Importance and Processes of Cleaning
Rizwan Sharnez
The purpose of cleaning a device or equipment is to render it suitable for further processing or for its intended use. From a regulatory and an end-user perspective, surfaces that are reusable should be free of visible residue, and microbial and chemical contaminants should be reduced to acceptable levels. The acceptable level of a contaminant depends on the purpose of the subsequent operation. For example, if cleaning is followed by disinfection or sterilization, it may be important for some applications that the bioburden (population of viable microorganisms) after cleaning should be less than the acceptable microbial load for the subsequent antimicrobial step. This criterion, an acceptance limit for postcleaning bioburden, is important in many environmental, manufacturing, and health care situations, such as in cleanrooms, pharmaceutical operations, food processing, and in health care facilities, where surface contamination with pathogens can pose an infection risk to humans. The ability of the cleaning operation to reduce contaminants to an acceptable level is also important in the preparation of newly manufactured and reusable medical devices prior to disinfection and sterilization. In addition to the requirements of the subsequent operation, storage conditions that affect microbial growth, such as time, temperature, moisture, and residual nutrients, are also important to consider when setting an acceptance limit for microbial contaminants.
Acceptance limits for chemical contaminants in many applications are derived from toxicological data. The data are used to derive an acceptable daily exposure (ADE) for the contaminant.1,2,3,4 The ADE of a substance is the largest dose a subject can be exposed to per day for a lifetime by any route of administration without any observable adverse effects. The lower the ADE of the contaminant, the lower its acceptance limit, and therefore, the more sensitive the analytical method needs to be to verify that the contaminant is reduced to an acceptable level.
Initially, cleaning mechanisms, methods, processes, and equipment design considerations are discussed. The effect of cleaning parameters, clean hold time (CHT), and storage conditions on microbial growth and the formation of biofilms are then considered. Later in the chapter, cleaning practices for preventing corrosion and microbial contamination and a first principles approach for setting rational alert and action limits for postcleaning bioburden and chemical residues are described. The cleaning of reusable devices and clinical surfaces is further discussed in chapter 47.

The development and optimization of a cleaning process requires an understanding of cleaning methods and mechanisms, the interaction between chemical and transport processes, and the physicochemical properties of the soil and the surface. These elements of cleaning and cleaning process and equipment design considerations are discussed in this section.
Cleaning Methods and Processes
In industrial applications, cleaning methods and processes fall into two broad categories: clean-in-place and clean-out-of-place. Cleaning can also be categorized as automated, as in washer-disinfectors (see chapter 47) or manual, which typically involves wiping or scrubbing in a sink with the cleaning solution or solvent—hereafter referred to as the cleaning fluid.
Clean-in-place
Clean-in-place (CIP) is a widely used method of automated cleaning that is performed directly within manufacturing equipment with little or no equipment disassembly. It typically consists of a programmable
cleaning cycle with multiple steps. In each step, a specific cleaning fluid (that may be a solvent such as water or a solution of various cleaning chemicals) is continuously circulated through the system at a controlled flow rate, temperature and cleaning agent concentration, and for a set duration. CIP is widely used in the food, dairy, beverage, brewing, pharmaceutical, and cosmetic industries to clean large fixed equipment, such as fermenters, bioreactors, centrifuges, heat exchangers, tanks, and piping. Vessels and other large equipment are cleaned by spraying the cleaning fluid on product contact surfaces, thereby creating a falling film of fluid on the surface. Piping and associated components, such as valves and pumps, are cleaned by flooding them with the cleaning fluid. Advantages of CIP systems include low labor requirement, fast equipment turnaround, and consistency. High initial investment and equipment maintenance costs and relatively high usage of water, chemicals, and energy are the main drawbacks.
cleaning cycle with multiple steps. In each step, a specific cleaning fluid (that may be a solvent such as water or a solution of various cleaning chemicals) is continuously circulated through the system at a controlled flow rate, temperature and cleaning agent concentration, and for a set duration. CIP is widely used in the food, dairy, beverage, brewing, pharmaceutical, and cosmetic industries to clean large fixed equipment, such as fermenters, bioreactors, centrifuges, heat exchangers, tanks, and piping. Vessels and other large equipment are cleaned by spraying the cleaning fluid on product contact surfaces, thereby creating a falling film of fluid on the surface. Piping and associated components, such as valves and pumps, are cleaned by flooding them with the cleaning fluid. Advantages of CIP systems include low labor requirement, fast equipment turnaround, and consistency. High initial investment and equipment maintenance costs and relatively high usage of water, chemicals, and energy are the main drawbacks.
