CORONARY ARTERY DISEASE
The heart has the highest metabolic demands when compared to other organs. The vast majority of the energy substrate utilization is expended during unrelenting periodic contraction of the myocardium. Blood flow is delivered at a rate of 1 mL/g of cardiac tissue per minute at rest. Increases in myocardial oxygen consumption, via adenosine diphosphate and adenosine mediated arteriolar vasodilation, can result in a reciprocal increase in blood flow, up to five times normal. This increased blood flow is accommodated by recruitment of an extensive capillary bed within the myocardium, with nearly 1 capillary per myocyte. Between 70% and 80% of available oxygen is extracted from coronary blood flow at rest. Thus, the metabolic needs of the heart are tightly coupled to the availability of coronary blood flow, since additional extraction is limited. In addition, blood flow through the left ventricular epicardial arteries is phasic. During myocardial contraction, extravascular compression of intramyocardial capillaries prevents forward flow during systole, limiting flow to the diastolic phase of the cardiac cycle. This is even more pronounced in the subendocardial region where myocardial oxygen demands are greatest as a result of increased wall tension and greater sarcomere shortening. Because of the elevated and insistent myocardial oxygen consumption, the restriction of blood flow to diastole, and the high basal level of oxygen consumption, the heart is particularly susceptible to ischemic injury related to stenosis of the epicardial coronary arteries.
The heart receives its blood supply from the left and right coronary arteries (Figure 19–1). These epicardial vessels originate as the first branches off of the aortic root, in their respective sinuses of Valsalva. The coronary circulation is traditionally divided into three territories or regions: the left anterior descending (LAD), the circumflex (arising from the left coronary artery), and the right (from the right coronary artery). The dominance of the heart refers to which major artery terminates as the posterior descending branch. Ninety percent of individuals are right dominant, as the right coronary artery supplies the posterior descending artery. The remaining 10% are left dominant, as the terminal branch of the circumflex artery supplies the posterior descending artery.
The left coronary artery is referred to as the left main coronary artery. After its origin in the left sinus of Valsalva, it courses between the left atrial appendage and the pulmonary artery. The left main coronary artery varies in length but is typically less than 2 cm long. It terminates in two branches: the LAD and the circumflex coronary arteries. In less than 1% of patients, the left main artery is absent, with the LAD and circumflex originating as separate ostia from the left sinus of Valsalva.
The LAD, or anterior interventricular artery, courses anteriorly and inferiorly in the interventricular groove toward the apex of the heart. Several branches of the LAD travel along the anterolateral surface of the left ventricle and are known as diagonal arteries. Their number and size are highly variable. Branches to the interventricular septum take off perpendicularly, the first of which is often sizable. The LAD is the most prominent of the three coronary territories and carries approximately 50% of myocardial blood flow.
After arising from the left main, the circumflex coronary artery dives posteriorly along the atrioventricular groove. Several obtuse marginal branches supply the lateral wall of the left ventricle; these branches vary in both size and number. In 10% of patients, the circumflex continues posteriorly and gives rise in its terminal branch to the posterior descending coronary artery, running from the posterior atrioventricular groove toward the posterior apex in the interventricular groove. Some patients have a third branch of the left main referred to as the ramus intermedius. If present, this branch is often large and supplies the anterolateral wall of the left ventricle.
The right coronary artery originates from the right sinus of Valsalva in the aortic root. It courses anteriorly and rightward until reaching the right atrioventricular groove. The vessel then descends around the acute margin of the heart, giving rise to one or more branches supplying the right ventricle. In 90% of patients, the right coronary artery continues posteriorly, terminating as the posterior descending coronary artery and the posterolateral artery.
Atherosclerosis, a progressive multifocal disease of medium and large muscular arteries of the systemic circulation, tends to occur predominantly at vessel bifurcations, sharp curvatures, and other regions creating pressure wave reflections and recirculation. Because of these flow-related considerations, atherosclerotic stenotic lesions are generally restricted to the proximal regions of the large epicardial coronary arteries. In particular, stenosis found in the LAD and circumflex vessels are frequently isolated, short, and in the proximal segments. The right coronary artery, however, develops diffuse obstructions, although rarely extending into the posterior descending or intramural branches.
The pathologic mechanism of coronary atheroma formation is identical to the lesions found elsewhere in the vascular tree. Endothelial injury from cigarette smoke, hypercholesterolemia, hyperglycemia, hypertension, or other causes of inflammation initiate a cascade of events. These include endothelial dysfunction with reduced nitric oxide production, monocyte adhesion and migration, lipid accumulation, and smooth muscle cell proliferation. The end result is an enlarging plaque encroaching on the arterial lumen, separated from the blood stream by a collagen-rich fibrous plaque. The lesion can cause flow limitations, particularly when the luminal cross-sectional area is reduced by at least 75%. With this degree of obstruction, the vasodilatory reserve required during increased myocardial demand is restricted, resulting in transient myocardial ischemia until demand returns to baseline. The atherosclerotic plaque also causes coronary ischemia when the lesion becomes unstable. The fibrous plaque can fracture, causing rupture of the plaque contents and complete epicardial thrombosis, the presumed mechanism of ST-segment elevation myocardial infarction (STEMI). Additionally, subtotal plaque disruption can cause vasoconstriction, platelet activation, and embolization, resulting in ischemia without total occlusion of the epicardial vessel. This is the presumed mechanism of unstable angina and non-ST-segment elevation myocardial infarction (NSTEMI).
