9.1 Case Studies of Human Population Structure
The three case studies chosen here (out of hundreds, if not thousands, of possibilities) show how culture, environment, and geography have affected the genetic structure of local populations in three parts of the world—New Guinea, northwestern Europe, and South America.
9.1.1 The Gainj and Kalam of New Guinea
A number of studies have investigated the patterns of migration and genetic diversity in the Gainj- and Kalam-speaking Melanesian populations that live in the northern part of the central highlands of Papua New Guinea (e.g., Wood et al. 1985; Long 1986; Long et al. 1987; Smouse and Long 1992). The Gainj and Kalam are neighboring groups that speak different languages, but live in a similar environment and are both tribal horticulturists. The Gainj and Kalam live in small groups known as parishes that range in size from 20 to 200 individuals. Migration and genetic data have been collected for 18 parishes. Geographically, these parishes are arranged in a more or less straight line within river valleys, with predominantly Gainj speakers at one end, predominately Kalam speakers at the other end, and several parishes that have a mixture of languages in between. People travel from one parish to the next along a narrow dirt trail. All parishes are within 40 km walking distance of each other (Wood et al. 1985).
Studies of Gainj and Kalam population structures have concentrated on how the overall magnitude of migration, how migration is affected by geographic distance and language differences, and whether these factors have had an impact on genetic variation. In terms of migration, Wood et al. (1985) found quite a difference in rates of parent–offspring migration between males and females. Among males, 84% were born in the same parish as their father, whereas among females, only 33% were born in the same parish as their mother. This sex difference is due to patrilocal postmarital residence, which means a married couple will tend to take up residence in the husband’s parish of birth, so that women are more likely to move. Even so, movement among both males and females tends to be highly restricted by geography. Among the actual migrants, the average distance from premarital to postmarital residence was about 8 km for males and 6 km for females. Far fewer migrations took place over long distances.
Wood et al. (1985) also looked at migration using a model that factored in geographic distance, linguistic differences (in terms of the percentage of Gainj and Kalam speakers in a parish), and population size. All of these factors had some effect on the rate of migrations between pairs of parishes. For both males and females, geographic distance and language differences had an effect, although in different ways. For males, language differences were more important in restricting migration than geographic distances, whereas for females the two factors had an equal influence. Differences in population size influenced migration rates in both sexes, with a tendency for individuals to move from larger populations to smaller populations more often than the reverse. This pattern might be a form of population size regulation in New Guinea, although this is not clear, as this effect of population size has been seen in a variety of human populations of different size and ecology (Relethford 1992).
Looking at genetic differences, Long (1986) found a value of FST = 0.0224 based on genetic marker data from the Gainj and Kalam parishes. This is a moderate level of genetic variation between groups given the fact that the parishes are not that far apart geographically and there is a high rate of migration outside one’s birth parish. Closer analysis suggested that the underlying population structure is more complex, where each parish is actually made up of a number of smaller breeding populations.
Long et al. (1987) and Smouse and Long (1992) focussed on the relationship of genetic distances between populations based on genetic markers and geography, language, and population size. They found that the smaller parishes were more genetically divergent from other parishes, which is expected given genetic drift. Geographic distance and language differences had little effect on genetic distances between populations. Although geography and language did affect levels of migration, these effects were not seen in the actual genetic distances. Instead, the only significant impact on the actual genetic distances was population size—smaller populations showed greater genetic drift. Smouse and Long (1992) argue that the relatively high rate of overall migration effectively “erases” previous population structure and the observed genetic differences reflect only the most recent effects of genetic drift. When we observe genetic differences between populations, we see a record of past demographic history, but sometimes, as is the case here, that history is relatively shallow.
An important thing to remember about studies of human population structure is that they are not necessarily representative of an entire geographic region of culture. Results can vary from one study to another depending on specifics of geography and culture. On one hand, the relatively low correlation of geography and language with genetics among the Gainj and Kalam have been found in other populations of highland New Guinea, so it could be argued to be typical of that region (Long et al. 1987). On the other hand, studies of villages on Bougainville Island, elsewhere in Melanesia, showed higher correlations of geography and language and genetics (Friedlaender 1975).
