Fig. 7.1
Schematic diagram representing folliculogenesis in mammals. Primordial germ cells are characterized by having flattened granulosa along the basal lamina and surround the oocyte. Granulosa cells become larger and more cuboidal in primary follicles. The recruitment of thecal cells begins at the end of the primary follicle stage and a single layer surrounds the secondary or pre-antral follicle. At this stage the granulosa remain cuboidal. Secretions from the oocyte lead to the formation of a cavity, or antrum, which distinguishes the tertiary, or antral, follicle. This is also referred to as the Graafian follicle. At this stage, the thecal layer is vascularized and multiple layers of thecal cell cells are present. Following ovulation the remaining follicle degenerates into the corpus luteum and eventually into the corpus albicans
In addition to gonadotropins and steroid hormones , studies have shown that other hormones play an important role in the regulation of ovarian development. Thyroid hormones have received considerable attention over the years, although a clear understanding of thyroid hormones regulation remains somewhat elusive. In lower vertebrates such as fish, however, the role of the thyroid hormones on ovarian function is more clearly defined [39]. There have been numerous studies in which temporal profiles of thyroidal status and reproductive function have been compared for many fish species of both genders. In many fish species, thyroid hormones play an important role in early oocyte development [39, 40]. In rainbow trout, triiodothyronine (T3) is particularly effective at low gonadotropin concentrations and appears to play a key role at the onset of gonadal development and subsequent reproduction [41, 42]. One component of thyroid hormone action involves cellular mechanisms blocking phosphodiesterase activity, thereby decreasing the rate of degradation of gonadotropin-stimulated intracellular cAMP [43]. Thyroid hormone levels also regulate the transactivation of the Cx43 promoter in vitro in the testis [44]. This effect is independent of cAMP, indicating that thyroid hormones can directly regulate gene expression in gonadal tissue [44].
In women, hypothyroidism has been associated with impaired ovulation, fertilization, implantation, miscarriage, and late pregnancy complications [45, 46]. In both hypo- and hyperthyroid women, there have been reports of alterations in the menstrual cycle [47]. These have generally been associated with effects on the LH/FSH cycles of these women [48]. A difficulty in establishing clinical interaction between altered thyroid function and infertility in women is the fact that most patients will likely be treated for their thyroid disorder before seeing a fertility specialist [45].
Direct actions of thyroid hormones on the ovary have also been documented. Studies have reported that the ovary expresses 5′-monodeiodinases (5′D) and can convert thyroxin (T4) into metabolically active T3 [49]. Both thyroid hormones are present in the fluid of antral follicles, and while levels of T4 are lower than circulating blood levels, levels of T3 are similar. 5′D activity is present in the ovary early during follicular development and peaks at the time of ovulation. Early studies have indicated that hypothyroidism in humans or in PTU-induced hypothyroid mice has been associated with decreased sensitivity to FSH and increased formation of polycystic ovary syndrome [50, 51]. Ovarian cyst formation has also been stimulated in hypothyroid animals administered high levels of gonadotropins [52, 53]. Whether or not this is due to an inability of the oocyte to mature or to other effects is not clear. Maruo et al. [54] have suggested that the role of thyroid hormones is associated with amplification of the FSH signaling on the ovarian thecal cells. These data support the notion that the role of thyroid hormone on ovarian maturation has been highly conserved in evolution. Given that many contaminants, such PCBs, PBDEs, and Bisphenol A, among others, have been shown to exert anti-thyroid effects [55], using thyroid hormone markers of ovarian function may be important when thyroid function is thought to represent a specific target for a given contaminant.
Cellular Communication in the Ovary
Cellular communication between cells occurs via endocrine, paracrine, or direct interactions. Direct cell–cell interaction results in the activation of signaling pathways in which information from the cell’s external contacts is relayed into the cell to regulate gene expression in response to changing external contacts between cells. These interactions are mediated by cell adhesion molecules and tight junction proteins. Other signals are conveyed directly between the cytoplasms of adjacent cells via intercellular channels, referred to as gap junctions, that connect the cytoplasms of adjacent cells [56–58].
Gap junctions are comprised of a family of proteins that includes connexins, innexins, and pannexins [59]. Connexins and innexins are implicated in the formation of gap junctions between adjacent cells that are present in vertebrate and invertebrate species, respectively. Pannexins are also present in vertebrates and share homology with the innexins. However, unlike the innexins, pannexins do not form intercellular channels but rather cellular pores that are implicated in paracellular communication via the release of ATP [59]. Connexins are transmembrane proteins that oligomerize to form hemichannels or connexons [60, 61]. There are 21 different connexins named according to either their molecular mass or sequence homology. The connexons that form the gap junction can be composed of either the same connexin or of different connexins. The composition of the connexon will determine the charge of the pore and the types of molecules which are able to pass through the pore.
In all mammalian species studied to date, four to six different connexins are expressed in the ovary [56, 57]. In all species, Cx43 appears to be the most prevalent connexin and is expressed in granulosa cells (Fig. 7.2), while Cx37 is expressed by the oocyte [56]. Certain studies have suggested that Cx43 is also present between the oocyte and the cumulus/granulosa cell layer [62], although others have failed to observe Cx43 between these cell layers [63, 64]. Veitch [64] claimed that gap junctions between oocytes and granulosa cell layer in fact comprised Cx37. In support of this, Cx37 knockout mice exhibit arrested folliculogenesis at the early antral stage [63] and oocytes fail to become competent [63, 65]. The role of gap junctional communication between the oocyte and the granulosa cells may involve regulation of cAMP transfer. Inhibition of gap junctional communication using heptanol or carbenoxolone results in decreased levels of cAMP in the oocyte [66, 67].
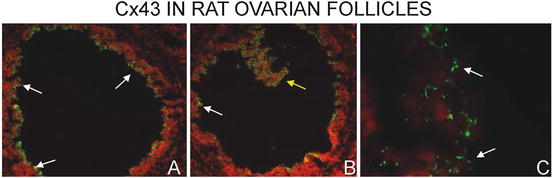
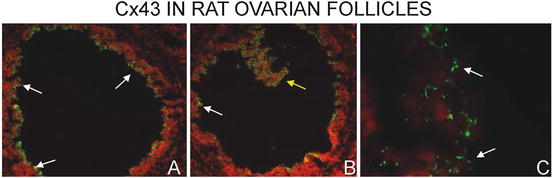
Fig. 7.2
Immunofluorescent localization of Cx43 in the oocyte of a rat ovary. Low power photomicrographs show extensive Cx43 immunostaining in the granulosa cells (white arrows). In the grazing section of panel B, one can observe the extensive reaction around the periphery of the cells suggestive of extensive gap junction formation (yellow arrow). At higher magnification (panel C), distinct punctate Cx43 immunostaining between adjacent follicular cells can be observed (white arrows). Nuclei were stained with propidium iodide and appear red while Cx43 is green
The regulation of Cx43 in rodent granulosa cells is mediated by FSH/LH [68, 69]. FSH appears to play an important role early in follicular development and positively regulates the expression of Cx43 [68, 70]). However, prior to ovulation, when LH levels increase, there is a decrease in Cx43 [71]. This decrease appears related to the LH surge which inhibits Cx43 via the MAPK and PKA signaling pathways [72].