Patients with coronary atherosclerosis can present with a variety of symptoms depending on the severity and nature of their occlusive lesions as well as on their other medical comorbidities. Chronic stable angina is the most frequent complaint of the patient with coronary artery disease. At rest, coronary blood flow is adequate to meet myocardial demand, and patients are without symptoms. However, during exercise or stress, as myocardial oxygen demand increases, autoregulatory mechanisms vasodilate to increase myocardial blood flow fivefold to sixfold. Stable coronary obstructions become flow limiting, resulting in an imbalance of oxygen demand and supply. Chest pain develops rapidly and is typically described as tightness, squeezing, constricting, or aching. It is usually midsternal and radiates to the left shoulder, arm, or neck. The classically described Levine sign with a clenched fist over the sternum is a common finding. Some patients, however, describe symptoms that are collectively referred to as “anginal equivalents.” These include dyspnea, diaphoresis, nausea, heartburn, and dizziness or presyncope. Although the clinical manifestations of angina are variable, the pathognomonic feature of chronic stable angina is that the symptoms occur predictably with exertion and are always relieved with rest.
Acute coronary syndrome (ACS) encompasses a spectrum of conditions related to coronary occlusive disease, including unstable angina, NSTEMI, and STEMI. Patients with unstable angina experience chest pain or an anginal equivalent that is new, occurs at rest, or occurs with increasing severity from their baseline chronic stable symptoms, also called crescendo angina. Patients who develop NSTEMI have evidence of myocardial injury with elevated blood levels of myocardial enzymes (troponin and the myoglobin [Mb] fraction of creatine kinase). Unstable angina and NSTEMI are important prognostic indicators, as 10% of patients will die of cardiovascular causes within 6 months.
STEMI represents the consequences of large epicardial vessel occlusion typically associated with plaque rupture. Patients usually describe severe retrosternal chest pain that persists for more than 30 minutes. Patients with previous chronic stable angina will report that the current pain does not resolve with rest or nitroglycerine and is more intense in quality. Patients often describe additional symptoms such as diaphoresis, nausea, and dizziness. Although improvements in health systems have drastically increased survival from myocardial infarction, mortality for STEMI remains near 10%.
Patients with suspected coronary artery disease should undergo a resting electrocardiogram. Although most patients with chronic stable angina have a normal electrocardiogram pattern, evidence of previous myocardial infarctions may be identified with the presence of either Q waves or conduction abnormalities. The most widely used diagnostic test to evaluate for coronary artery disease is exercise electrocardiography. Using standardized protocols, patients are exercised on a treadmill or bicycle ergometer while a 12-lead electrocardiogram is continuously recorded. The test is continued until the patient’s symptoms are noted or until the development of significant ST segment shifts suggesting myocardial ischemia. The diagnostic accuracy of exercise stress electrocardiogram testing can be enhanced with myocardial perfusion imaging. Several radioactive tracers are used clinically, the most frequent of which is thallium-201. Because of its similarities to potassium ions, it is taken up preferentially by the viable cardiac myocytes. Its distribution within the myocardium is proportional to the rate of perfusion. In some cases, patients are unable to exercise because of additional physical or psychological limitations. Pharmacologic agents can substitute for exercise by increasing myocardial oxygen demand (dobutamine) or by directly vasodilating coronary arteries (adenosine), thus demonstrating regions with fixed restrictions in myocardial blood flow. Echocardiography can be used as an alternative to nuclear perfusion imaging to increase the accuracy of exercise electrocardiogram testing. Echocardiography demonstrates regional changes in wall motion that can be observed during myocardial ischemia. It can also identify valvular abnormalities or other conditions that may influence treatment choices.
Coronary angiography, also known as cardiac catheterization, is indicated in symptomatic patients with suspected coronary occlusive lesions. After percutaneous access is obtained in the arterial system, preformed catheters of varying sizes are advanced fluoroscopically to selectively engage the ostia of the left and right coronary arteries. Radiopaque contrast is injected with imaging of the opacified coronary artery. Standardized views are obtained of both the right and left coronary systems to provide different projections to clearly define the vascular anatomy and to quantify the severity of occlusive lesions (Figure 19–2). Additionally, catheters can be inserted across the aortic valve into the left ventricular cavity. Contrast injection for ventriculography can provide information about ventricular systolic function, cavity size, and the presence of left-sided valvular abnormalities. During cardiac catheterization, stenotic lesions in the epicardial vessels can be treated using percutaneous techniques, described in the section on Percutaneous Intervention. Newer imaging techniques using high-resolution multislice CT scanning with 3D reconstruction and magnetic resonance imaging (MRI) are increasingly utilized. These noninvasive approaches have the potential to improve the safety and convenience of coronary imaging; however, resolution remains inferior to standard coronary angiography.