9.1.2 The Åland Islands
Another example of how migration and genetic data can be used to study human population structure comes from across the world in the Åland Islands, which are located between Sweden and Finland in northern Europe generations (Jorde et al. 1982; Mielke et al. 1976, 1982). The Åland Islands consist of over 6000 islands and skerries (rocky islands that are too small for inhabitation). Several centuries of migration data have been collected from archival sources that allow investigation of changes in expected population structure over a number of generations. In addition, a large amount of genetic marker data were collected between 1958 and 1970 representing three recent generations.
The overall picture seen in the Åland Island studies is one of change associated with the breakdown of isolation following cultural changes. These trends are clear on examination of migration data based on marriage records from 15 parishes. For example, Relethford and Mielke (1994) found that the average level of exogamy among the 15 parishes remained relatively stable from the mideighteenth century (9.0%) to the end of the nineteenth century (7.1%). During the most recent time period (1900–1949), however, the average exogamy rate increased dramatically to 14.7%. This change is likely related to a number of demographic and economic changes affecting availability of potential mates as well as improved transportation opportunities in the early twentieth century, such as the introduction of steamboat routes and an increase in privately owned motorboats.
Analysis of the marriage records from 1750 to 1949 showed that both geographic distance and population size had an effect on levels of migration between Åland Island parishes. As is typically the case for human populations (and many other species), migration rates declined with geographic distance; more individuals choose mates closer to home than from more distant parishes. Although geographic distance affected migration rates for all time periods studied, the rate of decline in migration with distance changed over time, such that more migration took place over longer distances in the twentieth century than in earlier times (Mielke et al. 1994). This change also reflects the changes resulting in greater mobility that took place in the early twentieth century. As people became more mobile and travelled farther, the exponential decline of migration with geographic distance became less acute, and the slope of distance decay leveled off.
The availability of data on martial migration patterns allowed Mielke et al. (1976, 1982) to use a migration matrix approach for predicting how gene flow and genetic drift might have changed over time. As expected, the predicted values of genetic similarity within and between populations fit the isolation by distance model, as expected given the effect of geographic distance on migration. The increase in migration over time, as well as an increase in population size, suggests that genetic drift declined and gene flow increased, resulting in reduced levels of genetic similarity between populations. Similar results were found using data on parent-offspring migration (Jorde et al. 1982).
Of course, the migration matrix approach can only tell us what we will expect to see at equilibrium between gene flow and genetic drift based on observed patterns of migration and population size. As noted in Chapter 8, the actual patterns of genetic variation might be different. For the Åland Islands, genetic marker data were available to examine how well the predictions from migration matrix analysis fit reality. Jorde et al. (1982) took data collected between 1958 and 1970 and collated it by each person’s year of birth, allowing them to sample three generations: (1) those born before 1900, (2) those born between 1900 and 1929, and (3) those born after 1929. They also examined genetic variation separately for the parishes on the main island as well as between parishes located in the more isolated outer islands. This strategy allowed Jorde and colleagues to look at changes in genetic variation over both time and space.
Using genetic markers, Jorde and colleagues found that FST was greater in the outer islands than on the main island, as expected given their greater isolation and smaller effective population size. However, there was little change in FST over time, unlike the expectations from migration matrix analysis. The same difference between migration and genetic data was found using isolation by distance analysis; the migration data showed a clear reduction in isolation over time, but this trend was less clear in the genetic data. The assumption of equilibrium used in migration matrix analysis may not appropriate, given the rapid demographic changes that took place in the Åland Islands during the twentieth century. Even so, further analysis showed that genetic variation was related to both population size and migration on attempts to separate the two effects (important because migration matrix analysis looks at both drift and gene flow at the same time). Here, Jorde et al. (1982) looked at the correlations between genetic distance and distances on the basis of a simple expectation of drift (based on population size differences) and a simple expectation of gene flow (based on migration rate differences). They found that drift had a greater effect in the earliest time period, but declined over time, and gene flow had a greater impact on genetic variation in the most recent time period. Again, this change is consistent with the observations of increasing migration rates (increasing the effect of gene flow) and increasing population size (reducing the effect of genetic drift).
