11 Eric S. Sobel and Stephen M. Roberts Exposure to a variety of chemicals and biological agents has been implicated in the onset of symptoms of immune origin, including acute and chronic respiratory distress, dermal reactions, and manifestations of autoimmune disease. The types of substances associated with immune system effects is extraordinarily diverse and include chemicals found in occupational and environmental settings, infectious materials, certain foods and dietary supplements, and therapeutic agents. As discussed in this chapter, dysregulation of the immune system by toxicants can lead directly to adverse health effects, as well as rendering the body more susceptible to infectious disease and cancer. The immune system is highly complex, with many facets poorly understood. Because of this, assessment of potential immunotoxic effects of drugs, chemicals, and other agents is not a simple task. Often, measurement of a variety of components of the immune system and/or their functionality is required to gain an appreciation of the likelihood of immune dysfunction from drug or chemical exposure. Increasingly, there is realization that the immune system may be among the most sensitive target organs for toxicity for many chemicals and, as a result, merits special attention. This chapter discusses the following: The immune system has evolved primarily to defend the body against the invasion of microorganisms, although normal immune function is important in regulating and sustaining the internal environment as well, such as recognition and removal of malignant cells. There are two types of immunity: natural immunity (also termed innate immunity) and acquired immunity (also termed specific immunity). Innate immunity encompasses a wide variety of protective mechanisms, including physical (e.g., barrier activity of skin), chemical (e.g., acidic environment in the stomach), and mechanical (e.g., coordinated movement of cilia in the respiratory tract removing particulate matter). It is also mediated by a number of agents, both secreted (e.g., complement, defensins, type I interferons) and cellular (e.g., neutrophils, macrophages, and natural killer (NK) cells). While originally thought that cells of the innate immune system were activated with little specificity, recent research has identified a number of pattern recognition receptors for ligands that represent potentially dangerous events. These include the recognition of common motifs from exogenous threats, collectively called PAMPs (pathogen-associated molecular patterns) and endogenous triggers, known as DAMPs (damage-associated molecular patterns). A family of 11 TLR (“toll-like” receptors), for example, recognizes specific motifs either not usually seen in mammalian cells or found in unexpected places. These motifs include bacterial lipoproteins, single-stranded and double-stranded RNA, flagellin, and bacterial DNA. Natural immunity differs from adaptive immunity in that the receptors are DNA-encoded at the germ line and is rarely enhanced by prior exposure to these substances. Acquired immunity, in contrast, increases in magnitude and specificity with successive exposure to foreign substances. Substances that trigger these specific immune responses are termed immunogens, and may be either foreign or endogenous. In many cases, immunogens are proteins or contain a protein as part of the complex. There are two major types of acquired immune responses: humoral immunity and cell-mediated immunity. Humoral immunity involves the production of proteins capable of binding to foreign substances. These belong to a special class of proteins called immunoglobulins (Igs), and the secreted proteins themselves are called antibodies. The substances to which the antibodies bind are called antigens. Antibody binding can neutralize toxins, cause agglutination of bacteria and other microorganisms, and lead to precipitation of soluble foreign proteins. By coating the pathogen with antibody that can activate complement, it can also direct cells of the innate immune system to eliminate the pathogen through enhanced phagocytosis—a process known as opsonization. Each of these is important in defense of the host. In cell-mediated immunity, specialized cells rather than antibodies are responsible for the destruction of foreign cells. A critical function of the immune system is to effectively distinguish between macromolecules that belong, or do not belong, in the body. The specific immune response is believed to be highly individualistic, a process which defines “self” while also defending the organism against “nonself.” This is evident by the response to certain environmental toxicants, to allergens or antigens, and the specific rejection of allografts. Recognition of “self” is known to be guided, in part, by genetic variations in proteins of the class I and II major histocompatibility complex (MHC). Initially, the ability of the immune system to differentiate “self” from “nonself” is the result of a rigorous selection process. During maturation, the system must ignore an infinite variety of self-molecules and yet be primed and ready to respond to an array of exogenous antigens. Immunomodulatory control mechanisms lead to immune tolerance of self and carefully orchestrate the immune response to targets and removal of foreign macromolecules and cells. These control mechanisms arise