Clean-out-of-place
Small vessels and parts that can be readily disassembled are typically cleaned out of place in a parts washer or a bath with a continuous circulation loop. Clean-out-of-place (COP) processes can be manual, semiautomated, or fully automated. COP systems are generally more customizable, require less initial investment and maintenance, and consume less water, chemicals, and energy than CIP systems. Labor requirements and equipment turnaround times, however, are generally higher for COP processes, especially for manual and semiautomated systems. Custom designed parts washers are generally more effective and efficient at removing tenacious deposits in localized “hot spots” that are deemed to be difficult to clean. This is because the nozzles in a parts washer can provide high fluid impingement and shear at specific locations. Examples of tenacious deposits are cell debris and denatured proteins that dry on heated surfaces in heat exchangers and autoclaves. Surfaces that are difficult to access, such as the insides of pumps, valves, and filling needles, can be cleaned effectively with sonication, typically at frequencies between 18 and 120 kHz. COP baths are also used to pre-soak and pre-clean parts to reduce cycle-time or facilitate the use of milder cleaning conditions for manual cleaning, such as a lower temperature and cleaning agent concentration, and the use of milder cleaning agents such as a weak alkali or acid.
Analytical Methods
Analytical methods that are widely used to quantitate chemical contaminants include conductivity, total organic carbon (TOC), chromatography, and ultraviolet and fluorescence spectroscopy. Additionally, sodium dodecyl sulfate polyacrylamide gel electrophoresis is used to assess fragmentation and aggregation of protein during cleaning.5
Conductivity is mainly used to verify clearance of ionic contaminants and cleaning chemicals such as alkali and acid cleaners. TOC is widely used to quantitate organic contaminants. It is also a useful method for detecting biological residues on reusable medical devices (see chapter 47).6,7,8 The limit of quantitation (LOQ) of TOC is approximately 0.1 ppm, which is equivalent to approximately 5 µg/cm2 for swab samples (R. Sharnez, unpublished data, 2019). In comparison, the visible residue limit of most process residues is between 1 and 4 µg/cm2.9 A drawback of TOC is that samples can become contaminated with organic solvents and disinfecting agents, such as alcohol, which in turn can result in apparent failures and complex investigations. Also, being a nonspecific method, TOC measures organic carbon in the entire residue, not just in the contaminant. Thus, all of the measured carbon in the residue is attributed to the contaminant, a worst-case assumption that can greatly overestimate the concentration of the contaminant. Another drawback of TOC is that the recovery is sometimes too low to accurately quantify the amount of contaminant in a sample.10 Also, to accurately determine the recovery of a contaminant, the theoretical (ie, actual, not measured) carbon fraction of the contaminant in the residue is required. This information is seldom available for complex soils, such as human, plant and animal-based materials, proprietary culture media, and biological waste. These drawbacks are especially significant when the contaminant is composed of a relatively small fraction of the overall carbon in the residue. Product-specific assays, such as enzyme-linked immunosorbent assay and other immunoassays, overcome some of the limitations of TOC but can require more time and expertise to develop. They also have greater specificity and sensitivity; for example, the LOQ of most immunoassays is approximately 1 ppb.
Acceptance limits, when applicable, for bioburden and endotoxin, and alert and action limits for swab and rinse samples are discussed later in this chapter.
Cleaning Mechanisms
The effectiveness of a cleaning procedure is determined by numerous factors including the post-use equipment hold time, the type of soil, humidity, materials of construction, surface characteristics (defects, corrosion, and roughness), hydrodynamics (fluid shear), solubility, temperature, pH, cleaning chemistry, active and enabling ingredients in cleaning agents (surfactants, wetting chelating agents, and additives) and their concentrations, mechanical action (in manual cleaning), and the duration of each step in the cleaning process (time).11 These factors are discussed in this section in the context of cleaning biological soils with aqueous cleaning solutions as an example.
Mechanisms Related to Soil and Surface Characteristics
The cleanability of a soil depends on several factors including composition, thickness of the deposit, surface defects and corrosion, affinity to the surface, and cohesive forces within the soil matrix; swelling, degradation, and denaturation of the soil during cleaning; and moisture content (dryness) and porosity (if the soil is sufficiently dry). Most soils become harder to clean as they lose moisture, but some soils can become easier to clean, and even slough off the surface, when they are sufficiently dry. Thus, the post-use hold time and drying rate can be critical in evaluating the cleanability of a soil. The drying rate is primarily determined by humidity, temperature, and circulation rate of the surrounding air. Because of their strong adhesive bond with most materials, proteins are likely to be the first soil constituent to attach to equipment surfaces, forming the framework for other constituents to attach. The adhesive bonds between proteins and some surfaces can be quite strong, especially if the deposit is formed on a hot surface as in a heat exchanger. Also, denaturation and aggregation of proteins increases with temperature and pH, which generally makes them harder to remove.
