooks and reference manuals (e.g., Manual of Clinical Microbiology, American Society for Microbiology Press) for more detailed information on taxonomy and classifications of fungi. In addition, a glossary of common mycological terms is often included.49
Fungi are eukaryotic and can be either unicellular or multicellular organisms. Fungi have cell walls composed mainly of chitins, glucan, and mannam with a membrane-bound cell nucleus with chromosomes. The dominant sterol in the cytoplasmic membrane of fungi is ergosterol, compared to cholesterol in mammalian cells. These organisms are heterotropic (e.g., require exogenous energy sources) and can reproduce by either asexual (involving mitosis) or sexual (involving meiosis) cell division. Fungi may exist in a morphologic form that results from sexual reproduction (teleomorph, or perfect state) and/or a form that results from asexual reproduction (anamorph or imperfect state), where each of the forms has its own name (e.g., the sexual form of Scedosporium apiospermum complex is Pseudallescheria boydii).
Fungi have traditionally been categorized into mold, yeast, or dimorphic fungi based on morphological and structural features (Table 17-11).49–53 Molds (or moulds) are long, cylindrical, and threadlike (filamentous) fungi that form multicellular mycelium or thallas, an intertwined mass of branching hyphae [tube-like extensions or filament-like cells], with septa (having cross walls; being septate) or pauciseptate. An asexual spore (conidium) is produced on conidiophores, a specialized hyphal structure that serves as a stalk, and macroconidia and/or microconidia may be present. Thermally, monomorphic molds can be divided into four groups: (1) Zygomycetes; (2) dematiaceous fungi; (3) dermatophytes; and (4) hyaline hyphomycetes. Zygomycetes have broad hyphae that are almost nonseptate with asexual spores (sporangiospores) formed by cleavage in a saclike structure (sporangium). The most common Zygomycetes observed in the clinical laboratory are from the order Mucorales, which are associated with severe fungal infections referred to as mucormycosis. Two genera from the order Entomophthorales, Basidiobolus and Conidiobolus, are less commonly observed Zygomycetes but are responsible for subcutaneous infections in otherwise healthy individuals. Dematiaceous fungi produce dark colored colonies of olive, brown, gray or black due to melanin pigment in the cell walls. Some of the common infections associated with dematiaceous fungi include chromoblastomycosis, phaeohyphomycosis, and mycetoma. Dematiaceous fungi also cause tinea nigra and black piedra. Dermatophytes are most often associated with superficial fungal infections (tinea or ringworm) of the skin, hair, and nails. These filamentous fungi colonize the outermost layer of the skin and digest keratin as a source of nutrients. The three genera (Microsporum, Trichophyton, and Epidermophyton) are differentiated by their conidium formation (macroconidia or microconidia). Hyaline hyphomycetes are colorless, septate hyphae molds that produce conidia that may be either colorless or pigmented. Coccidioides immitis and Coccidioides posadasii are known pathogens from this group while most other organisms cause opportunistic infections in immunocompromised patients.
aRefer to reference 53 for further details and other organisms not listed.
bCoccidioides immitis is often placed with dimorphic fungi (listed in this table under hyaline hyphomycetes since it does not produce yeast-like colonies or cells at 35°C to 37°C on routine mycology agar).
cResults from molecular studies have recommended that the phylum Zygomycota be divided among a new phylum, Glomeromycota and four subphyla, changing the class Zygomycetes to glomeromycetes. Many textbooks continue to Zygomycetes until the taxonomy is definitively resolved. Among the subphylum Mucormycotina, the order Mucorales includes the genera Rhizopus, Mucor, Rhizomucor, and Lichtheimia (formerly Absidia). The subphylum Entomophthoromycotina contains the order Entomophthorales, which includes genera Basidiobolus and Conidiobolus.
dSporothrix schenckii grows as a dematiaceous mold when incubated at 25°C to 30°C but is yeast-like at 35°C to 37°C. It is commonly categorized among dimorphic fungi but is often considered as a dematiaceous mold.
Yeasts (and yeast-like organisms) appear as round or oval cells that are unicellular and generally reproduce at their surface by budding (blastoconidia). Some produce pseudohyphae (an elongated chain of cells, like a chains of sausages, resembling hyphae; however, borders between cells are delineated by marked constrictions) while others have true hyphae (tend to be straighter and without constrictions at the septa), which may be septate or without septate (aseptate). Ascospores (a sexual spore in a saclike structure [ascus]) are produced by only some yeast. Yeasts are the most frequent encountered fungi in the clinical microbiology laboratory and are considered opportunistic pathogens. Candida spp. and Cryptococcus spp. are among the most common yeasts causing fungal infections. Yeasts are not considered as a formal taxonomic group but as a growth form of unrelated fungi (members of the phyla Basidiomycota and Ascomycota).
Dimorphic fungi have two distinct morphological forms where their growth forms can change from a multicellular mold (in their natural environment or when cultured at 25°C to 30°C) to budding, unicellular yeasts (during tissue invasion or when cultured at 35°C to 37°C). Medically important dimorphic fungi include Histoplasma capsulatum, Blastomyces dermatitidis, Paracoccidioides brasiliensis, Penicillium marneffei, and Sporothrix schenckii. All of these fungi are considered pathogenic and must be handled with caution in the clinical laboratory.
The Identification of Fungi
The following section provides a brief summary of the common methods currently used in diagnostic testing of medically important fungi.50–53 Fungal identification has traditionally been based on morphological characteristics such as the color of the colonies, the size and shape of cells, the presence of a capsule, and the production of hyphae, pseudohyphae, or chlamydospores; however, culture remains the “gold standard” in most clinical microbiology laboratories. Deoxyribonucleic acid (DNA) sequence analysis and molecular characteristics are being rapidly explored and gaining a larger role in fungal identification, particularly when morphology-based identification is atypical, confusing, or not helpful (e.g., organisms that fail to sporulate) and in cases where precise identification is required (e.g., epidemiological studies).
Laboratory diagnosis of fungal infections includes direct microscopic examination, isolation in culture, morphologic identification, biochemical, serologic, and molecular diagnostic testing. As with all types of infections, appropriate biological specimens need to be selected, collected, and transported to the laboratory for immediate processing.50 Since different fungi are capable to causing infection at a number of anatomical sites, specimens from the site of infection as well as peripheral blood specimens should be considered and submitted for culture and microscopic examination. Communication with the laboratory regarding the clinical infection and suspected fungi is important, and may be useful for determining how best to process specimens safely and efficiently, including pretreatment and staining procedures, selection and incubation of media, and choice of additional diagnostic testing. Early identification of the infecting fungal pathogen may have direct diagnostic, epidemiologic, prognostic, and therapeutic implications.