Medical therapy of coronary artery disease begins with controlling risk factors that contribute to formation and destabilization of the atherosclerotic plaque. The most important intervention is cessation of cigarette smoking. Other interventions include control of hypertension, diabetes, and hypercholesterolemia. Dietary modifications and exercise can improve all of these conditions, but pharmacologic treatment is often necessary.
Statins can improve cholesterol levels and improve the ratio of low-density to high-density lipoproteins, and they have been demonstrated to reduce rates of myocardial infarction and death. Angiotensin-converting inhibitors have been shown to reduce mortality and myocardial infarction in patients with coronary artery disease coupled with hypertension, diabetes, or left ventricular dysfunction. Aspirin, by inhibiting platelet activity, reduces death and myocardial infarctions in patients with coronary artery disease and should be prescribed to all patients unless significant contraindications exist. Beta-blocking agents reduce myocardial oxygen consumption by reducing heart rate and wall tension, and they increase oxygen delivery by increasing the diastolic phase and thus subendocardial perfusion. Despite these perceived benefits, beta-blockers have not been shown to reduce cardiovascular mortality or morbidity in patients without left ventricular dysfunction or hypertension. Nitrates also decrease myocardial oxygen consumption via venodilation, reducing cardiac preload and waltension, and vasodilation, reducing afterload. Some epicardial vasodilation will occur, improving coronary blood flow. Nitrates can control symptoms, either immediately when given sublingually or prophylactically when used as an oral long-acting agent. Headaches are notable side effects, and the vasodilatory properties of nitrates can be exaggerated by phosphodiesterase inhibitors frequently used for the treatment of erectile dysfunction. Medical treatment alone is appropriate for patients with coronary artery disease affecting one or two epicardial vessel territories and satisfactory symptom control.
In 1977, Dr. Andreas Gruentzig performed the first percutaneous intervention with balloon angioplasty on a stenotic lesion in the LAD. This pioneer laid the foundation for a revolution in the treatment of coronary artery disease worldwide. Percutaneous coronary intervention is among the most frequently performed medical procedures in the world, and its use continues to expand. These interventions include balloon angioplasty, intracoronary stent implantation, as well as rotational and laser atherectomies. Using the same techniques described for cardiac catheterization, small, highly flexible and steerable guidewires can be advanced percutaneously down the lumen of stenotic epicardial coronary arteries. Over this guidewire, balloon tipped-catheters can be advanced across the stenosis and inflated to supra-atmospheric pressures. This stretches and dilates the affected vessel, restoring the lumen to its predisease dimensions. Restenosis with balloon angioplasty was common, occurring in nearly 40% of patients. The use of nitinol scaffolding stents has greatly reduced restenosis rates to nearly 15% and they are now used in nearly 90% of percutaneous procedures in the United States. The latest addition in the armamentarium of percutaneous coronary interventions is the drug-eluting stent. The drugs impregnated into the walls of the stent are antiproliferative, similar to agents used to prevent replication of immune cells in transplant recipients. The sirolimus eluting and paclitaxel eluting stents have further reduced the rate of target vessel restenosis and the need for repeat interventions. However, reports of late stent thrombosis have raised concerns, and indefinite use of antiplatelet regimens has been recommended.
Although alternative surgical approaches to myocardial ischemia had been attempted previously, René Favaloro is credited with creation of the coronary bypass procedure from his work at the Cleveland Clinic. Since its inception in 1967, coronary artery bypass grafting (CABG) has increased in volume until the last decade, with minimal growth, presumably from improved percutaneous and medical treatments (Figure 19–3). Despite the recent trends, CABG continues to be among the most frequent, successful, and well-studied procedures performed in medicine.
Three large prospective multicenter randomized clinical trials evaluating CABG versus medical therapy are widely quoted and should be understood when considering the indications for coronary bypass grafting. Despite the historical nature of these studies and the evolution in both surgical technique and medical treatment, these trials continue to provide important information about the benefits of surgical revascularization in patients with advanced coronary atherosclerosis.
The Veterans Administration Cooperative Study enrolled 1000 patients between 1970 and 1974. Patients had chronic anginal complaints and were free of myocardial infarction within 6 months. Medical treatment consisted largely of nitrates and aspirin, and surgical mortality was extremely high when compared to contemporary results. A statistically significant survival advantage was seen in the surgical group versus the medical group at 7 years (77% vs 72%), despite 38% of medical patients crossing over to surgical treatment. Subgroup analysis demonstrated more pronounced survival advantages for patients with three-vessel disease, patients with left ventricular dysfunction, and patients with significant stenosis of the left main coronary artery.