Cx43 knockout mice die shortly after birth [73]. These animals, however, have fewer oocytes at birth [74]. If the oocytes from Cx43 knockout mice are placed under the kidney capsule of a wild-type mouse, or cultured in vitro, folliculogenesis can proceed to the primary follicle stage, but subsequent development is impaired [74]. Similarly, in mice harboring an autosomal dominant point mutation within the Gja1 gene (Gja1 Jrt/+ mice) which codes for Cx43, reduced granulosa cell coupling (0–20 % versus wild type) and impaired fertility are observed [75, 76]. This subfertility was due, at least in part, to impaired follicular development, with fewer pre-ovulatory follicles present in ovaries and reduced gonadotropin response. In addition to regulating folliculogenesis, Lin et al. (2003) have shown an inverse correlation between follicular apoptosis and Cx43 levels . Furthermore, the glucocorticoid dexamethasone, known to decrease apoptosis in ovarian follicles, increases levels of Cx43 [77, 78]. Interestingly, as Cx43 levels decrease in apoptosis, there is an increase in Cx32 levels. It has been suggested that Cx32 gap junctions propagate the death signal in the ovary [79, 80]. Thus, toxicants that stimulate a switch from Cx43 to Cx32 in the ovarian follicles may be useful for predicting ovarian apoptosis, thereby highlighting the importance of examining their expression and localization in toxicological studies. Whether or not toxicants like tributyltin (TBT), which induce apoptosis in the ovary during development, mediate changes in Cxs remains to be shown, even though TBT has been shown to decrease Cx43 in developing testes [81].
Toxicology data have also suggested a role of Cx43 in steroidogenesis. Exposure of rats to the environmental pesticide lindane blocks gap junctional communication [82]. In these animals there is also a concurrent decrease in the levels of P450ssc, the cholesterol transporter StAR, and a decrease in progesterone secretion, supporting the notion that Cx43 can influence steroidogenesis.
Ovary Cultures
In vitro culture of neonatal (postnatal day 0–4) rodent ovaries has been used to examine mechanisms underlying both ovarian follicular formation and development and toxicity of xenobiotics causing ovarian follicle loss [83–86]. Methodology involves isolating intact ovaries from young mice, rats, or hamsters and floating them on small pieces of nitrocellulose or other membrane which is in turn floating on the surface of culture medium [87, 88]. The majority of primordial follicles present remain dormant, but a slow steady number of follicles are able to develop normally to the secondary stage (multiple layers of granulosa cells) without added growth factors or hormones. Addition of growth factors or inhibitors can improve or inhibit follicle development or activation [86, 89]. Studies on direct toxic effects of drugs or pollutants have utilized this methodology as well. Follicles can be enumerated and staged by histology, gene expression can be monitored by either microarray or reverse transcription-polymerase chain reaction, and proteins can be localized by immunohistochemistry/immunofluorescence or quantified by Western blotting in spite of the small size of the tissue.
The role of DNA damage in the loss of primordial and primary follicles was examined, using cyclophosphamide as an example [28, 90]. Cyclophosphamide and other alkylating drugs induce DNA adducts, DNA double-strand cross-links, leading to DNA double-strand breaks [91]. In vivo studies determined that a reactive metabolite, phosphoramide mustard (PM), must be produced for follicle loss to occur [92]. Exposing cultured mouse ovaries to various concentrations of different metabolites of cyclophosphamide confirmed that only metabolites that could produce PM induced concentration-dependent loss of primordial and primary follicles [28]. TUNEL and cleaved Caspase 3 staining were used as markers of follicle injury or damage. Increased numbers of follicles with TUNEL staining were observed for 10 μM at 24 h and 3 μM at 48 h after exposure, suggesting that these markers are either not as sensitive as the follicle counts done 8 days after exposure or are time dependent, a more likely scenario. It was then determined whether or not DNA damage is an underlying factor in PM-induced ovarian follicle loss. Immunohistochemistry for phospho-histone H2AFX, a histone which becomes phosphorylated at sites of double-strand breaks [93], was performed to examine whether or not double-strand breaks can be observed in cultured rodent ovaries in response to PM [90]. Phospho-H2AFX staining was detected in a punctate pattern (foci) predominantly in oocytes of both cultured mouse and rat ovaries exposed to PM. Number of foci per oocyte and numbers of follicles with foci were both concentration dependent, peaking at 24–48 h with 3 and 10 μM. In another study, DNA cross-links in granulosa cells were observed and peaked 2 h following a single dose of CPA in immature Sprague–Dawley rats [94]. These results supported DNA damage as a mechanism of ovarian damage. Unfortunately, other immunohistochemical markers of DNA damage and repair remain to be developed for animal models, and more research is needed to further investigate oocyte-specific DNA damage signaling and repair capabilities in oocytes, as a unique target for DNA-damaging agents. In vitro work investigating VCD has examined the role of the KIT/KITL, PI3K, and FOXO3A signaling pathway in VCD-induced follicle loss. Initial evidence that follicle activation is affected by VCD was seen when a single dose of VCD induced an increase of primary follicles in rats [95]. Subsequent to that, global gene expression analyses of cultured rat ovaries and with isolated ovarian follicles identified cKit as a gene altered in both systems [96]. Addition of KITL, but not GDF9 or BMP4, to cultures partially protected against VCD-induced follicle loss. The PI3 kinase inhibitor, LY294002, inhibited follicle activation to primary follicles in cultured rat ovaries, and primordial follicles were not affected by VCD in the presence of LY294022, although numbers of small primary follicles were still reduced by VCD [97]. In cultures of postnatal day 4 rat ovaries, exposure to 30 μM VCD led to decreased immunofluorescence for KIT and FOXO3 protein in oocytes [98]. Decreased staining for phospho-AKT in oocyte nuclei was the earliest observed alteration on day 2 following VCD exposure.