The Aland Island studies comparing genetic and demographic data are important because they show us that some population genetic models, such as the migration matrix and isolation by distance models, may sometimes make assumptions that do not match up with the reality of rapid demographic and cultural change in human populations, particularly in more recent times. Still, careful examination of results can show us that genetic variation is shaped by gene flow and genetic drift, and the relative impact of these forces can change as well.
9.1.3 Altitude and Population Structure in Jujay, Argentina
A major (perhaps the major) influence on human population structure is geographic distance (e.g., Jorde 1980). As shown in the examples above and in Chapter 8, this makes sense, given the limiting effect that geographic distance has on migration. While this simple model is appealing, in many cases it may be too simple, as it ignores other influences on gene flow and population structure. The developing field of landscape genetics considers other ecological influences on gene flow and genetic variation, such as rivers and mountains (Storfer et al. 2007).
An example of an environmental influence on population structure is given here from a study of altitude and population structure in the Province of Jujay in northwestern Argentina (Gómez-Pérez et al. 2011). This study examined variation in a type of DNA marker known as an Alu insertion, which is a short section of DNA that replicates and moves to different locations on other chromosomes. Alleles are defined by the presence or absence of different insertion markers. Alu insertions are useful in tracking ancestry because the ancestral condition is the absence of an insertion, and any two individuals sharing the same Alu insertion marker would have inherited it from a common ancestor. Gómez-Pérez and colleagues looked at eight different Alu insertion markers in five regions of Jujay, sampling a wide range of altitude ranging from about 1200 meters (3937 ft) to over 3300 m (10,827 ft). The purpose of this study was to examine the impact of altitude on genetic diversity and genetic distance.
Gómez-Pérez et al. (2011) found that heterozygosity was lower in the high-altitude regions than in those at lower altitudes. Population density is lower in the high-altitude populations, contributing to increased genetic drift. In addition, the high-altitude populations have lower levels of exogamy, which means that there is less gene flow into the populations. The combined effect of increased genetic drift and decreased gene flow has resulted in lower levels of heterozygosity. In addition, the sources of gene flow also varied by altitude. The genetics of Jujay reflects a mix of Native American, European, and African ancestors, where European settlers and enslaved Africans entered the gene pool between the sixteenth and nineteenth centuries. Using the Alu insertion markers, Gómez-Pérez and colleagues found that Native American ancestry increased with altitude. Populations at the highest altitude had 100% Native American ancestry, whereas the lower-altitude populations showed less Native American ancestry but had significant European and African ancestry. Isolation by altitude appears to have affected levels of gene flow that entered the native populations over historic times. Immigrants tended to settle at lower altitudes, leading to more admixture.
Gómez-Pérez et al. (2011) also looked at the influence of altitude on genetic distances between the populations. They found a moderate and significant correlation between genetic distance and altitude difference; the farther apart two groups in terms of altitude, the greater the genetic distance between them. They also found a significant correlation with geographic distance, which was much stronger than the correlation with altitude. They conclude that the spatial distribution of Alu insertion markers in Jujay was best explained in terms of the isolation by distance model, further affected by differences in altitude. As with the studies of the Gainj/Kalam and the Åland Islands, the Jujay study shows the importance of using information on unique environmental, demographic, and cultural aspects of population structure to understand genetic variation.
9.2 The Origin of Modern Humans
The remaining case studies in this chapter focus on an examination of how the particular history of a population is reflected in patterns of genetic variation. A common aspect of the case studies is the use of genetic data to provide us with an understanding of population origins. Several different levels of analysis will be presented, starting with the most inclusive—the genetic history of our entire species. Additional case studies look at the origins of different populations around the world.