The first step is usually identifying yeast-like fungi (pasty, opaque colonies) from molds (large, filamentous, colonies that vary in texture, color, and topography). Drawings, color plates, and brief descriptions found in standard textbooks can serve as a guide and assist in the preliminary identification of fungi seen on direct microscopic examination of clinical specimens.52,53 Microscopic examination of the clinical specimen can delineate morphologic features (Table 17-12) and often provides preliminary identification of many fungi (e.g., Aspergillus spp., Zygomycetes, dematiaceous molds). Microscopic morphology can often provide definitive identification of a mold whereas the addition of biochemical tests, serology, and/or nucleic acid (NA)–based molecular testing are usually needed for identifying the genus and species of most yeast and yeast-like fungi. Direct microscopic examination of properly stained clinical specimens and tissue sections is usually the most rapid (within a few minutes or hours) and cost-effective method for a preliminary diagnosis of fungal infection. In addition, microscopic detection of fungi can assist the laboratory in the selection of media and interpretation of culture results.
The Gram stain that is typically used for bacterial processing may also allow the detection of most fungi, especially Candida spp., since the size of the smallest fungi is similar in size to large bacteria; the presence of budding cells can also be observed. A wide range of stains are available (Table 17-13) to assist in the rapid detection of fungal elements.51–53 A common approach to wet preparations of specimens or smeared dried material is to use a 10% solution of potassium hydroxide (KOH) with or without fluorescent calcofluor white (CFW) stain. The strong alkaline KOH solution digests tissue elements in order to allow better visualization of the fungi, while the CFW stain binds to chitin and polysaccharides in the fungal cell wall allowing it to appear white under ultraviolet light. Specific staining techniques are often used to outline morphologic features that are diagnostic and distinctive of the suspected fungal organism (e.g., India ink stain for detection of a polysaccharide capsule of Cryptococcus neoformans). In suspected cases of histoplasmosis, the Giemsa or Wright stain is useful for detecting intracellular yeast cells within macrophages from blood or bone marrow specimens.
BAL = bronchoalveolar lavage; CSF = cerebrospinal fluid.
Histopathologic stains are extremely valuable for identifying fungal elements in tissues and host tissue reactions to fungal infection. Histology laboratories commonly use stains such as hematoxylin and eosin (H&E) for these general purposes. Periodic acid-Schiff (PAS) and Gridley fungus (GF) stains can also assist in visualization of fungal elements, especially if debris is present in the tissue background. Special stains such as Gomori methenamine silver (GMS), mucicarmine, and Fontana-Masson (FM) are useful for enhancing the detection of specific fungal elements (see Table 17-13).51–53
Culture remains the gold standard for isolation and identification of fungi suspected of causing infection. Petri plates are preferred over screw-cap tubes because of the larger surface area and dilution of inhibitory substances in the specimens. However, for laboratory safety reasons, most thermally dimorphic fungi (e.g., Histoplasma, Blastomyces, Paracoccidioides, Penicillium marneffei) and Coccidioides spp. are pathogenic and should be grown on slants (i.e., avoid the use of Petri plates and slide culture). A variety of media are available for the isolation and cultivation of yeasts and molds (Table 17-14).51,53 Sabouraud dextrose and brain heart infusion (SDBHI) or BHI agar are enriched media commonly recommended to permit the growth of yeasts and molds. Several media, with (selective) and without (nonselective) inhibitory agents, should be used since no one media is adequate for all the different types of specimens or organisms. Antibiotics such as chloramphenicol or gentamicin are included as inhibitory substances of most bacterial contaminants whereas cycloheximide is used to inhibit saprophytic fungi and prevent the overgrowth of contaminating molds. Nonselective media (without inhibitory agents) should be used with specimens from sterile sites and when suspected fungi are likely to be inhibited by cycloheximide (e.g., Aspergillus fumigatus, Fusarium, Scopulariopsis, Cryptococcus neoformans/gattii, some Candida spp., most Zygomycetes) or by antibiotics (e.g., Nocardia or other filamentous bacteria). Direct microbiological examination (outlined above) of clinical specimens can assist in the selection of media based on specimen type and suspected pathogen. In addition, the choice of media will be influenced by the patient population, local endemic pathogens, cost, availability, and laboratory preferences.
Proper incubation temperature and sufficient incubation time are also needed to optimize the recovery of medically important fungi from clinical specimens. Inoculated media should be incubated aerobically at 30°C. If an incubator at that temperature is not available, then 25°C (room temperature) can be considered. Other temperatures (e.g., 35°C to 37°C for thermally dimorphic organisms) should be reserved for selected fungi that prefer a higher temperature. In general, yeasts are detected within 5 days or less, dermatophytes within 1 week, and dematiaceous and dimorphic fungi between 2–4 weeks. Cultures should be regularly reviewed (e.g., every day the first week, every 2–3 days the second week, twice during the third week, once weekly thereafter) to account for the growth rates and identification of fungi. Incubating cultures for 4 weeks is usually necessary before no growth of fungus should be considered. Several factors influence the length of incubation including the choice of media (e.g., yeasts on chromogenic [48 hours] versus routine media [5–7 days]) and type of fungus suspected (e.g., slow growing dimorphic systemic fungi may need 8 weeks).
Once the organism has been cultured and isolated, the following approach has usually been conducted: (1) determine the morphology of the unknown fungus and determine if it is consistent with any of the groups listed in Table 17-11 or filamentous bacterium (some of the aerobic actinomycetes [e.g., Nocardia] resemble fungi and must be ruled out); and (2) note the rate of growth, colony (macroscopic) and microscopic morphologies of the possible organism(s) (Table 17-12) and refer to necessary textbooks to compare descriptions, drawings, color plates, discussions of characteristics, and other test results to assist in differentiating the likely organism.52,53 In the case of yeasts and yeast-like organisms, additional testing such as the germ tube test, biochemical testing using commercially-available systems, or the urease test may allow species identification of isolates from various body sites.
Antigen Detection
Cell wall components of various invasive fungi have been used as diagnostic markers for antigen testing. Galactomannan is a polysaccharide component of the Aspergillus cell wall that is released by growing hyphae. A commercial enzyme-linked immunosorbent assay (ELISA) (Platelia Aspergillus [Bio-Rad], Marens-La-Coquette, France) is available to detect circulating galactomannan antigen in blood and has been shown to be an earlier diagnostic marker for invasive aspergillosis in neutropenic patients with hematologic malignancies.52 The monitoring of antigen titers has also been shown to correlate with the response to antifungal therapy, patient survival, and autopsy findings in neutropenic patients.
Latex antigen detection and enzyme immunoassay (EIA) are sensitive (93% to 100%) and specific (93% to 100%) diagnostic tests for the detection and quantitation of circulating Cryptococcus neoformans (capsular galactoxylomannan) polysaccharide antigen in serum and CSF.52 Antigen testing is considered to be the primary diagnostic test for screening CSF for suspected cases of cryptococcal meningitis since the India ink procedure has a low sensitivity. The reported titer determinations of the two testing methods (e.g., EIA versus latex testing) or from different commercial latex kits are not numerically similar. Thus, the same testing method and latex kit should be used to monitor serial samples for a patient. False-negative and false-positive (e.g., rheumatoid factor) results have been reported for each testing method.