Molecular Markers of Follicle Number and Health
Ovarian follicle counting is a necessary process for evaluating ovarian toxicants. Due to the relatively arduous process of determining follicle numbers during reproductive toxicology studies, automated or semi-automated methods have been sought. Typically for rodent studies, a thorough investigation of follicle numbers involves manually counting and determining the stage of all follicles in every 20th or 40th section of ovaries, following complete sectioning of one ovary per animal that has undergone standard processing and hematoxylin and eosin staining. Oocyte-specific markers were tested to either improve visibility of follicles for manual counting or for computer-assisted enumeration. PCNA has been tested, because oocyte nuclei stain darkly; however, proliferating granulosa cells are also stained and can be used to monitor granulosa cell proliferation and follicle health [99]. In spite of this lack of specificity, Muskhelishvili et al. [100] showed reduced variability in interindividual follicle counts with PCNA immunohistochemical staining. Furthermore, Picut et al. [101] demonstrated improved consistency and higher follicle numbers when PCNA-stained sections were counted manually, with a high correlation between manual counts and semi-automated computer-assisted counting. Cytochrome P450 1B1 immunostaining has also been reported to specifically stain oocyte nuclei and improve visibility of ovarian follicles for counting [102]. We have examined immunofluorescence of zona pellucida proteins (P Devine, Fig. 7.3, unpublished observations), using a specific antibody for this available from ATCC, originally developed by Jurrian Dean [103]. ZP3 are only present at the outer surface of oocytes in follicles of adults, including primordial oocytes although faintly (not shown). In adult mice, ZP3 is also observed in follicle remnants, with the zona pellucida forming sinuous structures within the interstitium (P Devine, Fig. 7.3, unpublished observations). This would represent a potential fluorescent marker that could be used to enumerate and even stage follicles based upon oocyte size if the empty zona pellucidae could be removed from analysis. It could be envisaged that transgenic rodents expressing a fluorescent protein under the regulation of oocyte-specific genes could be developed for specific use in reproductive toxicology studies; however, the cost of such a model and the effort required to produce it have precluded such efforts.
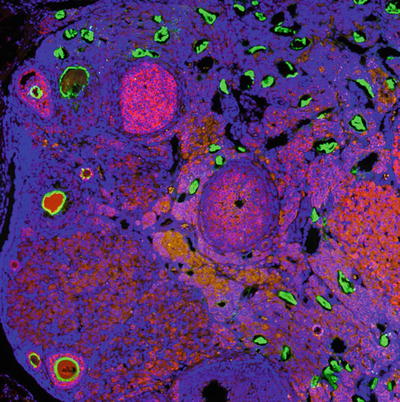
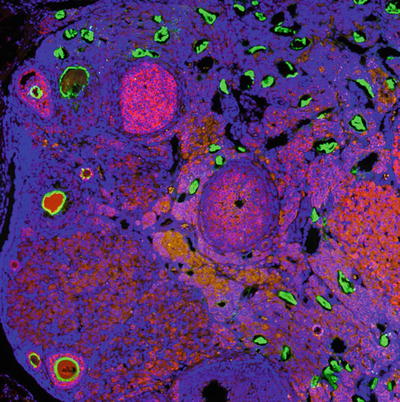
Fig. 7.3
Rat ovary stained for nuclei by Hoechst (blue) ZP3 (green) and anti-Mullerian hormone (pink/red). Corpora lutea had darker red background fluorescence, as well. ZP3 staining can be observed in structures that are likely follicle remnants, and can be differentiated from follicles by the shape of the structures and lack of oocytes within the zona pellucida
In order to identify healthy versus atretic follicles, TUNEL staining or immunohistochemistry for cleaved Caspase 3 has been typically performed to identify apoptotic granulosa cells in atretic follicles [28, 104]. Conversely, immunohistochemistry for PCNA or BrDU incorporation of proliferating granulosa cells has been examined for healthy follicles with proliferating granulosa cells (e.g., [105]). Immunohistochemistry staining for Anti-Mullerian hormone has also been used to identify non-atretic antral follicles when cisplatin was compared with vehicle only [104]. These markers tend to be qualitative for follicle atresia or health, but they complement, or confirm, histological features such as apoptotic bodies or mitotic figures in secondary or antral follicles.
Markers of Ovarian Cancer
Ovarian tumors are generally rare in rodent species. We know from numerous studies, with different tissues, that changes in the levels of proteins implicated in cell–cell interactions can undergo dramatic changes in either tumorigenic cells or during the process of carcinogenesis . Intercellular gap junctional communication is known to be consistently downregulated in carcinogenesis, and this can be induced by chemical carcinogens. For example, in the liver of rats treated for 5 days with hexachlorobenzene (HCB), the expression of the gap junction proteins, Cx32 and Cx26, remains significantly lower 50 days after the beginning of the treatment, and GJIC is still decreased 100 days later, but only in female rats [106]. Interestingly, there is a preponderant increase in cancer formation in these female rats when they are subsequently given diethylnitrosamine (DEN), while connexins in the male are not affected by HCB and males develop significantly fewer tumors in response to DEN [106]. In a study using several ovarian cancer cell lines, most of the cell lines displayed reduced expression of connexins [107]. In granulosa cell tumors, it has been reported that Cx43 is decreased or absent. Interestingly, in cells with decreased Cx43 there is an increase in Cx32, which is normally absent in the normal ovary [108]. Thus, the regulation and interplay between these two connexins provide an interesting set of makers which may be useful in understanding chemical-induced carcinogenic transformation in the ovary. In another study, cisplatin treatment of ovarian cancer cells resulted in the formation of both sensitive and resistant cells. Downregulation of p53 in both cell types with siRNA resulted in downregulation of several genes including Cx43. In fact, cells in which Cx43 expression was decreased showed increased drug resistance to cisplatin [109]. Whether or not drugs that induce transporters, such as those of the ABC transporter family, have lesser effects in cells when Cx43 is downregulated remains to be established. However, dual labeling of connexins and certain known transporters may provide important information on potential drug/chemical toxicity in the ovary.
FSH receptor knockout mice (FORKO ) develop ovarian tumors after 12 months of age [110]. These animals are hypergonadotropic but display hypogonadism and have been used as an animal model for menopause. After 12 months of age, FORKO mice develop different types of ovarian tumors, including granulosa cell tumors and serous papillary epithelial adenomas [110]. Ovaries of FORKO mice show increased levels of the tight junction protein claudins (Cldns) 3 and 4, as well as dramatic increases in Cldn11; Cldn1, on the other hand, is repressed [111]. These animals also display steroid hormone imbalance, with high levels of testosterone and low levels of progesterone [111]. These observations are important with regard to identifying effects associated with long-term exposure to steroid-mimicking endocrine-disrupting chemicals, particularly putative androgenic chemicals such as phthalates [112], or other endocrine-disrupting chemicals that alter the production of gonadotropins. Endocrine-disrupting chemicals are molecules that can mimic or inhibit the action of endogenous hormones on target tissues, or alter either hormone secretion or the synthesis of their receptors [113]. Thus, monitoring the expression levels and immunolocalization of ovarian Cldns or connexins represent strong histological biomarkers to evaluate the effects of these chemicals and whose changes may be associated with infertility or rendering the ovary to a precancerous state.