The European Coronary Surgery Study recruited 767 men with chronic angina from 1973 to 1976. Only patients with preserved left ventricular function were included. A survival advantage for the surgical group was seen in the overall cohort, but particularly in patients with three-vessel disease and those with proximal LAD stenosis. There were also advantages demonstrated in reduction of angina and exercise capacity.
The Coronary Artery Surgery Study enrolled patients from a nonrandomized registry of patients who underwent coronary angiography from 1974 to 1979 at 15 centers. Patients with mild angina were randomized to medical treatment and CABG. There was no survival advantage in the 790 randomized patients; however, the cohort with left ventricular dysfunction had better survival with surgery, particularly those with left ventricular dysfunction and three-vessel disease. Evaluation of the nonrandomized patients in the registry also found survival advantages for surgically treated patients with left main stenosis or left main equivalent stenosis.
Although these three landmark studies are nearly 30 years old, some important principles continue to apply: patients with more advanced coronary atherosclerosis, particularly those with left ventricular dysfunction, stand to achieve the most benefit from surgical revascularization.
Numerous clinical trials have more recently compared CABG with percutaneous interventions. The Randomized Intervention Treatment of Angina (RITA) trial compared balloon angioplasty with CABG for patients with single and multivessel disease. There was no difference in survival, but repeat intervention was required five times more frequently in the angioplasty group. The Bypass Angioplasty Revascularization Investigation (BARI) enrolled 1829 patients with chronic or unstable angina from 1988 to 1991. There was no survival advantage at 5 years; however, 31% of the patients in the percutaneous group crossed over for CABG. The need for repeat revascularization was again five times higher in the percutaneous group, and the subgroup of patients with diabetes had improved survival at 5 years (81% vs 66%).
A recent comparison between patients with multivessel coronary disease treated with PCI versus CABG was performed using Medicare claims data as well as the Society of Thoracic Surgeons Database and the American College of Cardiology Foundation PCI Data Registry. Adjusted and unadjusted survival rates were superior for those undergoing CABG at 4 years. As technology and pharmacology continue to evolve, additional studies will be required. However, CABG offers survival and quality-of-life advantages in selected patients with coronary artery disease. The most recent recommendations from the American Heart Association and the American College of Cardiology regarding the indications for coronary bypass surgery are presented in Table 19–1.
Asymptomatic
Symptomatic Stable angina
Unstable angina/NSTEMI
STEMI
Decreased LV function
Failed PTCA
|
The principles of coronary bypass surgery are to restore normal myocardial perfusion by creating alternative routes for blood to reach the jeopardized territories. This strategy has several advantages, including the large-caliber conduits, their extramyocardial location in avoiding the compressive forces during systole, and their attachment at strategic locations to maximize return of normal blood flow. The most important aspect of the procedure is construction of a technically sound and strategically well-created bypass graft.
A variety of conduits may be chosen; the most traditional and still most frequently utilized is the saphenous vein. The vessel is easily harvested with minimal morbidity, and it is technically easy to create the precise anastomosis. Minimally invasive approaches using endoscopic techniques now reduce the impact on the patient. Limitations of the saphenous vein are primarily based on its tendency to develop accelerated atherosclerotic lesions. The vein graft plaque is more frequently circumferential and diffuse, with a weaker fibrous cap and a higher predilection for distal embolization. Patency of saphenous vein grafts is approximately 50% at 10 years. In addition, suitable vein conduit may not be available, either harvested for previous coronary or peripheral bypass procedures or unusable from varicosities or sclerosis.
The left internal mammary artery can be mobilized from its pedicle on the left subclavian artery and anastomosed to the anterior or lateral epicardial vessels of the heart, most frequently to the LAD. This strategy has several well-described advantages owing to the improved patency rate of the internal mammary graft when compared to saphenous vein grafts. The right internal mammary artery can also serve as a bypass conduit; however, harvesting of both internal mammary vessels increases the risk of sternal ischemia and surgical wound-healing complications. Because of the improved patency of arterial grafts as compared to saphenous vein grafts, the radial and gastroepiploic artery have been explored as alternatives to vein grafts. However, improved long-term patency has not been reliably established, and morbidity from harvesting has slowed widespread adoption of this approach.
The standard approach for coronary bypass grafting is via the median sternotomy, where the sternum is divided longitudinally, exposing the heart and great vessels. The left thoracotomy could be alternatively used, particularly after previous heart surgery where sternal reentry could hazard injury to adhesed cardiac structures or patent grafts. Preparation is then made to institute cardiopulmonary bypass (CPB). Anticoagulation with 300 IU heparin per kilogram is infused to achieve an activated clotting time of greater than 400 seconds. Typically, the ascending aorta is used for arterial inflow, and venous return is accomplished via a cannula in the right atrial appendage (Figure 19–4). CPB is commenced, evacuating venous blood into the cardiotomy reservoir. Mechanical ventilation can be discontinued. Using a heat exchanger, the blood is actively cooled to 28-32°C to reduce tissue oxygen requirements and organ injury. Shed mediastinal blood can be scavenged and returned to the CPB system, reducing blood loss during the anticoagulation period. Cardioplegic arrest is then initiated by cross-clamping the ascending aorta and infusing cold blood cardioplegia solution into the aortic root. The makeup of cardioplegia solutions differs among centers and even among surgeons; however, most centers combine autologous blood obtained from the CPB system with crystalloid solution cooled to 12°C containing citrate to bind ionic calcium, dextrose, pH buffers, and potassium (~ 30 mM/L) to arrest all cardiac activity. Cardioplegia is administered intermittently to maintain myocardial temperature and diastolic arrest during the cross-clamp period.