When most people think about the long-term evolution of our species from earlier human ancestors, they typically think of the fossil record. Although the fossil and archaeological records are an important source of information on human evolution, the last several decades have seen increasing attention to the role that genetic variation can play in understanding human origins. When we study the genetic variation within and between human populations in the world today, we can detect the genetic signature of many past events in our evolutionary history. Further, advances in technology have now allowed direct estimates of past genetic variation through the analysis of ancient DNA extracted from fossil remains tens of thousands of years old. The use of genetics in analyzing evolutionary events was described briefly in Chapter 4 in terms of the split between the African ape and human lines. Here, the topic of how genetics informs us about the origin of modern humans will be reviewed briefly.
A brief review of the major stages of human evolution is needed to focus on the origin of modern humans. Bipedal ancestors arose in Africa approximately 6 million years ago. Around 2 million years ago, some evolved into the genus Homo, characterized by an increase in brain size, a reduction in the size of the face and teeth, and an increased reliance on stone tool technology. One species, Homo erectus, was the first human ancestor to spread beyond Africa, migrating into parts of Southeast and East Asia, and to the easternmost fringes of Europe. Between 800,000 and 200,000 years ago, some populations of early humans evolved a brain size that was almost the same size as modern humans, but still had a relatively large face and a differently shaped skull. Many anthropologists place these finds in the species Homo heidelbergensis, which occupied parts of Europe, Africa, and perhaps Asia. Homo heidelbergensis appears to give rise to a group of early humans known as the Neandertals in parts of Europe and the Middle East.
Anatomically modern humans, with a large brain, rounded skull, reduced face, and a distinct chin, appear over the past 200,000 years. The distribution of fossils that can be classified as modern humans shows that they appeared first in Africa, with representative fossils found at 160,000 years ago (White et al. 2003) and 200,000 years ago (McDougall et al. 2005), well before the appearance of modern humans elsewhere. There appears to have been a brief expansion of modern humans into the Middle East at about 90,000 years ago, followed by a major dispersion around 60,000–70,000 years ago, with modern humans spreading into Australia, East Asia, and Europe.