from interactions among the several different cell types with roles in proper immune function. Lymphocytes are considered to be the major cells involved in a specific immune response in humans. They are derived from pluripotent stem cells and undergo an orderly differentiation and maturation process to become T cells or B cells, with critical functional roles in the host defense. T-cell development occurs primarily in the thymus, where certain cell surface protein markers are acquired during the selection and differentiation process. Collectively, protein markers expressed on cells of the immune system are called CD antigens (for cluster of differentiation), and well over 300 different CD antigens have been identified in humans. The presence of combinations of CD antigens, detectable by immunofluorescence, has been used to positively identify immunocytes. In general, mature T cells exiting the thymus and present in the circulation and secondary lymphoid tissue (lymph nodes and spleen) are characterized by the presence of CD3+ and either CD4+ or CD8+ surface markers and are devoid of surface or cytoplasmic Ig. CD3 is a complex that associates with a T cell receptor (TCR), and it is the TCR that has been the target of the selection process, mainly to avoid those receptors that either cannot bind to self MHC (and are therefore useless) or bind too avidly to self MHC (and are therefore likely self-reactive). There are various subtypes of T cells, such as T-helper (TH) cells, regulatory T (Treg) cells, and cytotoxic T cells (TC). TH and most Treg lymphocytes carry the CD4+ marker, while TC lymphocytes have the CD8+ marker. Together, these T-lymphocyte populations play a vital role in initiating and regulating the immune response. Human B cells develop from stem cells in the fetal liver and, after birth, B-cell development occurs principally in the bone marrow. B-cell development and maturation are characterized by class-specific Ig expression on the cell surface. B cells also undergo a selection process to avoid self-reactivity, although it is not as rigorous as for T cells. Monoclonal reagents can identify the Ig expressed on the surface of B cells. Immunophenotypic characterization of cells via these and other CD markers has proved to be invaluable in certain clinical situations (such as identifying the type of lymphoma) and a useful research tool. B cells play an important role in recognition of antigens and are responsible for antibody production. Another important cell in the specific immune response is the “professional” antigen presenting cell (APC). These cells make first contact with the antigen and may also process the antigen; that is, modify it in such a way as to enable its recognition by T cells. This category of cells is defined more by function than cell type. There are three types of professional APCs: peripheral blood monocytes (which can differentiate into tissue macrophages), B cells, and dendritic cells. Professional APCs are distinguished by their ability to express both MHC class I and II; virtually all other nucleated cells express only class I. Peptides processed and bound to class II are generally of extracellular origin, while peptides bound to class I are derived from the cytoplasm of that cell, providing a pathway for internal surveillance against important pathogens, such as viruses. Dendritic cells are responsible for initiating most primary (naïve) T cell responses, and are unique in their ability to process exogenous antigen by both the class I and II pathways (cross-presentation). In order for the APC to present the antigen to T cells, the antigen must have a polypeptide component to it which is processed, or partially digested by the APC, and sequences from that peptide are “presented” on its cell surface bound to an MHC class I or class II molecule. Presentation of antigen to B cells does not require this processing, and in fact B cells are capable of recognizing antigens directly. These antigens interact with Igs serving as receptors on the cell surface of B-cell clones. Every Ig molecule expressed by an individual B cell has identical specificity, and these Igs can be quite specific in terms of the antigens with which they will interact. Thus, a particular antigen may interact with only one or a few B cell clones, a critical aspect in creating a specific immune response. When the antigen binds to an Ig receptor on the B cell surface, the antigen–receptor complex migrates to one pole of the cell and is internalized within the cell. While the Ig receptor can bind to virtually any structure, for the B cell to become activated and productively engage specific T cells, the antigenic complex must also have a polypeptide component that can be processed and antigenic peptides displayed via the MHC class II pathway. Naïve T-cell activation requires at least two signals. The first signal results from an interaction between the T-cell receptor and the MHC/peptide complex and provides the specificity. The second signal is the product of interactions between co-stimulatory receptors constitutively expressed by T cells and their ligands, usually inducibly expressed by the APCs. Expression of the co-stimulatory ligands is induced by the danger signals of the innate immune system. To facilitate the interactions between the rare T cell specific for a peptide derived from the pathogen and the APC