With the arrested heart, a dry and motionless surgical field is created, allowing creation of precise surgical anastomoses on even the smallest of epicardial coronary arteries. The targets are identified on the epicardial surface, and the sites for anastomotic reconstruction are determined on the basis of information from the preoperative cardiac catheterization and suitability of the native vessel. An arteriotomy is created on the exposed vessel, and it is extended for approximately 5 mm. The conduit is fashioned with an appropriately sized bevel or spatulation, and the anastomosis is created, typically in running fashion with fine polypropylene suture. The conduits are tested for patency and hemostasis, and they are cut to the appropriate size, avoiding tension or kinking. Saphenous vein or free arterial conduits are typically connected to the ascending aorta. A 4-5 mm punch device is used to create a circular aortotomy. The anastomoses are constructed in running fashion with polypropylene suture. If conduit length is limited, the proximal anastomoses can be created as Y grafts off of other vein grafts or off of the pedicled internal mammary artery graft.
After completion of all anastomoses, weaning from CPB is prepared. The patient is warmed to normothermia. As the heart begins to warm, ventricular fibrillation often occurs, requiring electrical defibrillation. Temporary conduction abnormalities may require epicardial pacing, but it is often transient. Mechanical ventilation is resumed, and the patient is gradually weaned from CPB. Pharmacologic inotropic support may be required but is often unnecessary if ventricular function was preserved preoperatively. An appropriate dose of protamine is infused to reverse the effects of heparin, and cannulae for bypass are removed. Once hemostasis is adequate, chest closure is performed with stainless steel wires. The pericardium is typically left open to avoid constriction of the atria or kinking of the bypass grafts. The pedicled left internal mammary artery graft is positioned posterior to the anterior surface of the left lung to protect it from injury should sternal reentry be required in the future.
Recently, attempts to reduce the invasive nature of coronary bypass grafting and the potential complications of CPB have been introduced. Techniques to perform bypass grafting without CPB have improved and are promoted by its advocates. Off-pump coronary bypass grafting (OPCAB) have potential advantages in reducing neurologic complications associated with air and atheroemboli, as well as reducing blood transfusion requirements and cost. The procedure involves manipulation and stabilization of the beating heart to expose the epicardial targets. Particularly for vessels on the posterior and posterolateral surfaces, hemodynamic instability can result while the heart is elevated and rotated for optimal exposure. The anesthesiologist must be capable of responding to these rapid changes, and the surgeon must have the judgment and ability to immediately abandon off-pump attempts and institute CPB before significant organ injury occurs. The anastomoses are more challenging, with blood in the moving operative field. Although several single-center reports have demonstrated satisfactory short-term results and mid-term graft patency rates, multicenter randomized and observation studies have suggested that OPCAB techniques may offer some benefits but at the expense of reduced graft patency rates and increased incomplete revascularization. As a result, OPCAB penetrance has not markedly increased over the last several years.
Since the creation of the Society of Thoracic Surgeons voluntary reporting database in 1989, short-term outcomes of coronary bypass grafting are widely available to the public, providing information for patients, referring physicians, and practicing surgeons to compare their results to national averages. The Society also provides a validated risk assessment scoring system, creating mortality estimates based on an individual patient’s clinical data. Overall, risk of perioperative death is 1%-3%. Multivariate predictors of death include advanced age, recent myocardial infarction, decreased ventricular function, renal insufficiency, and female gender. Conduit patency is determined from clinical trials that included angiographic assessments at varying time intervals in the absence of symptoms. Data are largely historical and are subject to biases of patient selection but are largely considered to be reliable. Saphenous vein grafts occlude 20%-30% by 1 year. Early graft loss is felt to be attributable to anastomotic imperfections or graft kinking, endothelial injury during harvest, limited native coronary runoff, or progression of native occlusive disease. Late failures appear to occur at a rate of 5% per year, with 10-year patency approximately 40%-50%. Late failures are primarily attributed to accelerated atherosclerosis of the vein conduit. The pedicled internal mammary artery has far superior patency, particularly when anastomosed to the LAD. With adequate target vessel runoff, 10-year patency rates of 90%-95% have been reported in multiple independent studies. Radial arteries and free mammary artery conduits appear to have patency rates intermediate to saphenous vein and pedicled mammary arteries and are more frequently utilized in younger patients.