Enzyme immunoassay can be used to detect Histoplasma capsulatum antigen in body fluids (e.g., blood, urine, CSF or bronchoalveolar lavage [BAL] fluid). It has been recommended that the antigen screening test be validated by antibody testing (e.g., immunodiffusion [ID] and/or complement fixation [CF]).52 The diagnosis of histoplasmosis should be based on a combination of diagnostic test results since antigen testing is associated with cross-reactivity to other fungal infections and the test sensitivity varies with disease presentation (e.g., 77% for acute pulmonary histoplasmosis, 34% for subacute pulmonary histoplasmosis, 21% for chronic pulmonary histoplasmosis, 92% for progressively disseminated histoplasmosis) and specimen type (80% to 95% in urine, 25% to 50% in CSF, 93.5% in BAL). Antigen detection is generally not used as a diagnostic tool for blastomycosis and has a limited role for coccidioidomycosis due to low levels of detection in antigenemia and antigenuria, cross-reactions, and false-positive reactions. Antigen detection tests for Histoplasma capsulatum, Blastomyces dermatitidis, and Coccidioides species are performed by MiraVista Diagnostics (Indianapolis, Indiana) on a fee-for-service basis.
Commercial assays for the detection of (1→3)-beta-D-glucan, a major cell wall component of common pathogenic yeasts, have been used as a panfungal diagnostic tool for invasive fungal infections such as aspergillosis, Fusarium infection, trichosporonosis, and candidiasis.52 In the United States, Fungitell (Glucatell) serum detection test is the only available EIA for detecting (1→3)-beta-D-glucan. The manufacturer’s recommended guidelines for a positive (1→3)-beta-D-glucan value is ≥80 pg/mL. False-positive results have been observed in hemodialysis patients (with cellulose membranes), patients treated with certain blood products (e.g., albumin, immunoglobulins), and patients with bacterial infections or have been exposed to glucan-containing materials (e.g., gauze). Concurrent beta-lactam therapy, such as piperacillin–tazobactam or amoxicillin–clavulanate, and antitumoral polysaccharides have been associated with cross-reactions. This assay is nonspecific and should be used in conjunction with clinical examination of the patient and other diagnostic tests and procedures in order to make a conclusive diagnosis of invasive fungal infection. This diagnostic assay is not useful for mucoraceous molds (e.g., Zygomycetes, Rhizopus), which do not produce (1→3)-beta-D-glucan), or Cryptococcus species and Blastomyces dermatitidis because they produce only low levels of (1→3)-beta-D-glucan).
Antigen detection methods and serology for Candida spp. in blood cultures have not been reliable in distinguishing between colonization, candidemia, and disseminated candidiasis.52 Extreme variability has been observed in both sensitivity and specificity, making the currently available tests unreliable for establishing a diagnosis. Several commercial kits are available for the detection of capsular galactoxylomannan (cryptococcal antigen) and are able to detect Cryptococcus neoformans and Cryptococcus gattii. The combination of antigen detection test and an India ink stain of the CSF are recommended for the primary evaluations of suspected cases of cryptococcal meningitis. False-positive and false-negative results have been reported with the various methodologies.
Serology
Several different methodologies for antibody testing (e.g., tube precipitin [TP], CF assays, ID, LA, and EIA) have been investigated for the detection of specific fungal pathogens.52 Interpretation of serology results for most fungal infections requires knowledge of the laboratory technique used to perform the antibody testing. Serologic assays are most useful as diagnostic testing of fungal infections in the immunocompetent host since a poor antibody response is common in immunosuppressed patients resulting in a false-negative result.
The presence of antibody has assisted in the diagnosis of invasive infections such as coccidioidomycosis, histoplasmosis, and paracoccidiodomycosis.52 The most reliable serological tests for diagnosing coccidioidomycosis have been ID and CF, where heated and/or unheated coccidioidin is used as the principal antigen in these tests. Serologic tests (ID, CF, and LA) for the clinical diagnosis of infections caused by Histoplasma capsulatum are commercially available. These tests have been the most useful in patients with chronic pulmonary or disseminated histoplasmosis. Immunodiffusion and CF are the most common serologic methods used for the diagnosis of paracoccidioidomycosis. No commercial kits for either method are available (fee-for-service, Cerodex Laboratories, Washington, OK). Finally, serology testing has been useful for the diagnosis of noninvasive diseases such as allergic bronchopulmonary aspergillosis and aspergilloma.52
Molecular Diagnosis
Currently there are a limited number of molecular diagnostic tests available for the detection and identification of fungi in the clinical laboratory.52,53 For fungal isolates grown in pure culture, NA hybridization probes, DNA sequencing, peptide NA fluorescence in situ hybridization probes, and laboratory-developed PCR tests are available. Molecular methods used in the direct detection and identification of fungi from patient specimens are limited to a few laboratory-developed PCR tests targeting specific fungal agents. A commercially-available platform (Luminex xMAP, Luminex, Austin, TX) with PCR amplification, flow cytometry, and dual-laser system is available for high throughput and species-specific identification with user-designed (or outside vendor) probes. For laboratory-developed methods, proper validation of NA tests is needed before routine use can occur in the clinical laboratory. Clinicians will need to contact their laboratory to determine which tests are available and which molecular diagnostic tests may need to be sent to a reference laboratory. At this time, a combination of morphologic and molecular testing methods is best used for species identification.
Several probe-based assays have become commercially available for the identification of dimorphic fungi and Candida spp.52,53 AccuProbe (Gen-Probe, San Diego, CA) uses hybridization to target rRNA present in a fungal culture, which is detected by a labeled single-stranded DNA probe. Three separate probes, with high sensitivity and specificity, are approved by the Food and Drug Administration (FDA) and available for the identification of Blastomyces dermatitidis, Coccidioides immitis, and Histoplasma capsulatum. The Blastomyces dermatitidis probe has the potential to cross-react with other fungi including Emmonsia species, Paracoccidiodes brasiliensis, and Gymnascella spp. In addition, the Coccidioides probe is unable to distinguish between species, namely Coccidioides immitis and Coccidioides posadasii.