Toxicants and Mammary Gland Development
Over the past 30 years, many studies have demonstrated that mammary glands develop earlier in women from many countries, as compared to the 1930s–1940s [114–117]. Estrogens , secreted by the ovaries under the influence of the follicle-stimulating hormone (FSH) and the luteinizing hormone (LH), are essential for mammary gland development during puberty [118, 119]. However, precocious breast development is not associated with an earlier menarche, implying an action of estrogens without the activation of the hypothalamic–pituitary axis [120]. It has been suggested that environmental factors mimicking estrogens actions may be responsible for those perturbations [120–122]. Because endocrine disruptors are widespread in the environment, and because their effects at low concentrations can have major impacts on the tightly orchestrated hormone-dependent development of mammary gland, exposure to endocrine disruptors is particularly disconcerting for women [113, 123, 124]. Mice models are typically used to assess developmental defects induced by chemical exposure or genetic modifications on mammary gland development. While whole mounts have been used to visualize gross developmental defects in mammary glands, the recent development of molecular markers, as well as new in vitro models, allows the assessment of subtle changes at the cellular and molecular levels, particularly changes in cell–cell interactions.
Structure of the Mammary Gland
The mammary g land is formed by two compartments, the stroma (or parenchyma) and the epithelium (Fig. 7.4). The stroma physically and nutritionally supports the epithelium [125]. It is composed mainly of adipocytes and fibroblasts but also contains endothelial cells and cells of the immune system, all of which influence mammary gland development [126]. In humans, the mature epithelium resembles a tree-like branching system comprising 15–20 different lobes. These lobes are themselves formed by numerous terminal lobular units of secretory alveoli (also referred to as acini) and converging ducts (Fig. 7.4). The epithelium consists of two layers of cells: an inner layer of luminal cells surrounded by an outer layer of myoepithelial cells (Fig. 7.4), and basement membrane [127, 128]. These structures develop at different stages of mammary gland organogenesis, and result in a substantial remodeling of the gland induced primarily by hormones. Since the structure and development of the murine mammary gland is similar to that of the human, this aspect of the review will focus on the murine mammary gland model.
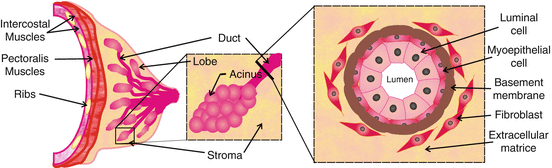
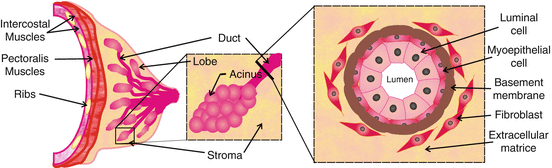
Fig. 7.4
Schematic diagram of the anatomical structure of the mammary gland
Mouse Mammary Gland Development
Embryonic Development
Mammary gland development in mice begins around embryonic day 10.5 with the formation of bilateral milk lines (Fig 7.5a) located on the abdomen of the animal [129]. These milk lines are produced by epidermal cells which become columnar and multilayered. By day E11.5, cells within the milk line migrate to specific locations to form five pairs of lens-shaped placodes (Fig. 7.5a) [130]. During the following days, placodes enlarge and invaginate to form bulb-shaped buds, while cells located directly around the developing buds condense and differentiate, to form the primary mammary gland mesenchyme. The final phase of embryonic development begins around day E15.5, with proliferation of epithelial cells and elongation of the primary sprout into the mesenchyme. Simultaneously, mesenchymal cells located at the neck of the bud and specialized epithelial cells form the nipple at the surface of the body. Once the growing sprout has reached the developing fat pad under the dermis, it gives rise to the rudimentary ductal tree that is present at birth (Fig. 7.5).
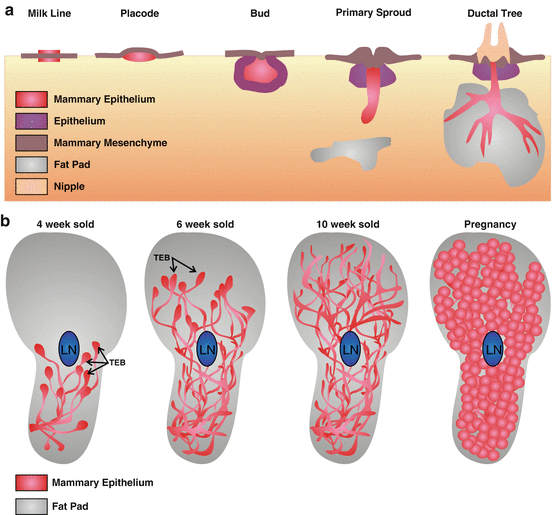
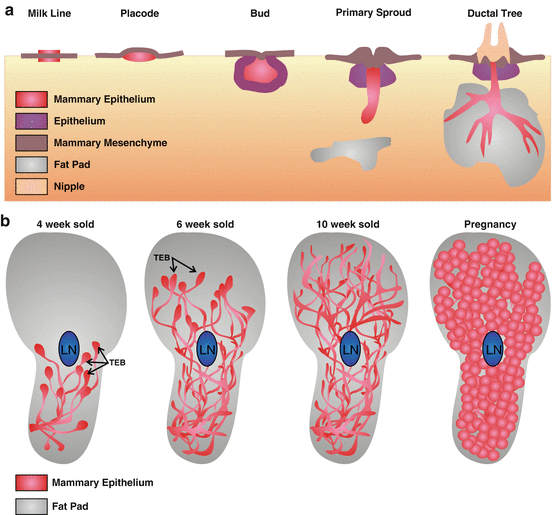
Fig. 7.5
Mammary Gland Organogenesis. Schematic model depicting (a) embryonic mammary gland development, (b) ductal elongation during puberty and alveologenesis during pregnancy. TEB Terminal end bud, LN Lymph Node
Ductal Elongation
After birth, the mammary gland remains quiescent until the beginning of puberty (~4 weeks old), when the rise in circulating hormones and growth factors induces epithelial cell proliferation (Figs 7.5b and 7.7). The ductal elongation occurs in highly proliferating specialized structures located at the tips of the ducts called terminal end buds (TEB) [131]. It has been demonstrated that estrogens and estrogen receptor α (ERα) are required for ductal elongation , since ERα knockout mice display impaired mammary gland development [132–134]. Similarly, the epithelial growth factor (EGF) and the insulin growth factor-1 (IGF-1) are necessary for proper ductal elongation [119]. At the end of puberty, around week 10 (Fig. 7.5b and 7.6), a complex tree-like system of ducts exists throughout the entire stroma and TEBs are no longer present [127]. The epithelium then undergoes cycles of branching and regression with each estrous cycle [127]. Circulating levels of progesterone and progesterone receptor (PR) are necessary for the formation of these tertiary side branches along the ducts. In some strains of mice, lobulo-alveolar structures also develop at the ends of tertiary branches [135].