Mechanisms Related to the Cleaning Process
Most cleaning processes remove surface residues by mechanical means (fluid shear or scrubbing) and chemical diffusion (Figure 8.1). These mechanisms are facilitated by wetting, chemical reactions (hydrolysis), and solubilization. Cleaning processes for biological residues generally rely on both shear and diffusion to adequately remove them.
Soil Removal by Fluid Shear
Fluid shear stress (tF) is the tangential force per unit of surface area exerted by the flowing cleaning fluid on the surface. For a given flow rate and configuration, tF increases with fluid viscosity. Also, viscosity decreases sharply with temperature; for example, the viscosity of water at 20°C (1 centipoise) is over 2.8 times greater than it is at 80°C (0.35 centipoise). Thus, at a given flow rate, tF decreases substantially with increasing temperature. For this reason, soil removal by fluid shear is more effective at a lower temperature.
Under optimum cleaning conditions, the shearing action of the fluid can remove over 90% of the soil. Depending on the type of soil and surface, and the drying conditions (post-use equipment hold time, temperature, and humidity), the minimum shear stress required to effectively remove process residues (tF,MIN) is typically of the order of 1 N/m2 (10 dynes/cm2). For pipes and hoses, this corresponds to an average fluid velocity of approximately 5 ft/s and a Reynolds number (Re) of approximately 10,000. For dairy deposits, which are typically very difficult to remove, tF,MIN was reported to be between 0.75 and 2.5 N/m2.12 Fluid shear also aids in removing biofilms, especially those with structures that protrude beyond the laminar sublayer into the turbulent zone (see Figure 8.1).
Soil Removal by Diffusion
Diffusion is the movement of a chemical species A through a mixture of A and a medium B because of a concentration gradient of A. Ordinarily, this movement occurs from a region of higher concentration to a region of lower concentration. The rate of diffusion is determined by the magnitude of the concentration gradient of A in B, and the diffusivity of A in B (DAB). For liquids, DAB increases with temperature and decreases with viscosity and molecular weight (size) of A. Thus, for a given chemical contaminant (A) and cleaning fluid (B), the kinetics of cleaning by diffusion increases with temperature. Increasing the temperature generally also provides the additional advantages of higher solubility and faster rate of hydrolysis and other chemical reactions, such as those that break down proteins into peptides and other smaller fragments. Smaller fragments are generally more soluble and have higher diffusivities and are therefore more readily removed from the surface by diffusion.
Equipment and Design Considerations
Equipment should be designed and maintained to ensure adequate contact with cleaning fluids and proper drainage. Surfaces should be sloped at an angle of repose of at least 5 degrees to facilitate drainage. Dead ends should be avoided, but if they are necessary, the length-to-diameter
ratio should be minimized and preferably should be less than two. Equipment should be fabricated with materials and surface finishes that resist corrosion under processing and cleaning conditions. Surfaces with high free surface energy, such as stainless steel and glass, are more hydrophilic and generally have greater bacterial attachment and biofilm formation rates than hydrophobic surfaces such as Teflon, Viton, ethylene propylene diene monomer, and silicone rubber.
ratio should be minimized and preferably should be less than two. Equipment should be fabricated with materials and surface finishes that resist corrosion under processing and cleaning conditions. Surfaces with high free surface energy, such as stainless steel and glass, are more hydrophilic and generally have greater bacterial attachment and biofilm formation rates than hydrophobic surfaces such as Teflon, Viton, ethylene propylene diene monomer, and silicone rubber.
Flow of cleaning fluids should be turbulent to provide sufficient fluid shear at the surface. Typically, this requires a Re greater than 4000 for pipes and hoses and a flow rate greater than 2.5 gpm per foot for a falling film. Also, the velocity of cleaning fluids should be sufficient to ensure flooding of pipes and hoses. Typically, this requires the average fluid velocity to be greater than 5 ft/s.
Another important consideration is the residence time or turnover rate of water in the system and its effect on microbial growth. For a given equipment configuration, the lower the fluid velocity, the longer the residence time. With longer residence times, disinfectants can become less effective and water temperatures can rise. These conditions generally favor microbial growth and the formation of biofilms. Increases in coliform contamination have been reported in distribution systems with longer residence times.13 Additionally, at lower fluid velocities, particles are more likely to settle and thereby create new habitats for microbial growth. Residence time can be reduced by eliminating dead legs in pipes and periodic flushing of stagnant zones. Routine flushing and equipment maintenance can help identify mistakenly closed valves, dead legs, and other areas of poor circulation.