Peptide nucleic acid-fluorescence in situ hybridization (PNA FISH Yeast Traffic Light Probe, AdvanDx, Woburn, MA) is available for the direct identification of Candida spp. on blood smears from cultures that are Gram stain positive for yeasts.53 After the Gram stain and the hybridization process is completed, Candida albicans and Candida parapsilosis are identified microscopically as bright green fluorescing cells, while Candida tropicalis fluoresces bright yellow, and Candida glabrata and Candida krusei fluoresce bright red. Other yeasts do not fluoresce. The colors of the light probes also provides an indication about the potential use of fluconazole in these patients since Candida albicans and Candida parapsilosis are generally susceptible to fluconazole (green light for go), Candida glabrata can be resistant to fluconazole and Candida krusei is intrinsically resistant to fluconazole (red light for stop). The yellow signal produced by Candida tropicalis indicates that caution should be used since fluconazole susceptibility is variable for this organism. This method has a significant impact over traditional identification methods, which could take up to three or more days for identification of Candida spp., as well as guiding the most effective antifungal drug therapy.
MALDI-TOF Mass Spectrometry
Matrix-assisted laser desorption ionization time-of-flight (MALDI-TOF) mass spectrometry (MS) has been shown to be a rapid and accurate method for identifying yeasts and molds recovered on culture media.52–54 Reports on the use of MALDI-TOF MS for routine rapid identification have focused on clinically important yeasts (e.g., Candida spp., Cryptococcus neoformans) and dermatophyte species. Filamentous fungi have been more difficult because of different developmental forms on agar media and the influences of the phenotype. MALDI-TOF MS is likely to become the primary diagnostic method for rapid identification of fungus isolates in the clinical microbiology laboratory. This technology is ideal for genus and species identification, and has the potential for accurate strain typing and identification for fungi, bacteria, and mycobacterium.
The advantages of the MALDI-TOF MS for fungal identification is the low cost of materials (a few cents) for each organism identification and the rapidity to results (approximately 11 minutes if just one isolate is tested; 2.5 minutes per isolate in a batch of 96 isolates, with the average time per isolate in published reports being 4–6 minutes). However, the current limitations include the initial costs of instrumentation for the system, lack of sample preparation techniques, inadequate fungal spectra in the database and software of commercial systems (e.g., Bruker and Shimadzu), and lack of FDA approval of any currently available system. Expansion of database libraries and developments in sample preparation are rapidly occurring to establish validated and routine procedures for a large number of clinically important fungal strains and species. Evidence from several studies also suggests that MALDI-TOF MS could be developed for performing rapid antifungal susceptibility testing.
Antifungal Susceptibility Testing
The importance of antifungal susceptibility testing has become increasingly recognized as a useful component in the treatment optimization of invasive infections caused by Candida spp. because of the increasing number of available antifungal agents, emerging resistance issues to standard therapy, and the changing epidemiology of invasive fungal disease. The CLSI has developed standardized reference methods for macrodilution and microdilution susceptibility testing of yeasts and molds, as well as disk diffusion methods of yeasts and nondermatophyte filamentous fungi.55–58 The commercial availability of simplified and/or automated testing methods (e.g., Etest strip; Vitek 2; Sensititre YeastOne) consistent with CLSI reference methods is allowing an increasing number of clinical laboratories to routinely perform antifungal susceptibility testing.
Interpretive MIC breakpoints based on CLSI-recommended in vitro susceptibility testing methods have been recommended for Candida spp.58 The results of several recent comprehensive reviews regarding the microbiological, molecular, pharmacodynamic, and clinical antifungal data for Candida spp. has been recently reviewed, and species-specific interpretive clinical breakpoints have been proposed for fluconazole, voriconazole, and the echinocandins (Table 17-15).59–61 These recent reports have also been useful for the establishment of epidemiologic cutoff values, detection of emerging resistance among Candida spp., and harmonization of antifungal susceptibility testing standards by CLSI and European Committee on Antimicrobial Susceptibility Testing (EUCAST).59–61 Interpretive breakpoint criteria for other fungal pathogens remain to be standardized.
I = intermediate; R = resistant.
VIRUSES
There are approximately 650 viruses that are known to cause infection in humans and other vertebrate animals.62 The three major properties that classify viruses into families include (1) the NA core (either DNA or ribonucleic acid [RNA], but not both); (2) whether the viral NA is single- or double-stranded; and (3) the presence or absence of a lipoprotein envelope (Tables 17-16 and 17-17).62-64 Virus families can be further categorized on the basis of morphology (e.g., size, shape, and substructure), mode of replication, and molecular and genomic characteristics. The most recent information on the rapidly changing classification and taxonomy of viruses can be obtained from the website database (www.ncbi.nlm.nih.gov/ICTVdb/) that has been established by The International Committee on Taxonomy of Viruses (ICTV). The 2011 ICTV report now recognizes five hierarchical ranks consisting of six orders, 94 families, 22 subfamilies, 395 genera, and 2475 species of viruses; however, over 3000 viruses remain unclassified.
(-) = negative stranded; CMV = cytomegalovirus; EBV = Epstein-Barr virus, EM = electron microscopy; dsDNA, double-stranded DNA; HAV = hepatitis A virus; HBV = hepatitis B virus; HPV = human papillomavirus; HSV = herpes simplex virus; IA = immunoassay; NA = nucleic acid; ssDNA = single-stranded DNA; VZV = varicella-zoster virus.
aCommonly used methods in clinical laboratories: Immunoassays (including immunofluorescence assay (IFA); enzyme-linked immunosorbent assay (ELISA), and immunochromatographic assay (ICA).
bThe isolation of some pathogens (e.g., Smallpox) requires biosafety level (BSL) 3 or 4 facilities, usually only in specialized centers collaborating with World Health Organization. The isolation of Vaccinia virus requires BSL-2 (grows readily in cell culture).
(+) = positive stranded; (-) = negative stranded; BSL = biosafety level; CSF = cerebrospinal fluid; dsRNA = double-stranded RNA; EM = electron microscopy; HAV = hepatitis A virus; HCV = hepatitis C virus; HDV = hepatitis D virus; HEV = hepatitis E virus; HIV = human immunodeficiency virus; IA = immunoassay; LA = latex agglutination; NA = nucleic acid; ssRNA = single-stranded RNA; RSV = respiratory syncytial virus.
aCommonly used methods in clinical laboratories: Immunoassays, including immunofluorescence assay (IFA); enzyme-linked immunosorbent assay (ELISA), and immunochromatographic assay (ICA).