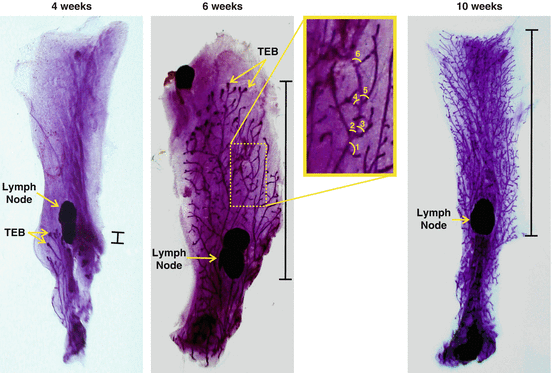
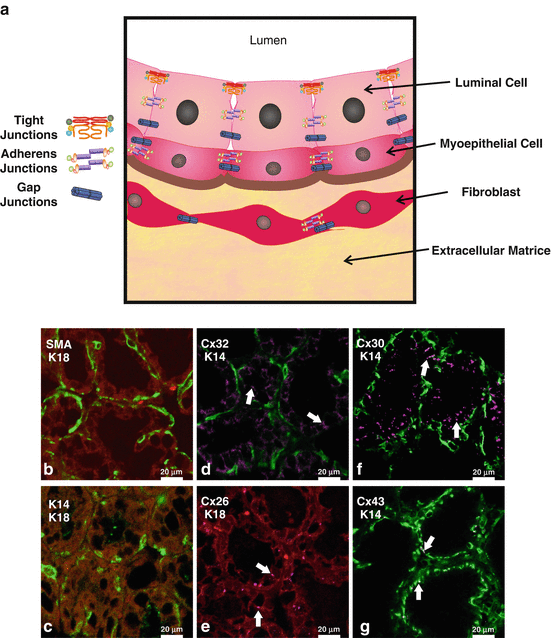
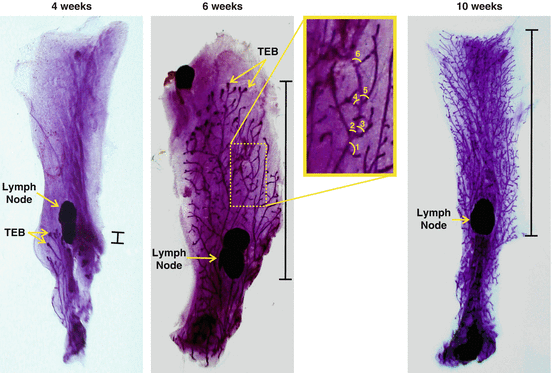
Fig. 7.6
Mammary Gland Whole Mount. Representative pictures of mammary gland whole mounts at 4, 6, and 10 weeks of age showing the lymph node and terminal end buds (TEB). Bars on the right-hand side represent the extent of ductal extensions from the bottom of the lymph node. Inset demonstrates how ramifications can be counted in order to evaluate mammary gland development
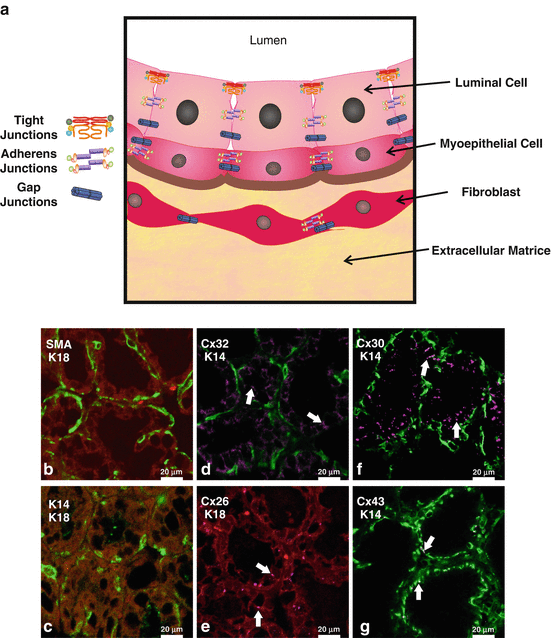
Fig. 7.7
Localization of intercellular junctions in mammary gland structure. (a) Schematic model depicting the localization of tight, adherens, and gap junctions in mammary gland. (b) Representative sections of mice mammary glands at parturition immunolabeled for luminal cell-specific keratin 18 (K18, red; b, c, e), myoepithelial cell-specific keratin 14 (K14, green; c, d, f, g), or Smooth muscle actin (SMA, green; b). Cx32 (d), Cx26 (e), and Cx30 (f) punctate structures typical of gap junction plaques were present at the cell surface of luminal cells while Cx43 (g) (purple, d–g) was present at the surface of myoepithelial cells
Alveologenesis, Lactation, and Involution
At the end of puberty, the mammary gland is not functional. Function, namely milk production and lactation, is gained after another phase of remodeling induced by hormonal changes at the onset of pregnancy [136]. Epithelial cells proliferate and differentiate to form grape-shaped structures, the alveoli, where milk is produced and stored (Fig. 7.5b). Luminal cells inside the alveoli become secretory, while myoepithelial cell bodies and processes extend around the alveoli to form a basket-shaped network [52]. Expression of milk proteins and cell survival and proliferation signals required for alveologenesis are triggered by increased levels of progesterone and prolactin [137]. During lactation, suckling of the pups stimulates the secretion of oxytocin, which induces contraction of myoepithelial cells around the alveoli and along the ducts to transport milk [138]. After weaning, milk accumulation within the alveoli induces a cascade of events resulting in massive epithelial cell apoptosis, which returns the mammary gland back to its mature stage [139].