VALVULAR HEART DISEASE
The mitral valve separates the left atrium from the left ventricle. It should be considered as the sum of its three components: the leaflets, the annulus to which the leaflets attach, and the subvalvar apparatus, consisting of the cords and papillary muscles. The mitral valve has two leaflets, anterior and posterior (Figure 19–5). The anterior leaflet is larger in surface area, but its attachment to the annulus represents only one-third of the circumference. The anterior portion of the mitral valve annulus is in direct continuity with the annulus of the left and noncoronary cusps of the aortic valve, also known as the aortomitral continuity. The posterior leaflet is shorter, but its annular attachments cover two-thirds of the circumference. The posterior leaflet often can be separated into three distinct scallops, although the prominence of these separations varies among individuals. The anterior and posterior leaflets are separated from each other by the anterolateral and posteromedial commissures, which mark the location of the right and left fibrous trigones respectively. The trigones are dense collagenous structures within the annulus representing a portion of the fibrous skeleton of the heart. The mitral annulus is elliptical in shape, and its dimensions change dynamically during cardiac contraction, reducing its cross-sectional area by as much as 40%. The anterolateral and posteromedial papillary muscles are vertically oriented bundles of cardiac myocytes. Chordae tendineae originate from the heads of the papillary muscles and span the distance to both the anterior and posterior mitral valve leaflets. Chords are designated as primary if they attach to the leading edge of the leaflet, secondary if they attach to the ventricular surface of the leaflets, or tertiary if they attach to the ventricular surface of the mitral annulus. The chords play an important role in preventing leaflet prolapse. The posteromedial papillary muscle is prone to ischemic injury, since it relies on a single right coronary artery for circulation. In contrast, the anterolateral papillary muscle gains its blood supply from the LAD and circumflex branches and tends to be more resistant to ischemic injury.
Regurgitation through the mitral valve can result from a variety of pathologic conditions. Alain Carpentier developed a simplified classification scheme based on leaflet motion (Table 19–2) to organize the different disease processes that can cause mitral regurgitation. In Carpentier type I mitral regurgitation, the leaflet motion is normal. The regurgitation is a result of either dilation of the annulus, as can be seen in cardiomyopathy with progressive ventricular enlargement, or of leaflet perforation, as can be seen with destructive endocarditis. Type II is associated with excessive leaflet motion. Patients with type II mitral regurgitation have ruptured chordae or papillary muscles from ischemia or endocarditis, or they have with pathologically redundant mitral leaflet tissue. Redundant, prolapsing, or myxomatous mitral leaflets can be either acquired from fibroelastic deficiency or hereditary from weak connective tissue. In either case, the excessive leaflet motion prevents proper coaptation of the anterior and posterior leaflets. Patients with type III have restricted leaflet motion. Type III is often associated with rheumatic heart disease, where leaflets can become calcified, and the chords are thickened and foreshortened. The leaflets do not adequately rise in systole, and coaptation is impaired. Alternatively, some patients develop severe mitral regurgitation owing to ischemic injury. Typically, a previous myocardial infarction has resulted in ventricular remodeling, resulting in dilation and retraction of the papillary muscles. As a result, the leaflets are tethered into the ventricle, limiting mobility and preventing proper coaptation.
Mitral regurgitation is always pathologic but can be tolerated surprisingly well when the onset is gradual, allowing for a series of physiologic adaptations. On the other hand, acute mitral regurgitation, as can be associated with infective endocarditis or ischemic papillary muscle rupture, results in immediate pulmonary congestion because the unprepared left atrium is incapable of handling the additional volume load. Several compensatory mechanisms occur as mitral regurgitation develops and progressively worsens. The left atrium and pulmonary venous system gradually dilates, thus increasing compliance to better accommodate the excess volume. The backwards flow of mitral regurgitation reduces ventricular afterload, reducing myocardial wall tension. The reduction in forward flow is accounted for by increased diastolic filling, increasing preload. This maintains cardiac output and can delay onset of symptoms for significant time. Gradually, left ventricular end diastolic volume continues to rise, resulting in pathologic remodeling, creating a dilated and more spherical ventricular cavity. Systolic function is progressively and inexorably impaired as a result of both mechanical considerations owing to the altered shape and molecular mechanisms both intracellular and extracellular. A vicious cycle ensues, with deteriorating systolic function and rising end diastolic volume promoting further ventricular remodeling and dilation and worsening mitral regurgitation. Longstanding mitral regurgitation will result in sustained elevation in left atrial pressure and volume, resulting in pulmonary vascular changes, pulmonary hypertension, and eventually, right ventricular dysfunction.
Acute mitral regurgitation is poorly tolerated and is typically associated with pulmonary congestion and low cardiac output. Patients describe dyspnea, poor exercise tolerance, and fatigue. Often, the etiology of the mitral regurgitation is more dominant in the clinical presentation. Patients with acute endocarditis may have fever, shaking chills, and manifestations of septic embolization such as stroke and intestinal or extremity ischemia. Patients suffering from acute myocardial infarction with a ruptured papillary muscle complain of chest pain and diaphoresis.