The Identification of Viruses
The ability to detect and accurately identify viruses in the clinical laboratory has increased during the last 30 years as a result of wider applicability of diagnostic laboratory techniques with increased sensitivity and decreased turnaround time, the availability of newer reagents and rapid commercial diagnostic kits, and the addition of new antiviral drugs for specific viral infections.64–66 In addition, the improvements in cell cultures and increased availability of viral antigen and nucleic-acid hybridization and amplification-based tests (including real-time PCR) are allowing diagnostic virology laboratories to provide clinical services for the increasing frequency of infectious diseases that depend on viral diagnosis.66,67
It is important to note that all diagnostic tests for the identification of viruses are not available at each institution, and the clinician will need to establish a relationship with the laboratory that will be performing viral testing. In certain clinical situations, samples may need to be sent out for diagnostic testing at either large reference or public health laboratories since they are able to provide the necessary methods that are difficult or impossible to routinely perform in the clinical virology laboratory. In addition, certain viruses (e.g., arboviruses, arenaviruses, filiviruses, Variola virus, and rabies virus) require testing at biosafety level (BSL) 3 or 4 facilities and are often sent to the Centers for Disease Control and Prevention (CDC) or the CDC’s Division of Vector-Borne Infectious Diseases.64
The ability to accurately diagnose a viral infection is highly dependent on appropriate selection, timing, collection, and handling of biological specimens.63,65 In general, the highest titers of viruses are present early in the course of illness and decrease as the duration of illness increases. Therefore, it is very important to collect specimens for the detection of viruses early in the course of an infection. In most cases, identification of viruses is a specimen-driven process. Since collection procedures are highly dependent on viruses being suspected, attention needs to be taken regarding collection containers and devices as well as transport systems (e.g., whether a viral transport medium is needed). The different types of clinical specimens that can be collected for viral culture and antigen detection include respiratory specimens (e.g., nasopharyngeal swabs, aspirates, and washes; throat swabs; BAL and bronchial washes), blood, bone marrow, CSF, stool, biopsy tissue, urine, ocular specimens, vesicles and other skin lesions, and amniotic fluid. In addition, specimens for molecular diagnostic testing (e.g., PCR and other nucleic amplification techniques) must be obtained following specific guidelines so that the stability and amplifiability of the nucleic acids are ensured.
Once the sample is collected, it should be promptly transported to the laboratory in a sterile, leak-proof container using the appropriate viral transport media to maximize viral recovery. Every effort should be made to prevent delay between the time of specimen collection and its arrival to the laboratory. When delays are expected, viral samples should be refrigerated at 4°C or frozen at –70°C. Subsequently, the laboratory will need to follow specific processing procedures for each specimen and the different diagnostic viral test methodologies.
The laboratory techniques used in the diagnosis of viral infections include cell culture, cytology and histology, electron microscopy (EM), antigen detection, NA detection, and serologic testing.64–67 The choice of test(s) will vary depending on the clinical syndrome or disease, virus(es) involved, patient characteristics, collection site, purposes of the test (e.g., screening, diagnosis, confirmation or monitoring), time to result, laboratory capabilities/staff expertise, and cost. The following section, as well as Tables 17-16 and 17-17, provide a brief summary of the common methods currently used in diagnostic testing of common viruses.64–67 For more detailed information, the reader is referred to current published literature, standard reference books, and the latest edition of reference manuals (e.g., Manual of Clinical Microbiology, American Society for Microbiology Press).
Cell Culture
The use of cell culture rapidly expanded the knowledge about the epidemiology, clinical characteristics, and diagnosis of common viral infections in the 1950s and 1960s. Subsequently, the use of cell cultures to isolate a virus became the gold standard method for the diagnosis of viral infections in most clinical virology laboratories.64,67 The advantages of cell culture include good specificity and sensitivity, the capability of detecting multiple viruses if present, and the cultivation of the virus for further laboratory testing (e.g., susceptibility testing, serologic strain typing), if needed. Cell cultures can be useful when combined with highly specific monoclonal antibodies (MAbs), if the cost of other testing methods are greater than cell cultures or when the clinical laboratory does not have the ability and/or equipment to perform molecular detection methods. The disadvantages of cell culture include the long time needed for the detection of viruses using conventional cell culture (e.g., days to weeks), the need for cell culture facilities, the expense of performing cell culture, and the methodology is not applicable to all viruses (e.g., some viruses have not been successfully grown in cultures). The technical demand that cell cultures place on the diagnostic virology laboratory is being challenged by the rapid evolution of antigen screening assays and NA amplification tests.
There are several different types of cell culture that are available to grow clinically important viruses.65,67 A cell line can be established once a cell culture has been subcultured in vitro. The different types of cell lines can be divided into three categories: primary, diploid (also called low passage cell lines), and heteroploid. Primary cell lines (e.g., rhesus monkey kidney [RhMK] cells or human amnion cells) are prepared from animal or human tissues and can withstand only one or two passages until the cells die. Diploid cell lines are usually derived from fetal or newborn cells (e.g., human embryonic lung fibroblast lines such as WI-38 or MRC-5) and can undergo 20 to 50 passages before cells are unable to survive. Continuous cell lines can undergo an indefinite number of passages without reducing the sensitivity to virus infection. Heteroploid cell lines are characteristically derived from human or animal cancers (e.g., human epidermoid lung carcinoma [HEp-2, HeLa]) or are cells transformed in vitro (e.g., LLC-MK2). Heteroploid cell lines can also include genetically engineered cells (e.g., ELVIS cell mixture for the detection of HSV types 1 and 2). Most specimens are inoculated onto two or more cell lines (e.g., RhMK, MRC-5, HEp-2) based on the most likely viruses associated with the type of clinical specimen that was submitted.
The growth of a virus from a clinical specimen provides direct evidence that the patient was infected with a virus. The main method for detecting growth from the cell culture method is by microscopic examination of the unstained cell cultured monolayers for morphological changes or cytopathic effect (CPE).65–67 The characteristics of the CPE (e.g., which cell culture types were affected; what is the resultant shape of the cells; whether the effect is focal or diffuse; the time of its appearance and progression) can be used for primary and/or definitive identification of the virus. Subsequently, fluorescent antibody (FA) staining of cells with virus-specific MAbs harvested from the culture is often used to confirm the identification of the virus. Molecular or ancillary traditional testing can alternatively be used for viral identification.
Some viruses, such as influenza, parainfluenza, and mumps virus, will grow in cell cultures without producing CPE so that other methods are used to identify and detect these viruses, including hemadsorption and interference.65–67 Hemadsorption is used to detect these viruses, which can grow rapidly and reach high titers in cell cultures without producing CPE. Hemadsorption involves the removal of the culture medium from the inoculated cell culture, adding a suspension of erythrocytes and examining for hemadsorption with a low-power microscope as manifested by adherence of the red cells to the cell culture monolayer due to the presence of a hemadsorbing virus. Used to detect viruses such as rubella, interference involves growing a virus that yields a cell culture resistant to other viruses (to which it is normally susceptible). The viruses that produce hemadsorption or interference subsequently can be identified by staining with virus-specific monoclonal antibodies or antiserum.