Cleaning Cycle
Based on the cleaning mechanisms and equipment design considerations discussed in previous sections, cleaning cycles in industrial processes often include the following steps.
Pre-rinse
The purpose of a pre-rinse is to wet the soil and physically remove it from the equipment. Wetting weakens the cohesive bonds between soil components, thereby facilitating their removal by fluid shear. It also opens up pathways for soil components to diffuse into the cleaning fluid, and for cleaning chemicals to diffuse into the soil matrix during subsequent chemical washes. As discussed earlier, because of the inverse relation between temperature and fluid shear, pre-rinses are generally more effective at a lower temperature. Pre-rinsing at a lower temperature also minimizes denaturation, aggregation, and subsequent redeposition of proteins and other biological macromolecules on equipment surfaces.
At sufficiently high fluid shear (typically of the order of 1 N/m2), the pre-rinse can remove over 90% of the soil. Under these conditions, the optimum duration of the pre-rinse is generally between 1 and 5 minutes. A more precise estimate of the optimum duration of the pre-rinse can be obtained by monitoring the concentration of solids in the effluent rinse water by measuring optical density, for example, and noting the time it takes for the effluent to become clear.
Alkaline Wash
Alkaline cleaning chemicals such as sodium hydroxide (NaOH) can be very effective at removing proteins and other biological resides from device and equipment surfaces. Alkaline cleaning agents hydrolyze proteins into peptides and other fragments that are more soluble and therefore less likely to aggregate and redeposit on equipment surfaces. They can also enhance wetting and solubilization. These mechanisms are generally favored at higher NaOH concentration, that is, higher pH; however, most proteins denature and aggregate rapidly at NaOH concentrations above 1% and temperatures greater than 70°C (R. Sharnez, unpublished data, 2019). Because denatured protein aggregates are generally more difficult to clean, the optimum concentration and temperature of NaOH for cleaning proteinaceous soils is typically between 0.5% and 1% (pH approximately 12.5) and between 50°C and 70°C. Under optimum conditions, an alkaline wash can reduce most proteinaceous residues to visually undetectable levels within 5 to 10 minutes.
In some instances, the duration of the alkaline wash can be shortened or otherwise optimized by adding surfactants and wetting agents (as in formulated cleaning agents; see chapter 6). Nonionic wetting agents are good emulsifiers and can reduce foaming. Sequestrants, such as sodium phosphate derivatives, may be required to chelate minerals in hard water and prevent them from precipitating during the cleaning process. Chlorine compounds and peroxides, such as sodium hypochlorite (bleach) and hydrogen peroxide, can also enhance the effectiveness of alkaline cleaning agents; however, they can corrode stainless steel and other metals, especially at concentrations and temperatures above 1% and 50°C (R. Sharnez, unpublished data, 2019).
Intermediate Rinse
The alkaline wash is typically followed by an intermediate rinse to remove the cleaning agent and entrained particles.
Acid Wash
An acid wash is sometimes necessary to remove inorganic constituents and precipitated minerals. It is also used to passivate stainless steel and other metal surfaces.
Final Rinse
The purpose of the final rinse is to reduce residual cleaning chemicals and entrained particles to acceptable levels. The final rinse is performed with high purity water, such as water for injection (WFI), or distilled or deionized water.