Chronic mitral regurgitation can be asymptomatic for many years as a result of progressive atrial and ventricular adaptation. Eventually, patients develop symptoms of heart failure, including dyspnea, fatigue, and lower extremity edema. When left atrial dilation results in atrial fibrillation, patients may describe heart palpitations. Often, the onset of atrial fibrillation is the initial presentation of symptoms, as rapid ventricular response decreases diastolic filling time and creates a sudden reduction in cardiac output. As ventricular function deteriorates and pulmonary vascular changes occur, signs of right-sided heart failure develop, such as lower extremity edema and ascites.
The physical findings of mitral regurgitation vary depending on the duration and the degree of compensation. For patients with longstanding mitral regurgitation, ventricular dilation displaces the point of maximal impulse (PMI) laterally. On auscultation, an S3 gallop is often heard, resulting from increased diastolic flow. The systolic murmur is characteristically described as blowing, best heard over the cardiac apex and radiating to the axilla. In acute mitral regurgitation, the murmur tends to be limited to early in systole. With more chronicity, the murmur is progressively holosystolic.
The chest radiograph often demonstrates cardiomegaly from ventricular dilation. With severe uncompensated heart failure, pulmonary edema may be evident; however, this is more commonly seen with acute mitral regurgitation. The electrocardiogram is often nonspecific but may demonstrate evidence of previous myocardial infarction and will confirm the presence of atrial fibrillation.
Echocardiography is the mainstay in the diagnosis of mitral regurgitation; it provides information about the mechanism of the disease, which is essential in planning surgical intervention. Images of the leaflets can determine if the leaflet motion is normal, restricted, or excessive. Information about annular size and mobility can be obtained. Using color Doppler, the size and direction of the regurgitant jet can quantify the severity and give clues as to the mechanism (Figure 19–6). The severity of the regurgitation is determined by the width and length of the regurgitant jet or the presence of reversed flow within the pulmonary veins. Echocardiography also provides information about the chronicity of the disease and can document progressive adaptation such as left atrial and ventricular dilation. This information is often used in determining timing of surgical intervention, particularly in asymptomatic patients. Echocardiography is usually performed transthoracic. However, in some patients, the views are obscured by body habitus or emphysema, limiting the image quality. Transesophageal echocardiography (TEE) can improve the resolution of images and better clarify the severity and mechanism of the mitral valve pathology.
Cardiac catheterization is an important adjunctive tool, helping to identify additional cardiac pathology, and can determine the adequacy of preoperative medical optimization. Coronary angiography is performed preoperatively to identify coronary arterial occlusive lesions that may require bypass grafting at the time of mitral valve repair or replacement. Although contrast ventriculography can demonstrate the regurgitant jet, it is no longer necessarily used to quantify the regurgitant volume, since echocardiography has become the standard technique. Right heart catheterization will demonstrate intravascular volume overload and low cardiac output. It may also be helpful in diagnosing pulmonary vascular changes in patients who deny symptoms.
The indications for operation on the mitral valve depend on the specific pathology as well as clinical symptoms. In addition, the indications have evolved in recent years owing to improved outcomes related to better surgical techniques, anesthetic considerations, myocardial protection, and postoperative care. Furthermore, intervention on the mitral valve may be performed for less severe disease if operation is indicated for coronary artery disease or aortic valve pathology.
Certainly, among patients who represent reasonable operative risks, those with severe mitral regurgitation and heart failure symptoms should be offered an operation. In addition, those with severe mitral regurgitation and signs of left ventricular dysfunction should undergo operation because myocardial decompensation can progress rapidly without corrective action. There is insufficient evidence that operative intervention for asymptomatic severe mitral regurgitation and normal ventricular function improves survival. Historically, these patients were observed with close clinical follow-up and serial echocardiography. Signs of left ventricular dilation, dysfunction, or new-onset symptoms prompted surgical referral. Some have argued that the presence of pulmonary hypertension or atrial fibrillation represent maladaptive changes and suggest surgical correction.
Surgical techniques of addressing the mitral valve have changed dramatically in recent years. Increasingly, operations have focused on repair rather than prosthetic replacement of the mitral valve. Valve preservation has several advantages. The interaction of the mitral valve and the left ventricle goes beyond just competence and the handling of blood volume. A complex interdependence exists between ventricle and valve, and long-term ventricular function depends on its relationship with the mitral annulus, papillary muscles, and chordae tendineae. Surgical techniques to correct mitral regurgitation that preserve these relationships are more likely to maintain normal ventricular function. In addition to the effects on ventricular function, prosthetic mitral valves carry limitations. Bioprosthetic devices (porcine or bovine) have limited durability because of structural valvular degeneration. The rate of bioprosthetic valve failure is proportional to age, with faster deterioration in younger patients. Mechanical valves are more durable but require lifelong systemic anticoagulation with warfarin. Because of the limitations imposed by prosthetic valves (durability and anticoagulation), surgical referral for mitral regurgitation is often delayed if there is a high likelihood of valve replacement. If valve repair is expected because of mitral pathology and surgeon experience, earlier operation for mitral regurgitation is often considered, particularly in the absence of symptoms or ventricular dilation.