Endocrine Disruptors and Mammary Gland Development
Mammary gland development is tightly regulated and requires precise levels of hormones at different stages of development. Mammary cells can, therefore, be more susceptible to the detrimental effects of endocrine disruptors and other chemicals at certain periods of exposure. Even though hormones are not required for embryonic mammary gland development [119], studies have demonstrated that in utero exposure to bisphenol A (BPA), genistein, phthalates, atrazine, or vinclozolin results in precocious embryonic development [140], in addition to developmental defects observed during puberty and in adults. These include changes in morphology of the gland, cell proliferation, and gene expression [141–145]. Similarly, perinatal exposure to these chemicals results in increased numbers of both TEB at puberty [146] and alveolar buds in adult mice [147]. Pubertal ductal development involves cell proliferation, differentiation, and apoptosis, all of which are induced by increasing levels of steroid hormones [119]. This period, therefore, is also sensitive to developmental defects induced by exposure to endocrine disruptors [148]. For instance, rat exposed to BPA, diethylstilbestrol (DES), genistein, ep-DDT, Aroclor 1221, Aroclor 1254, or 2,3,7,8-tetrachlorodibenzo-p-dioxin (TCDD) during puberty showed higher than normal rates of mammary epithelial cell proliferation and premature development of lobular terminal ductal structures [149, 150]. Alveologenesis during pregnancy is another period of hormone-induced epithelial cell proliferation and differentiation that can be disturbed by endocrine disruptors. While few toxicological studies have focused on this period of development, it has been reported that exposure to dioxin during pregnancy resulted in poor lobulo-alveolar development and impaired lactation in mice [151]. Similarly, data from genetically modified mice demonstrated that alveologenesis, milk secretion, and lactation were impaired by improper signaling through hormone receptors [152], suggesting that exposure to other endocrine disruptors could result in similar defects.
Together, these data support the notion that exposure to endocrine disruptors, and in particular to those that mimic estrogenic effects, can promote precocious breast development and may contribute to the other effects observed in humans [120–122]. Moreover, the fact that embryonic and perinatal periods of mammary gland development appear to be sensitive to EDs is of particular concern, since many endocrine disruptors are found at higher concentrations in infants and children than in adults [153–156]. Over the years, various methods and models have been developed which can now be used to understand deleterious effects of endocrine disruptors EDs on mammary gland development.
Methods to Study Mammary Gland Development, Function, and Toxicology
One of the classical, and most commonly used, methods to study mammary gland developmental defect is whole mount. Generally, ductal development is assessed by evaluating the number of TEBs, ducts, and sub-branches and the length of ducts during embryonic development, during puberty, and in adults (Fig. 7.6) [157–161]. While alveologenesis can also be evaluated by whole mount, quantification during late pregnancy or lactation stages is difficult due to the density of the tissue. Moreover, whole mount gives only a macroscopic evaluation of the mammary gland structures. As a result, improved histological and imaging technologies are now used to evaluate defects at the molecular and cellular levels.
Imaging the Intercellular Junctions in Mammary Glands
The need for interaction between the epithelium and the stroma to propagate signals generated from hormonal responses was confirmed years ago by numerous transplant and in vitro studies [162–167]. More recently, studies have demonstrated that mammary gland development and function require bridging and connecting of the myoepithelial and luminal cells, a function ensured by gap, adherens, and tight junctions (Fig. 7.5a) [168–171]. As indicated previously in the text, gap junctions are channels formed by connexins, which permit direct communication between adjacent cells [172]. Adherens junctions are composed of transmembrane and cytoplasmic proteins, including cadherins and catenins, and are involved in adhesion between cells and in cell signaling [173]. Tight junctions are composed of transmembrane proteins, claudins, occludin, and tricellulin, bound to a complex of cytoplasmic proteins [174]. Tight junctions form a semipermeable barrier that controls paracellular transport between the cells and allows for cell polarity [173–175]. Studies using genetically modified mice models have shown that proteins forming gap, tight, and adherens junctions are required for proper mammary gland development [158, 176–178]. Moreover, it has been shown that many endocrine disruptors can modulate junctional protein expression in various tissues, resulting in developmental defects and cancer promotion [106, 179–185]. Thus, imaging of junctional proteins represents a significant tool for assessing mammary gland development in toxicological studies.
Luminal or myoepithelial localization of junctional proteins has been traditionally assessed by immunohistochemistry [186, 187] or electron microscopy [171, 188]. However, the increased availability of high-quality antibodies and accessibility to confocal microscopes now allow for easier imaging of the mammary gland components. As with many multilayered epithelia, luminal and myoepithelial cells express different types of cytokeratins (cytokeratins 8/18 (K18) and 14 (K14), respectively) and other markers facilitating the localization of proteins (Fig. 7.7b and c) [189]. Consequently, we and others have demonstrated that in the mouse mammary gland, Cx26, Cx30, and Cx32 are expressed at the plasma membrane of luminal cells, and expression levels of these junctional proteins vary throughout development (Fig. 7.7d–f) [190–192]. Similarly, Cx43 is expressed at the plasma membrane mainly of K14-positive myoepithelial cells and of fibroblasts at all stages of mammary gland development (Fig. 7.7 g) [158, 191].
Cellular junctions are also required for proper function of the mammary gland. Tight junctions, in particular, allow the formation of a lumen by forming a barrier between the milk and the interstitial fluid [188, 193]. Moreover, tight junction closure is required for secretory activation. While imaging technologies can be used to determine proper localization of junctional proteins, tight junction function needs to be assessed by measuring transepithelial resistance [194] or by intraductal injection of a foreign substance, such as [14C]-sucrose into the lumen of the gland [188]. The presence of cytoplasmic lipid droplets (CLD) after parturition is another indication of improper activation of secretion. While the presence of CLDs is normal during late pregnancy, CLDS are typically replaced by small lipid droplets at the apical surface of the epithelial cells after parturition [195]. CLDs can be observed in histological sections (Fig. 7.8a, b) and quantified using adipophillin immunostaining, which coats the surface of CLDs and milk lipid globules [196]. Another phenotype associated with a leaky epithelium or impaired mammary gland function is the presence of milk in the lumen of the alveoli after parturition. To quantify and determine if milk is more frequently found in the alveoli lumens of mammary glands from mutant mice or mice exposed to endocrine disruptors, histological sections of mammary glands can be double stained with a milk protein, such as whey acidic protein (WAP), and lectin wheat germ agglutinin (WGA) (Fig. 7.8c, d). WGA binds to the apical border of the luminal cells, providing a visual indicator of the luminal border of alveoli and ducts. Mis-localization of WGA or increased detection of milk components throughout the lumen can thus reflect developmental or functional defects (Fig. 7.8) [191]. An elegant study recently published suggested that developmental and functional defects can be assessed at different stages of mammary gland organogenesis at the molecular levels using an array of markers referred to as “tissue proteotyping” [197].