presenting those peptides, dendritic cells activated by danger signals at the site of contact take up antigen and migrate (along with free antigen) via the lymphatic system to the nearest draining lymph node. There, they encounter naïve T cells that are programmed to continuously circulate through lymph nodes. The architecture of the lymph node promotes efficient cell-to-cell contact and intercellular signaling. In general, an effective immune response requires the differentiation and proliferation of specific subsets of TH cells (TH1, TH2, and TH17 cells) which respond to and, in turn, secrete different cytokines in a complex interplay of positive and negative feedback loops. Cytokines are low-molecular-weight proteins that mediate communication between cell populations, and the net product of these influences at the initiation of the primary response leads to activation of a master transcriptional factor. A partial list and functional classification of cytokines is shown in Table 11.1. TH1 cells (master transcriptional factor Tbet) are involved in the activation of macrophages by INF-γ, secrete tumor necrosis factor (TNF), and mediate delayed-type hypersensitivity responses. The most critical function of TH2 cells (master transcriptional factor GATA-3) is to stimulate B cells to produce antibody, but they also secrete cytokines (specifically, interleukins, designated IL) that may regulate mast cells (IL3, IL4, and IL10), eosinophils (IL5), and IgE (IL4) responses in allergic diseases. Of the several factors known to participate in immunomodulation, IL4 and IL10 are particularly noted to upregulate the humoral response while suppressing the cell-mediated response (see in the following text for more discussion of humoral vs. cell-mediated immunity). IL13, which is produced by activation of T cells (Table 11.1) and shares many of the properties of IL4, also suppresses cell-mediated immune responses and the production of proinflammatory cytokines (IL1, IL6, IL8, IL10, IL12, and TNF). More recently, TH17 cells (named for the effector cytokine IL-17 and differentiated by the master transcriptional factor RORγ) have been shown to be important mediators for many chronic inflammatory responses. An effective cytotoxic T-cell response to a novel pathogen typically also requires prior or coincident activation of the APC (typically a DC) by TH cells. Table 11.1 Major Cytokines and their Functions Similar to naïve T cells, naïve B cells circulate through lymph nodes via the blood. Within the lymph node, the rare B cell with Ig receptor specificity for an epitope (antigenic domain) present in the antigen can bind antigen with high affinity and process the protein component via the MHC class II pathway. When an activated TH cell binds to the antigenic peptide–MHC complex on that B cell, the B cell is stimulated to replicate and differentiate into an antibody secreting plasma cell. This B-cell clonal expansion occurs in the germinal center of the lymph node in a highly orchestrated fashion that leads to survival and proliferation of the B-cell clones with the highest affinity for the epitope that originally selected that B cell. This process of affinity maturation occurs through somatic hypermutation (programmed mutations in the DNA of the regions of the Ig genes coding for the binding site) and selection. Through this mechanism, the immune system is able to produce the necessary quantities of antibodies targeting specific molecules (antigens) regarded as foreign. The synthesis of the antibody is tightly regulated; however, the proliferation of plasma cells and antibody synthesis are controlled by cytokines and interactions with T cells. Regulatory T cells (Treg; master transcriptional factor FoxP3) function to suppress the immune response. Control of the immune response is achieved by balancing the stimulatory and inhibitory effects of T cells and various cytokines. After an encounter with an antigen, antigen-specific T cells undergo rapid proliferation and differentiation in secondary lymphoid tissue into “armed effector” and “memory” cells. The specific signals that determine whether an individual T cell becomes an effector or memory cell is not yet well-understood. However, with clearance of the antigen, there is an active down-regulatory mechanism that rids the immune system of now unneeded armed effector T cells but retains an expanded population of antigen-specific memory T cells. It is these memory T cells that are responsible for the stronger and more rapid response to subsequent encounters with the antigen that characterizes adaptive immunity. Moreover, subsequent responses no longer absolutely require a co-stimulatory signal. An analogous process occurs for B cells, with the outcome being either differentiation into antibody-secreting plasma cells or memory B cells. Like memory T cells, memory B cells are responsible for the brisker and higher-titer antibody response seen on subsequent antigen encounter. Also similar to armed effector T cells, the majority of the plasma cells survive only for a few weeks. However, some migrate to the bone marrow and successfully compete for limited niches. There, they receive survival signals and become long-lived plasma cells. Because immune responses to viruses or immunization encountered in childhood generally result in lifelong immunity, it has been assumed that “memory