Shell vial with centrifugation and pre-CPE detection are used to decrease the amount of time required to grow a virus by conventional cell cultures.65–67 This technique makes use of cells grown on microscope coverslips that are placed within shell vials and covered with culture media. After cultures are incubated for 1–3 days, FA staining is performed on the cells on the coverslips to recognize an antigen in the nucleus of infected cells. Shell vial cultures have been commonly applied for the detection of cytomegalovirus (CMV), HSV, varicella-zoster virus (VZV), enteroviruses, and the human respiratory viruses. Centrifugation-enhanced rapid cell cultures can also be used with co-cultivated cells (e.g., mixture of two cell lines together) or genetically engineered cells (e.g., ELVIS [enzyme-linked virus-inducible system], BGMK-hDAF [buffalo green monkey kidney cell line]) for the rapid identification (e.g., 16 to 72 hours) and blind staining of multiple viruses from a single shell vial or tray well.66,67
Cytology and Histology
Cytologic examination can be performed on smears prepared from samples that are applied to a microscope slide or “touch preps” of unfixed tissues.65 Cytologic findings are suggestive of a viral infection and provide identification of cell morphologies (e.g., “owl’s eye” nuclear inclusions consistent with CMV). The specific virus cannot be identified unless virus-specific immunostaining techniques are used. Applications of cytology to viral diagnosis include the Tzanck smear with Giemsa reagent for demonstrating the presence of HSV or VZV infection, Papanicolaou staining of cells obtained from the uterine cervix (Pap smear) for providing evidence of human papillomavirus (HPV) infection, and cytologic staining of urinary sediments for screening the presence of either CMV or polyomaviruses JCV and BKV.
Similar to cytology, histologic examination of tissue provides evidence to suggest a group of viruses that may be causing infection, but it does not identify a specific virus.64,66 Despite this shortcoming, histopathology has been useful in differentiating between asymptomatic viral shedding and clinically important infections of CMV and has been used for the diagnosis of CMV infections in tissue samples obtained from biopsy or at autopsy. In addition, detection of specific viral antigens by immunohistochemistry (IHC) and detection of specific viral nucleic acids by in situ hybridization (ISH) has allowed specific viruses to be identified by histopathology.
Electron Microscopy
Viruses are the smallest infectious pathogens that range in diameter from 18–300 nm.65 Direct visualization of a virus with a light microscope can only be performed on pathogens with a diameter greater than 200 nm. The electron microscopy (EM) allows visualization of characteristic viral morphology, and unlike direct detection or molecular methodologies, is capable of detecting the distinctive appearances of multiple viruses, if present.65 Electron microscopy is considered the most useful routine test for poxviruses.66 Diagnostic virology laboratories also commonly use EM for detection of viruses that are not detected with cell cultures or other methods (e.g., gastroenteritis viruses such as noroviruses, coronaviruses, astroviruses, enteric adenovirus, and calicivirus).64,66
Several techniques have been incorporated to allow the visualization of viruses with EM from various types of clinical specimens. Negative staining is a technique for identification of viruses in fluid samples, stool samples, and blister fluid. Thin sectioning can be performed on tissue samples that have been fixed with specific fixatives for EM study, and it can be used to visualize the herpesviruses, respiratory viruses, and rabies virus.
More sensitive methods are replacing the routine use of EM for detecting clinically significant viruses.64,66 The advantages of EM include its economical, quick (e.g., same day), adaptable, and straightforward approach for detecting viruses. The major disadvantages of EM include poor sensitivity, initial equipment expenses, and the need of highly skilled laboratory staff.
Direct Antigen Detection
Antigen detection methods involve the use of virus-specific antibodies directed toward viral antigens in a clinical specimen.59 Examples of viruses that can be identified by direct antigen detection include respiratory syncytial virus (RSV), influenza virus, parainfluenza virus, adenovirus, HSV, VZV, CMV, rotavirus, hepatitis B virus (HBV), and measles virus.64,66 The advantages of direct antigen detection include the rapidity of diagnosis (e.g., hours to 1 day), the usefulness for the identification of viruses that are difficult to culture, and the detection of viral specific antigens even if viable virus is not present in the clinical specimen. The disadvantages include the potential for false-positive and false-negative results, the difficulty of performing batch testing, and the lack of sensitivity necessary for diagnostic applications for all viruses (e.g., not applicable for rhinoviruses since there are more than 90 serotypes and cross-reacting antibodies).
The techniques commonly used for antigen detection include immunofluorescence assay (IFA; direct and indirect), EIA (including ELISA), chemiluminescent and fluorescence-based immunoassay, and particle agglutination assays. Several membrane immunochromatographic assays (ICAs; dipstick tests) are available as influenza diagnostic tests (e.g., Directigen Flu A or A+B Test, QuickVue influenza, Directigen Flu A+B). These viral antigen tests have become simple to use and allow rapid detection (within minutes) of specific antigens from a single specimen in the clinical laboratory and at the point of care (e.g., outpatient facilities, physician offices, patient’s bedside). A recent systematic review found rapid influenza diagnostic tests (RIDTs) to have high specificity but moderate and variable sensitivity (higher in children and for detecting influenza A).68 The need for improved sensitivity of RIDTs was also observed during the 2009 pandemic of H1N1 influenza.
Serology
Serologic tests are designed to detect an antibody response in serum samples after exposure to viral antigens has occurred.65 The major uses of serology for the detection of viral infections include the demonstration of immunity or exposure to a virus, the diagnosis of postinfectious sequelae, and the screening of blood products. In several clinical situations, serologic testing remains the primary means for the laboratory diagnosis of viruses that are difficult to culture or detect by direct methods (e.g., rubella virus, Epstein-Barr virus [EBV], hepatitis viruses, HIV, arboviruses).64,66 Serologic testing may also serve as a supportive or adjunctive role in clinical situations where viral cultures or direct detection methods are available.
For viral infections, serologic testing can (1) identify the virus; (2) distinguish the strain or serotype; (3) differentiate between primary infection and reinfection; and (4) determine if the infection is in an acute or convalescent phase. Virus-specific immunoglobulin antibodies (e.g., IgM or IgG) are produced during the time course of a viral infection. In general, virus-specific IgM is detected in serum sooner than virus-specific IgG. The results measure the relative concentration of antibody in the body as a titer, with the titer representing the lowest antibody concentration (or inverse of the greatest dilution; a dilution of 1:128 is expressed as a titer of 128) that demonstrates activity in a patient’s serum. The exact value for a titer varies with each testing method, the specific virus involved, the timing of specimen collection, and the presence of active disease.
For most viral infections, virus-specific IgM can be detected as soon as 3–7 days after the onset of infection. The presence of virus-specific IgM in a single serum sample shortly after the onset of symptoms (acute phase) is usually indicative of a very recent or current primary infection. Titers of virus-specific IgM usually decline to near undetectable amounts within 1–4 months after the onset of infection. Virus-specific IgG can be detected during the acute phase of infection (e.g., 1–2 weeks) and will continue to increase for several months before reaching a maximal titer. Thereafter, the IgG titer will decline, but it usually remains detectable in serum for the remainder of a person’s life. Seroconversion has occurred when at least a fourfold increase in IgG titer has occurred between serum samples collected in the acute and convalescent (2–4 weeks afterward) phases. The presence of virus-specific IgG is also indicative of a past infection.