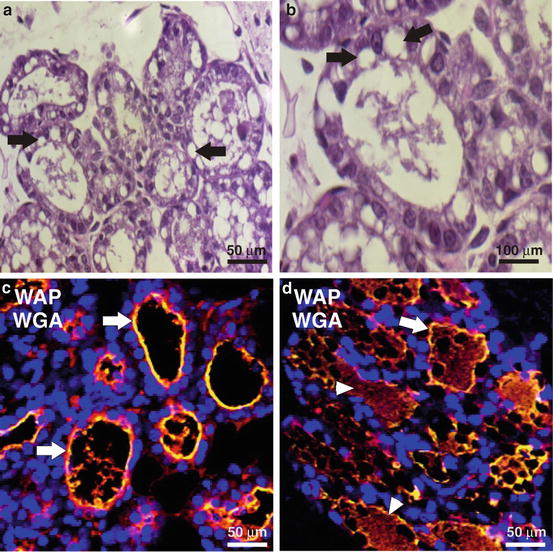
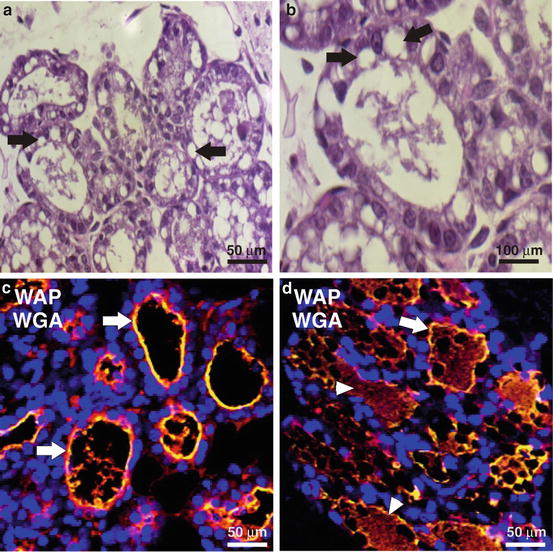
Fig. 7.8
Evaluation of secretion activation and lactation defects using imaging. (a, b) The presence of cytoplasmic lipids droplets (arrows) in histological sections after parturition is an indication of improper secretion activation. Paraffin-embedded mammary gland sections from mice after parturition were stained with eosin and hematoxylin. (c, d) Paraffin-embedded mammary gland sections were labeled with wheat germ agglutinin (WGA, green) to denote the luminal border (arrows) and anti-whey acidic protein (WAP) antibody (red) to indicate the presence of milk proteins (arrowheads)
In Vitro Models for Studying Mammary Glands
A limitation of immunohistochemistry is the ability to visualize the structure of the mammary gland during alveologenesis. While myoepithelial cells surrounding luminal cells in ducts are oriented parallel to the long axis of the ducts, forming a continuous layer that is easily identifiable (Fig. 7.9a), myoepithelial cell bodies and processes extend around the alveoli during pregnancy and lactation, forming a basket-shaped network (Fig. 7.9b) [52]. As a result of these structural changes, developmental defects induced by toxicants or resulting from mutations can be easily observed in luminal cells, but may be undetectable in myoepithelial cells. Moreover, while animal studies are crucial to evaluate the toxicological effects of compounds, they are both cost- and time-demanding. To overcome the limitations of animal studies, many mammary luminal cell lines have been used for in vitro toxicity assays, with excellent results. On the other hand, myoepithelial cells have been mostly ignored, both in developmental and toxicological studies, since it was believed that their role was limited to milk transport and ejection during lactation. We now know that myoepithelial cells are required for proper mammary gland differentiation and function [52, 198–200]. As there are few tools available to study myoepithelial cells, we have developed a new method for the isolation and culture of pure populations of myoepithelial cells from murine mammary glands [158, 191]. Cultured myoepithelial cells are differentiated, as they retain expression of specific myoepithelial markers, and these maintain the capacity to communicate via gap junctions and to contract upon oxytocin exposure (Fig. 7.9c–e). Primary myoepithelial cells offer a new avenue in toxicological studies to understand the effects of toxicants on these important cells.
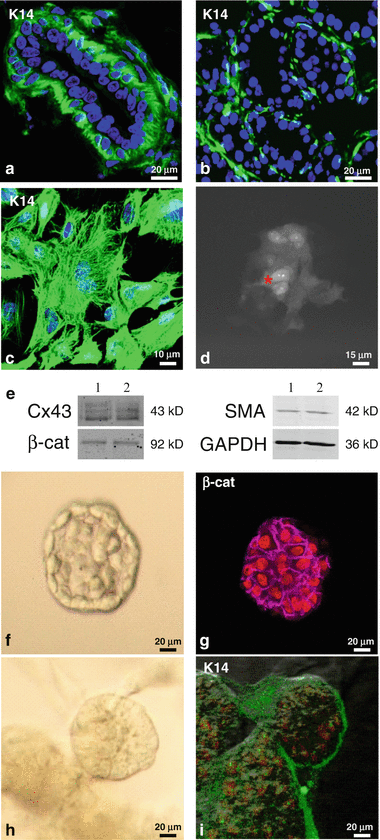
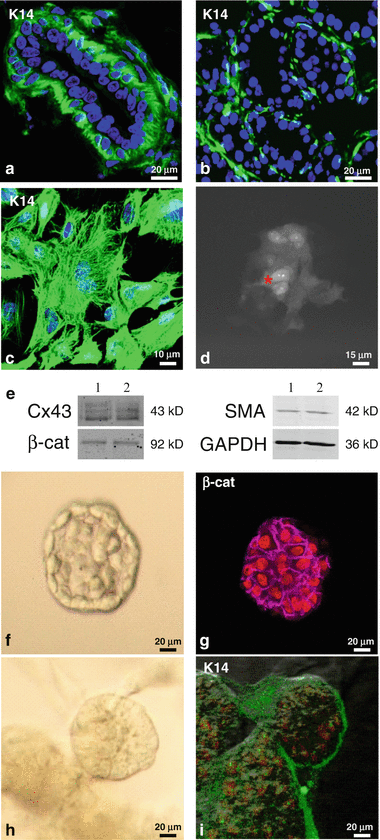
Fig. 7.9
In vitro models to study mammary gland. (a, b) Representative sections of mouse mammary glands at 10 weeks of age (a) or at parturition (b) immunolabeled for keratin 14 (K14, green), a marker of myoepithelial cells, showing the shape and localization of the myoepithelial cells. Fig. 7.9 (continued) Nuclei were stained with Hoechst (blue). (c) Cells isolated from mammary gland were mainly of myoepithelial origin, as confirmed by keratin 14 staining, and were competent in passing Lucifer yellow to neighboring cells (d), an indication of gap junctional intercellular communication. The asterisks indicated which cell was microinjected with Lucifer yellow dye. (e) Western blot analysis indicated that connexin 43 (Cx43), β-catenin (β-cat), and Smooth Muscle Actin (SMA) were expressed in primary myoepithelial cells. Protein levels were normalized to GAPDH. (F-I) MCF12A (luminal) were cultured (f, g) or cocultured with primary myoepithelial cells (h, i) in Matrigel for 7 days. Light microscopy (f, h) and confocal images (g, i) show acini formation by MCF12A (f, g) and organotypic structures (h, i). Cells were immunolabeled for either β-catenin (purple, g) or keratin 14 (green, i). Nuclei were stained with Draq5 (red ). i is superimposed on its own DIC image
Although 2D culture systems are extensively used and are valid models, they do not mimic the 3D structure of organs. Recent studies have used 3D models in which cells are cultured within a matrix containing components of the extracellular matrix. When cultured as such, luminal mammary gland cells form acini-like structures (Fig. 7.7f, g) [180, 184]. An even more innovative approach can also be used, namely, “organotypic” models. Organotypic models consist of a mixture of myoepithelial and luminal cells cocultured within a matrix, resulting in 2-layered acini, thus mimicking more closely the mammary gland structure [201–203]. Human luminal cell lines, such as MCF10A and MCF12A, form polarized double-layered acini when cocultured with primary myoepithelial cells (Fig. 7.9 h, i). Combined with imaging techniques, these new models thus provide a unique opportunity to study the effects of toxicants not only on luminal and myoepithelial cells but also on the junctional interactions between the two epithelial cell layers.