cells” last indefinitely following a single antigen contact. However, evidence suggests that the life span of memory cells may be related to repeat contact with antigen. In order to be recognized by the immune system, antigens must be of appreciable size. Some of the smallest antigens, for example, are natural substances with molecular weights in the low thousands. There are circumstances where much smaller molecules can elicit an immune response, but this requires the participation of a large molecule to serve as a carrier. For example, some metals, drugs, and organic environmental and occupational chemicals too small to be recognized by the immune system can become antigenic when bound to a macromolecule, particularly proteins. Once the immune response has been initiated, antibodies will recognize and bind the small molecule even when it is not bound to the carrier molecule. In situations such as this, the small molecule is called a hapten. The antibodies themselves are glycoproteins, the basic unit of which consists of two pairs of peptide chains (see Figure 11.1) connected by disulfide bonds. The longer peptide chain is termed the heavy (or H) chain and the shorter is the light (or L) chain. There are five main types of antibodies, or immunoglobulins (Igs): IgG, IgM, IgA, IgE, and IgD. They differ both in structure and function. IgG is present in the greatest concentration in serum, has a molecular weight of around 150,000 (there are four subtypes of somewhat different sizes), and is important in secondary immune responses. They distribute into the extracellular space throughout most of the body. IgM is a primary response antibody, meaning that it is increased very early in an immune response, especially on the naïve encounter. IgM is much larger than the other Igs, consisting of five sets of heavy/light-chain pairs bound together at a single point with another peptide (the J chain). Its molecular weight is about 970,000. Its size largely confines it to the vascular space, and its multivalency allows it to bind to target even when affinity is low. It is these properties that make it useful as a first responder of the adaptive immune system in eliminating blood-borne pathogens. IgA may exist as a monomer (one basic unit of two pairs of H and L chains) or as a dimer (two basic units bound together with a J chain). The monomeric IgA is the primary Ig found in blood, although present at low concentrations. The dimeric form, with a molecular weight of 385,000, is actively transported to mucosal surfaces, where it serves to prevent binding of certain pathogens to mucosal surfaces. Its dimeric structure resists degradation in that challenging environment. IgD has a molecular weight of about 184,000, and is present in very low concentrations in serum. Its function is unclear, but it may play a role in B-cell differentiation. IgE is slightly larger than IgG (molecular weight of 188,000) and is normally present in vanishingly low concentrations in serum. It binds through high-affinity receptors on mast cells strategically located skin and mucosal barriers and arms them to respond to parasites. It is the primary antibody involved in immediate hypersensitivity reactions.
IMMUNOTOXICITY: TOXIC EFFECTS ON THE IMMUNE SYSTEM
11.1 BIOLOGY OF THE IMMUNE RESPONSE
Cytokine
Produced By
Function(s)
IL1 (IL1-α and IL1-β)
Macrophages, epithelial cells
Variety of effects, including neutrophil and macrophage activation, T- and B-cell chemotaxis, and increased IL2 and IL6 production
IL2
T cells
Stimulates replication of T cells, NK cells, and B cells
IL3
T cells, thymic epithelial cells
Involved in regulation of progenitor cells for several different cell types, including granulocytes, macrophages, T cells, and B cells
IL4
Activated T cells, mast cells
Differentiates TH2 cells; activates B cells and induces class switching to IgE
IL5
T cells and activated B cells
Increases secretion of immune globulins by B cells; eosinophil growth
IL6
T and B cells, macrophages
Important in inflammatory reactions and in differentiation of B cells into Ig-secreting cells; fever
IL7
Bone-marrow stromal cells
Growth of pre-B cells and pre-T cells
IL8
Activated monocytes and macrophages
Activates neutrophils; important for chemotaxis of neutrophils and lymphocytes
IL10
Monocytes
Inhibits inflammatory and immune responses
IL12
Macrophages, dendritic cells
Activates NK cells; induces differentiation to TH1 cells
IL17
Activated T cells
Stimulates fibroblasts, epithelial cells, and keratinocytes to secrete cytokines that recruit inflammatory cells
IL23
Dendritic cells
In combination with other cytokines, stimulates differentiation of naïve T cells to become TH17 cells
TNF-α
Variety of cells, primarily activated macrophages
Important in inflammatory responses; effects similar to IL1
TGF-β
Variety of cells
Inhibits T-cell proliferation and suppresses inflammatory responses
TNF-β
Activated CD4 + cells(TH)
Important in mediating cytotoxic immune responses, cell lysis
Interferon-α
Leukocytes and dendritic cells (IFN-α), fibroblasts (IFN-β)
Increased MHC class I expression; inhibits viral protein synthesis
Interferon-β
Interferon-γ
T cells, natural killer cells
Macrophage activation; suppresses TH2
Stay updated, free articles. Join our Telegram channel

Full access? Get Clinical Tree
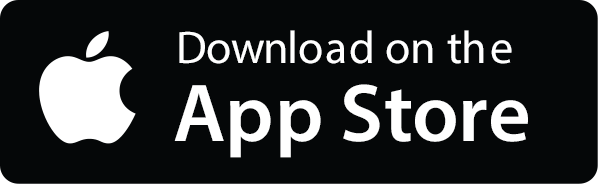
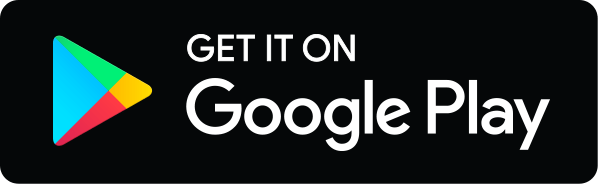