Serologic tests are also used to assess the immunity or exposure to a virus. The presence of antibody can detect which patients have been previously infected by or vaccinated for a specific virus. For example, a positive result (presence of antibody) for rubella in a woman of childbearing age implies that congenital infection will not occur during subsequent pregnancies. A negative result (absence of antibody) implies susceptibility to infection, and the woman should receive rubella vaccination if she is not pregnant. Some other examples of viruses for which serologic determination of immune status is useful include hepatitis A and B (HAV, HBV), measles, mumps, parvovirus B19, and VZV.65,66
The techniques commonly used for serologic assays include CF, EIA, IFA, anticomplement immunofluorescence, and Western immunoblotting. In the diagnosis of certain viral syndromes (e.g., central nervous system [CNS] infections), a serology panel may be helpful so that a battery of antigens is tested for antibody to several viruses. The advantages of viral serology include the assessment of immunity or response of a virus isolated from a nonsterile site, serum specimens are easy to obtain and store, and it can be used to identify viruses that are difficult to culture or detect by immunoassay. The disadvantages include the time to results (e.g., few days to weeks), the potential for cross-reactions between different viruses, and the need for both acute and convalescent specimens.
Molecular Diagnosis
The detection of specific viral nucleic acids (NAs) by molecular diagnostic techniques is revolutionizing the field of diagnostic virology.64-67 Molecular methods are rapidly becoming the “gold standard” in clinical virology laboratories and will likely replace older techniques such as cell cultures for detecting clinically significant viruses. The techniques used in viral NA detection include direct hybridization assays, target (template) amplification (e.g., PCR, self-sustained sequence replication [3SR] method, strand displacement amplification [SDA]), and signal amplification (e.g., branched-chain DNA [bDNA] assay and hybrid capture assay). Among these, PCR has been the most important technique in diagnostic virology because of its versatility in detecting DNA or RNA, as well as being able to provide qualitative and quantitative information on specific viral nucleic acids.
The use of NA detection has become the standard of care (e.g., HCV and HIV) or the test of choice for routine diagnosis of many viral infections (e.g., bocaviruses, HSV CNS infections, human HVS 6 and 7, human metapneumovirus, HPV).64,66 The FDA has cleared or approved commercial molecular detection assays, several viruses including hepatitis B and C viruses (HBV, HCV), HIV, HSV, CMV, adenovirus, avian flu, enteroviruses, influenza, and HPV. An FDA-approved multiplex PCR kit (e.g., xTAG Respiratory Viral Panel) is also available for rapidly screening 12 common respiratory viruses (e.g., RSV, influenza A and B, adenovirus) or subtypes. An up-to-date listing of FDA-cleared/approved molecular diagnostics tests is available on the website of the Association of Molecular Pathology (www.amp.org).
The advantages of viral NA detection methods include the rapidity of results (e.g., hours for real-time PCR and one to several days for other methods), maximal sensitivity for virus-specific detection and identification, adequate to excellent specificity, increasing availability of commercial assays, the ability to detect viruses that are difficult to culture, and the ability to detect nucleic acids without viable virus present in the clinical specimen. The disadvantages of these techniques include the need for molecular facilities for selected tests, labor-intense methodology, and a potential for PCR contamination that may lead to false-positive results. Molecular assays are rapidly becoming the standard of care for diagnosing viral infections as well as monitoring antiviral therapy and patient outcomes.64,66
Antiviral Susceptibility Testing
The emergence of drug-resistant strains of viruses to antiviral agents is an increasing problem, especially in immunocompromised hosts. Unlike antibiotics, in vitro susceptibility testing of viruses has not been routinely available. The major variables that have limited the standardization of antiviral susceptibility testing include cell lines, inoculums titer, incubation period, testing range of antiviral drug concentrations, reference strains, assay methodology, and criteria, calculation, and interpretation of end-points.69
Susceptibility testing has been performed by phenotypic and/or genotypic assays for HIV-1, HBV, HSV, VZV, CMV, and influenza viruses.69 The CLSI has published an approved standard for phenotypic susceptibility testing of HSV.70 This standard outlines the use of a plaque reduction assay and denotes resistance to acyclovir and foscarnet when IC50 values are ≥2 mcg/mL and ≥100 mcg/mL, respectively. Interpretation of these values must be carefully made in conjunction with the clinical response of the individual patient. Other consensus documents for antiviral susceptibility testing are needed.
HUMAN IMMUNODEFICIENCY VIRUS
Human immunodeficiency virus (HIV) is the causative agent of AIDS. The HIV virus is an enveloped, positively-stranded RNA virus that belongs to the Retroviridae (retrovirus) family and Lentivirus genus. The mature virus measures approximately 100 nm in diameter and has a characteristic conical core containing proteins, enzymes, and two identical copies of single-stranded RNA. Viral proteins within the core and the lipid envelope play a significant role in the detection, diagnosis, and treatment of HIV.71,72 The replication process of HIV involves transcription of viral RNA into proviral DNA using the reverse transcriptase (RT) enzyme. The proviral DNA is then integrated into the host’s genome using the integrase enzyme, resulting in lifelong latent infection. The virus is transmitted to humans by the exchange of blood or other body fluids containing the virus through sexual contact; exposure to contaminated blood; transfusion of contaminated blood and/or blood products; or via contaminated needles (e.g., intravenous drug abusers or accidental needle sticks). In addition, infants can acquire HIV from an infected mother in utero, during labor or delivery, or during breast-feeding.71,73
There are two distinct serotypes of HIV, namely HIV-1 and HIV-2; while HIV-1 is the most prevalent serotype of HIV infections worldwide, HIV-2 infection is most commonly distributed in Western Africa and other limited geographic locations.71–73 Routine diagnostic testing of HIV-2 is not recommended in the United States since its prevalence is extremely low. Thus, the following discussion will focus mainly on laboratory tests used for the diagnosis and management of HIV-1 infection. However, HIV-2 testing may be indicated in persons at risk for HIV-2 infection or for those who have symptoms suggestive of HIV infection with negative or indeterminate test results for HIV-1. In addition, all blood donations in the United States are tested for both HIV-1 and HIV-2.71–73
Laboratory Tests for HIV-1 Infection
Several laboratory tests are available for the diagnosis and monitoring of patients with HIV-1 infection. The most common virologic testing methods include HIV-1 antibody assays, HIV-1 p24 antigen assays, DNA-PCR, plasma HIV-1 RNA (viral load) assays, and viral phenotypic and genotypic assays. In addition, the absolute number of CD4+ lymphocytes and the ratio of helper (CD4+) to suppressor (CD8+) lymphocytes (CD4+:CD8+ ratios) are routinely measured to evaluate the patient’s immune status and response to antiretroviral therapy, since HIV primarily infects and depletes CD4+ T helper lymphocytes. Viral cultures for HIV are not typically performed beyond clinical research studies due to the labor-intensive nature of the testing methods as well as the extensive time required to obtain results.63–65
Laboratory tests for HIV-1 infection are clinically utilized for (1) diagnosing HIV-1 infection; (2) monitoring progression of HIV infection and the response to antiretroviral therapy; and (3) screening blood donors. The selection of these tests is highly dependent on the clinical situation, the patient population, and the specified purpose for the testing, as described in Table 17-18.73–77 (See Minicase 4.) The following section briefly reviews each of the specific tests. Detailed descriptions of the various commercial assays and their clinical applications have been recently reviewed elsewhere.71,73,74,78
DNA = deoxyribonucleic acid; ELISA = enzyme-linked immunosorbent assay; HIV = human immunodeficiency virus;
RNA = ribonucleic acid; PCR = polymerase chain reaction; WB = western blot.