The effectiveness of the earlier steps is determined by several equipment parameters such as contact between cleaning fluids and soiled surfaces (spray coverage), drainability of equipment surfaces, and effective control of critical process parameters (CPPs) such as flow rate and temperature and concentration of the cleaning fluids.14,15,16 Spray coverage is evaluated at full scale with a dilute aqueous suspension of riboflavin. Bench-scale cleanability studies are used to optimize CPPs12,17,18,19 and identify suitable master soils for developing and validating cleaning procedures at full scale.20,21
Various other cleaning formulations and processes can be used depending on the type of soil and the acceptable level of the contaminant. These can be as simple as using high purity water alone, particularly for residues that are highly soluble in water, but can also include the following:
Organic solvents such as methanol for residues that are not water soluble
Acid cleaners as described earlier for inorganic residues
Formulated cleaners with additives that facilitate cleaning, such as surfactants, chelators, and wetting agents
Enzyme-based cleaners that target specific types of biological macromolecules, such as proteases for proteins and lipases for lipids (see chapter 47)

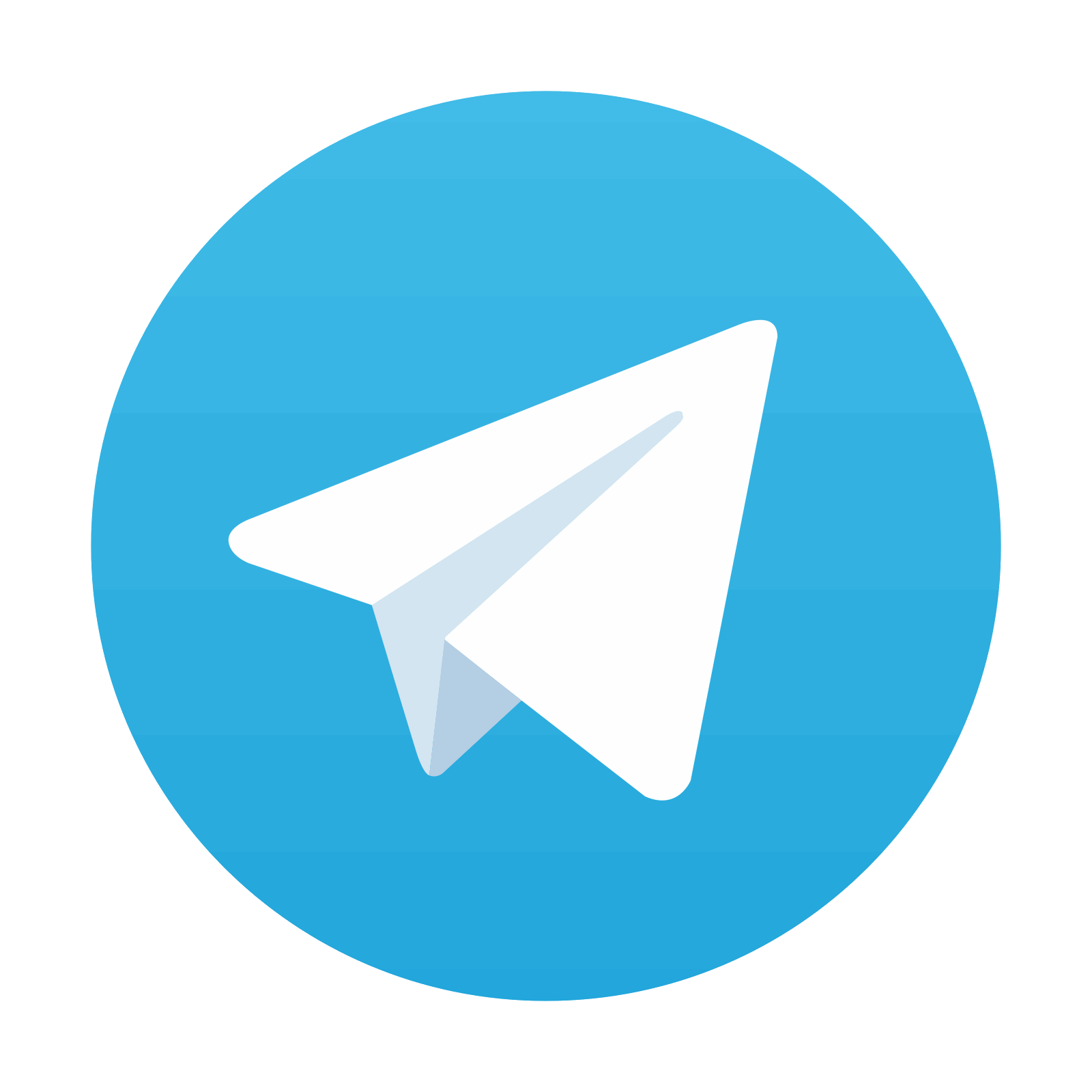
Stay updated, free articles. Join our Telegram channel

Full access? Get Clinical Tree
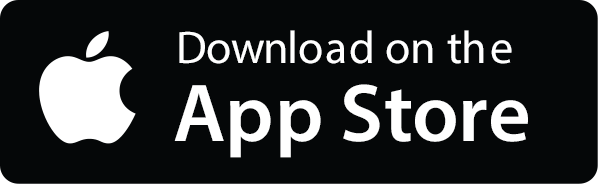
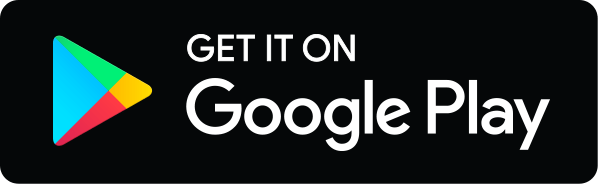