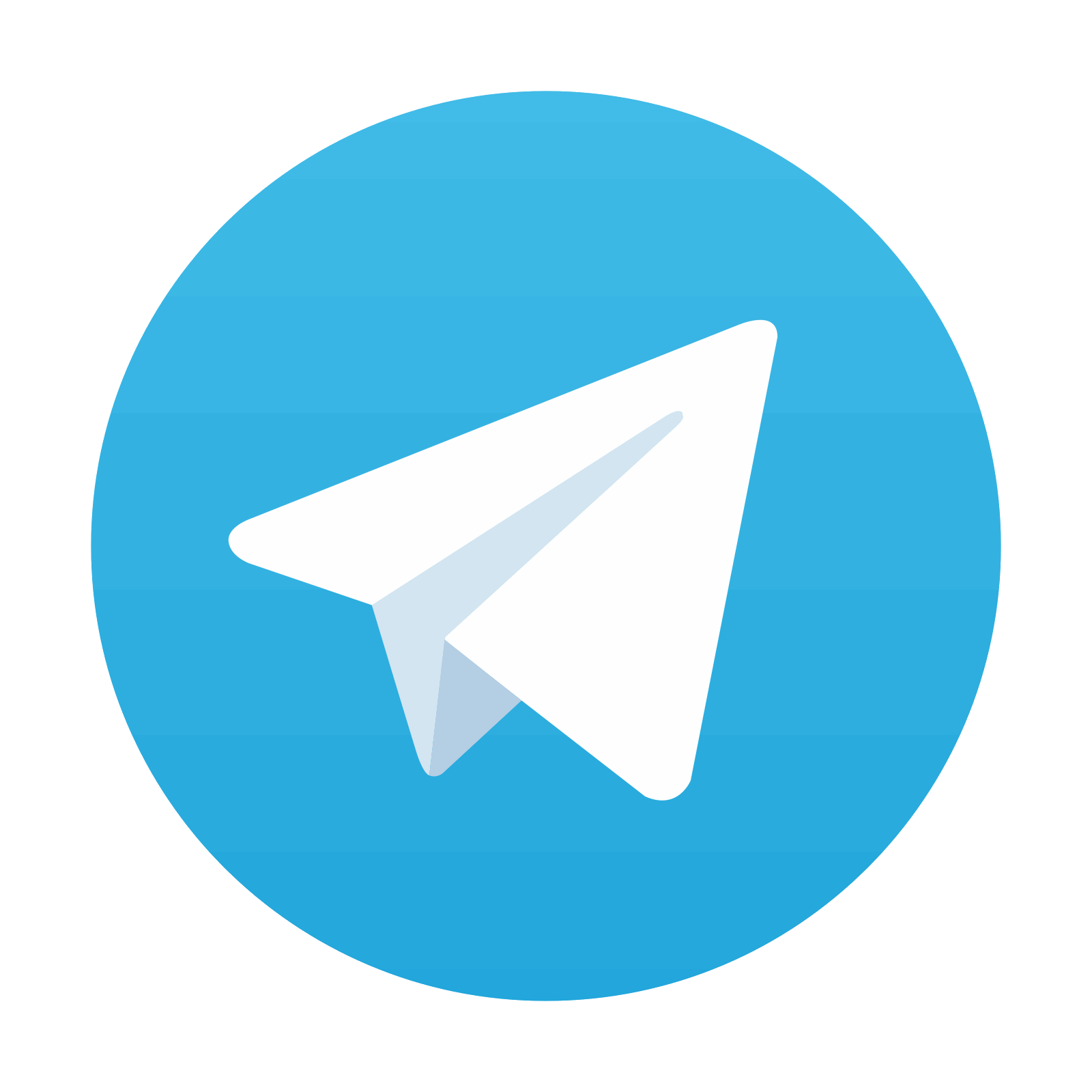
Stay updated, free articles. Join our Telegram channel

Full access? Get Clinical Tree
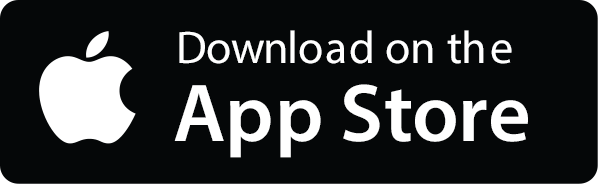
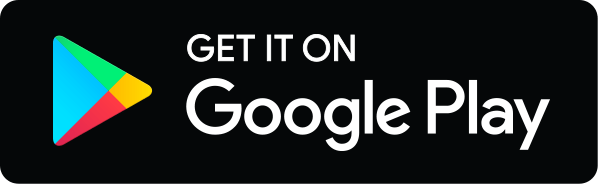