Testing for HIV After Occupational Exposure
JAMES D. IS A NURSE IN THE EMERGENCY DEPARTMENT who is currently finishing up his overnight shift. He is drawing blood from an unresponsive patient during an emergency and he accidentally sticks himself with a used large bore needle before the patient is transported to a different hospital. Because of this exposure, James D. is instructed to immediately seek attention at Employee Health to obtain testing for HIV and HCV. The HIV and hepatitis C status of the patient is unknown. James D. is advised by the physician at Employee Health to submit a blood sample for immediate testing, as well as to return at regular intervals during the next 6 months for additional testing.
Question: How long will James D. have to wait before he can be certain that he did not acquire HIV from the exposure?
Discussion: The hallmark tests for diagnosing HIV infection include the ELISA (or EIA) and a confirmatory western blot. The ELISA test detects the presence of anti-HIV antibodies, which may take several weeks to months before they appear in the blood of a newly infected patient. The HIV status of the source patient is unknown. Therefore, if possible, the source patient should be questioned regarding risk factors that may predispose to HIV infection, as well as undergo HIV testing (rapid screen and ELISA). Even if the source patient is currently HIV negative by ELISA, James D. will need to undergo testing for at least 6 months before HIV infection can be ruled out.
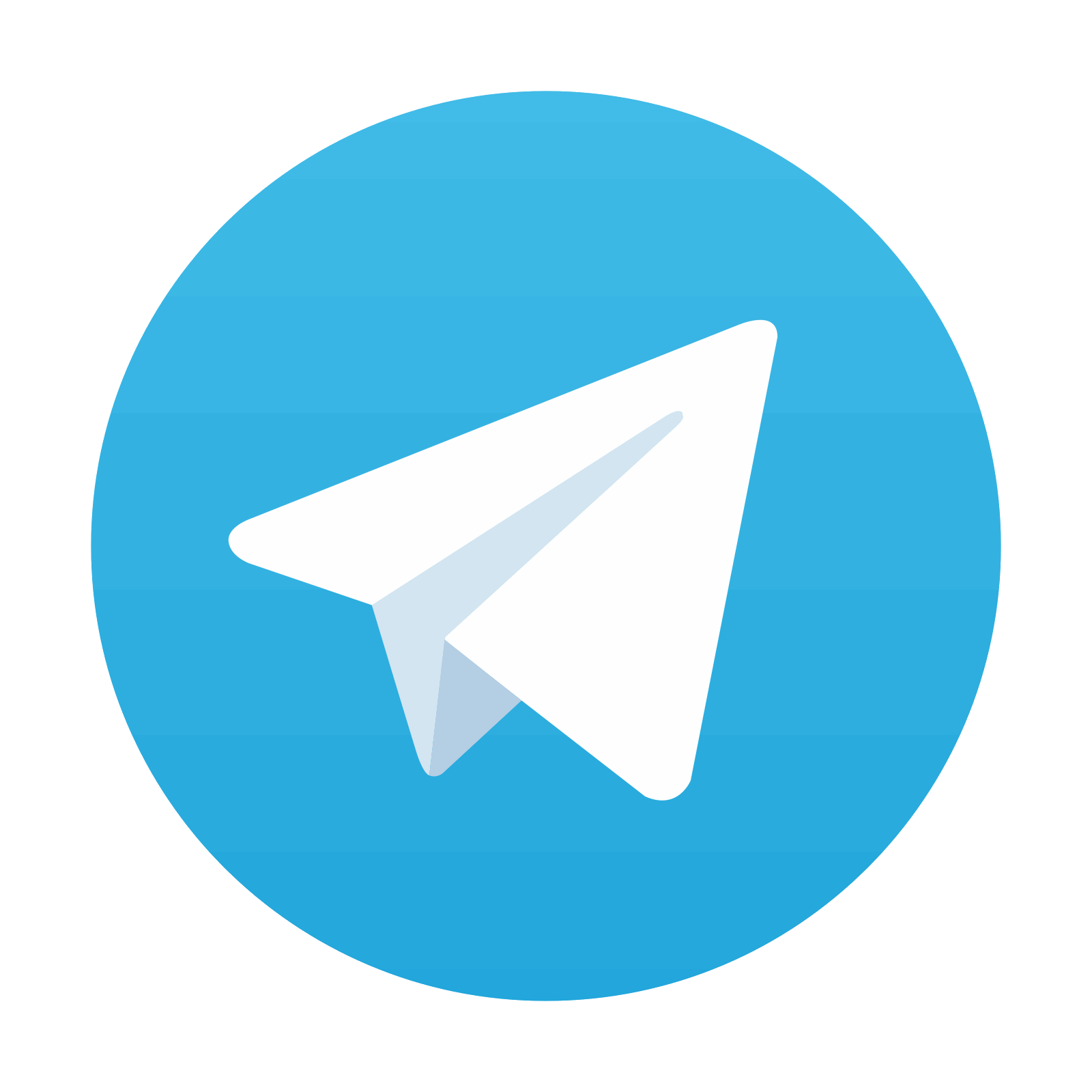
Stay updated, free articles. Join our Telegram channel

Full access? Get Clinical Tree
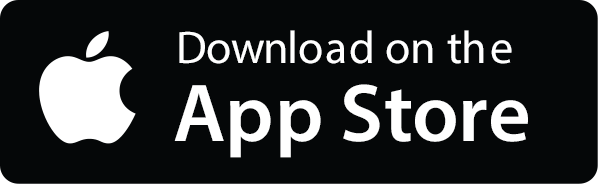
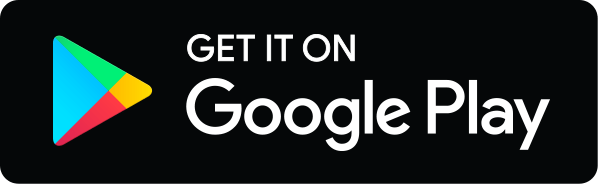