Perspectives and Conclusions
There have been an increasing number of studies that have reported increased use of in vitro fertilization throughout the world. Through the advent of newer in vitro fertilization technologies, many women are waiting until they are older to have babies, thus extending potential exposure period of oocytes to toxicants. It is, therefore, important to elucidate the cellular and molecular processes which occur in the reproductive system. Recent findings provide novel approaches to explore how contaminants may alter cell gonadal development. Furthermore, because the ovary undergoes substantial changes during oogenesis, and because it comprises different cell types, the use of immunolocalization is essential for understanding pathological changes in the ovary. Over the past several years, many studies have reported higher incidences of breast cancer and precocious mammary gland development in women from many countries. Since mammary gland development is highly regulated by steroid and hypophyseal hormones, slight changes in hormonal balance can result in alterations to both development and function. Whether or not precocious mammary gland development contributes to increased chance of breast cancer development later in life, or if the chemicals that stimulate mammary gland development predispose the gland to the development of cancer, remains to be fully addressed. The use of sensitive tools such as markers of cell–cell interactions in both animal and human tissue samples, as well as in vitro cell lines and 3D cell culture models, represents important components of future screening programs aimed at understanding effects of toxicants on both ovary and mammary glands.
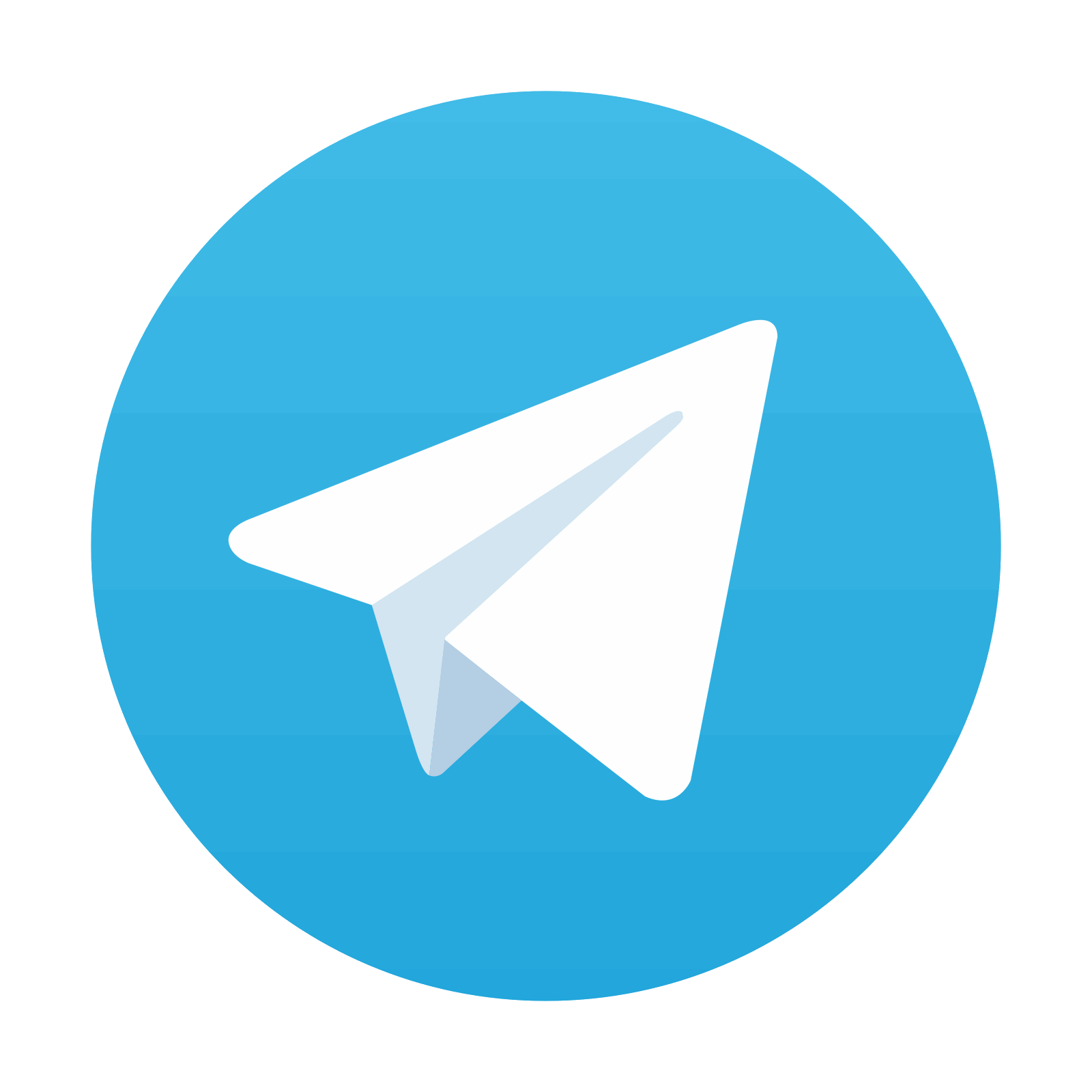
Stay updated, free articles. Join our Telegram channel

Full access? Get Clinical Tree
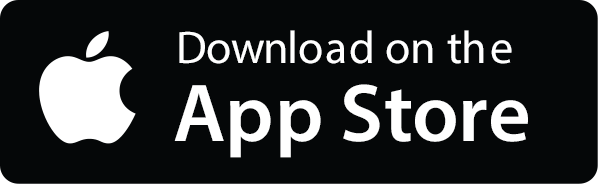
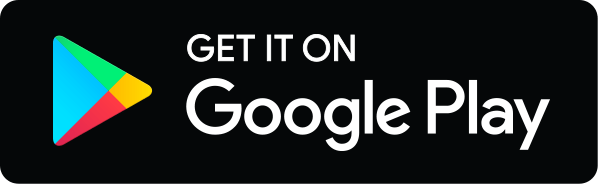