These time frames indicate that modern humans appear first in Africa and, as they spread out throughout the rest of the world, they would have encountered earlier humans (such as the Neandertals) that were already there. This raises the question of possible gene flow between modern and other human populations. Anthropologists have proposed two models that focus on this initial African origin and the issue of gene flow outside of Africa. The first is the African replacement model, which proposes that modern humans completely replaced earlier humans without any interbreeding. Under this model, other humans such as the Neandertals became extinct without contributing anything to our ancestry. An alternative view, the assimilation model, proposes that modern humans interbred with other human populations outside of Africa. Here, groups such as the Neandertals would have contributed something to our ancestry even though as a distinct population they have become extinct (Smith et al. 2005; Relethford 2008a).
9.2.1 Genetic Consequences of an African Origin
For the moment, we will put aside the question of possible interbreeding and focus on the African origin of modern humans. From a genetic perspective, we are interested in seeing to what extent data on genetic variation in living humans are consistent with the African origin that we see from the fossil record, and how an African origin explains certain features of genetic variation in our species. Numerous studies of coalescent trees based on different DNA markers have shown that most (but not all) gene trees have roots in Africa, consistent with our African origin (Garrigan and Hammer 2006). In addition, Relethford (2011) lists five characteristics of genetic variation in living humans that relates in whole or in part to our African origin:
1. Low levels of genetic variation relative to other species
2. Higher levels of genetic variation in Africa
3. Genetic diversity declines with distance out of Africa
4. Low levels of genetic differentiation between geographic regions
5. A strong global correlation between genetics and geography
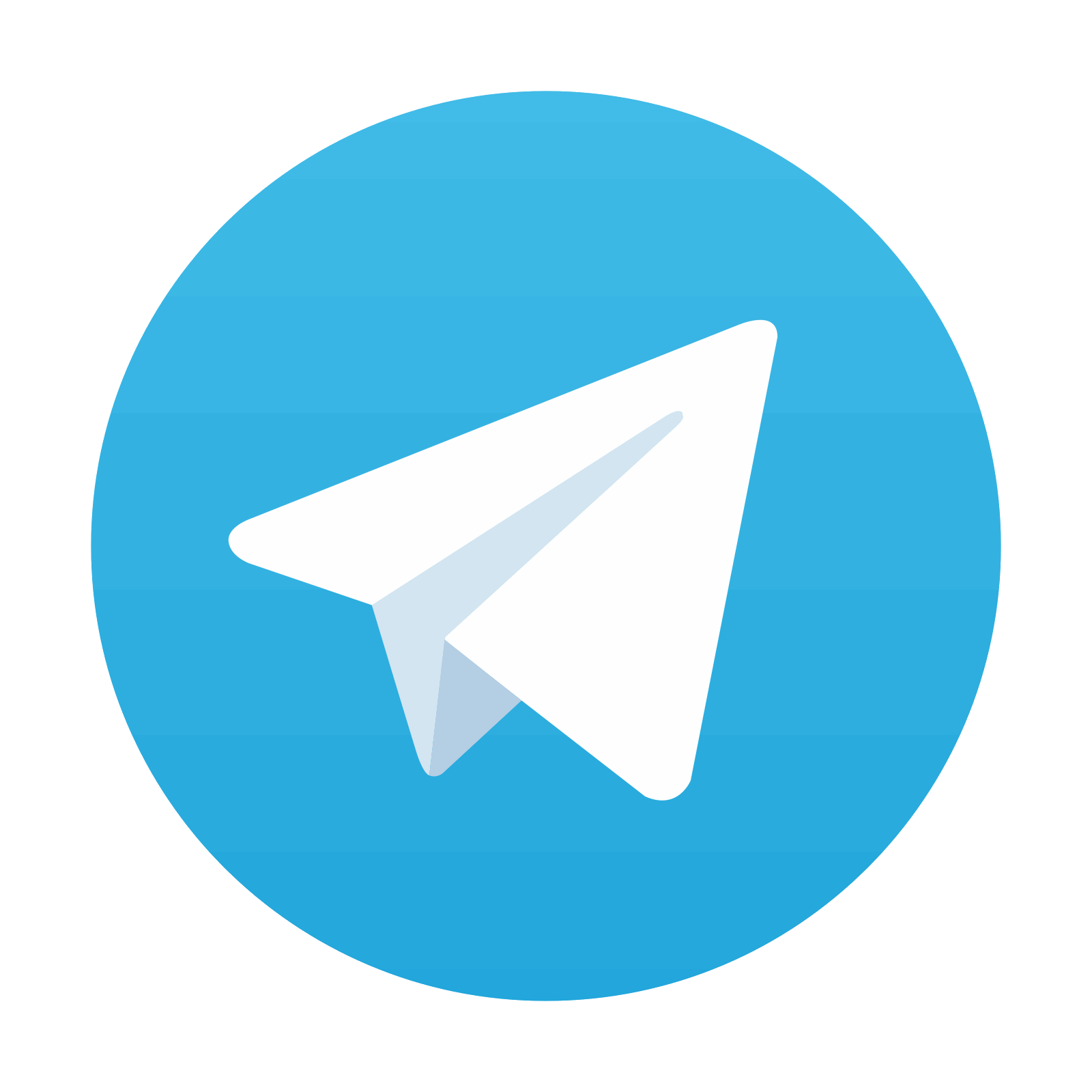
Stay updated, free articles. Join our Telegram channel

Full access? Get Clinical Tree
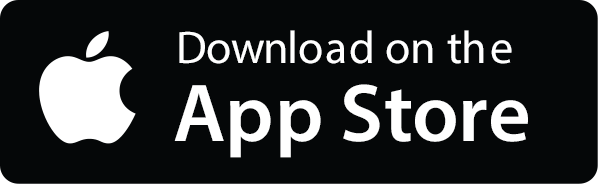
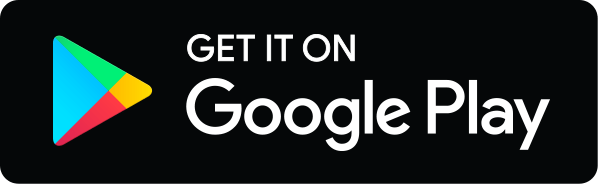