9 How histological stains work
Introduction
The key questions to bear in mind when seeking to understand histological stains are as follows:
A general theory of staining
Why are stains taken into the tissues?
Various contributions to stain-tissue affinity are outlined in Table 9.1, and are discussed below. Practical staining processes commonly involve several such factors.
Table 9.1 Factors contributing to dye-tissue affinities
Interactions | Practical examples where the factor is important |
---|---|
Reagent-tissue interactions | |
Acid and basic dyes, and other ionic reagents, including inorganic salts Most important with large molecules such as the elastic fiber stains, and final reaction products such as bisformazans in enzyme histochemistry Staining of glycogen by carminic acid, and collagen by Sirius red Methods such as the Feulgen nucleal, PAS, & mercury orange for thiols | |
Solvent-solvent interactions | |
The hydrophobic effect | Staining systems using aqueous solutions of dyes or other organic reagents; enzyme substrates for example |
Reagent-reagent interactions | Metachromatic staining with basic dyes, inorganic pigments in Gomori-type enzyme histochemistry, silver impregnation |
Reagent-tissue interactions
Coulombic attractions, which have also been termed salt links or electrostatic bonds, are widely discussed reagent-tissue interactions. These arise from electrostatic attractions of unlike ions, e.g. the colored cations of basic dyes and tissue structures rich in anions such as phosphated DNA, or sulfated mucosubstances (Lyon 1991; Prentø 2009). In practice, the amount of dye ion binding to a tissue substrate depends not only on the charge signs of dye and tissue but also on their magnitude, on the amount of non-dye electrolyte present in the dyebath, and on the ability of the tissue substrate to swell or shrink (Scott 1973; Bennion & Horobin 1974; Goldstein & Horobin 1974b; Horobin & Goldstein 1974).
Such phenomena are important for all ionic reagents, not just dyestuffs, an example being the periodate anions used as the oxidant in the periodic acid-Schiff procedure (Scott & Harbinson 1968). Even initially uncharged tissue substrates acquire ionic character after binding ionic reagents, e.g., glycogen staining by the PAS procedure and with Best’s carmine.
Consequently proteins rich in tyrosine and tryptophan residues, and nucleic acids with their heterocyclic bases, favor van der Waals’ attractions, as do the large aromatic systems of stains such as bisazo dyes and bistetrazolium salts, halogenated dyes (such as rose Bengal and phloxine), and enzyme substrates based on naphthyl and indoxyl with extended conjugation (Horobin & Bennion 1973). For instance, van der Waals’ attractions contribute substantially to stain-tissue affinity when staining elastic fibers – rich in aromatic desmosine and isodesmosine residues – with polyaromatic acid and basic dyes such as Congo red and orcein.
Hydrogen bonding is a dye-tissue attraction arising when a hydrogen atom lies between two electronegative atoms (e.g. oxygen or nitrogen), though it is covalently bonded only to one. Water is hydrogen bonded extensively to itself, forming the clusters important for the hydrophobic effect discussed below, and also to other molecules with hydrogen bonding groups, such as many dyes and tissue components. As there are many more molecules of water present than dye, hydrogen bonding is not usually important for stain-tissue affinity when aqueous solvents are used. An exception arises when hydrogen bonding is particularly favored by the substrate, as is the case with connective tissue fibers (Prentø 2007). In wholly or partially non-aqueous solutions, hydrogen bonding can also be significant, as with Best’s carmine stain for glycogen (Horobin & Murgatroyd 1970).
Covalent bonding between tissue and stain also occurs, which bonds may be regarded merely as another source of stain-tissue affinity. Practical reactive methods, e.g. the Feulgen nuclear and the periodic acid-Schiff procedures, are described elsewhere in this volume. The polar covalent bonds between metal ions and ‘mordant’ dyes are a special case. Dye-tissue binding due to such bonds has been termed mordanting, but is of uncertain status. The characteristic staining properties of mordant dyes may have other, or at least additional, causes. For instance, unlike most cationic dyes used as biological stains, cationic metal-complex dyes are usually markedly hydrophilic (Bettinger & Zimmermann 1991) and consequently resist extraction into alcoholic dehydration fluids (Marshall & Horobin 1973).
Solvent-solvent interactions
A major contribution to stain-tissue affinity when using organic reagents or dyes in aqueous solution is the hydrophobic effect. This is the tendency of hydrophobic groupings (such as leucine and valine side chains of proteins; or biphenyl and naphthyl groupings of enzyme substrates and dyes) to come together, even though initially dispersed in an aqueous environment. The process occurs because water is a highly structured liquid. Many water molecules are held together by hydrogen bonding (see above) in transient clusters, whose formation is favored by the presence of hydrophobic groups. Processes breaking clusters into individual water molecules occur spontaneously, because these events increase the entropy of the system. Consequently, removing cluster-stabilizing hydrophobic groups from contact with water, by placing them in contact with each other, is thermodynamically favored. For background on the hydrophobic effect see textbooks of biochemistry or chemical thermodynamics (Tanford 2004). The effect becomes more important as the substrate and reagent become more hydrophobic, as with staining of fats by Sudan dyes. When these hydrophobic dyes are applied from substantially aqueous solutions, the hydrophobic effect will be a major contribution to affinity. It should be noted that the hydrophobic effect is sometimes termed hydrophobic bonding, even though no special bonds are involved (only water-water hydrogen bonds and, sometimes, stain-tissue van der Waals’ attractions).
Stain-stain interactions
Dye-dye interactions can also contribute to affinity. Dye molecules tend to attract each other, forming aggregates. Even in dilute solutions, and especially in aqueous solutions where the hydrophobic effect is important, dimers or larger aggregates of dye ions are often present. van der Waals’ attractions (see above) between dye molecules will be important in both aqueous and non-aqueous solutions. Dye aggregation increases with concentration, e.g. when high dye concentrations build up on tissue sections. With basic (cationic) dyes this occurs on substrates of high negative charge density, e.g. sulfated polysaccharides in mast cell granules, a classic site for metachromatic staining by dyes such as toluidine blue. This phenomenon occurs because dye aggregates have spectral properties different from the monomeric dye. That dye-dye interactions contribute to affinity in tissue sections was demonstrated quantitatively by Goldstein (1962).
Other examples of stain-stain interactions contributing to affinity include metallic nano- and micro-crystals generated by gold or silver impregnation, metal sulfide precipitates formed in Gomori-type enzyme histochemistry, and the purple azure-eosin charge transfer complex produced during Romanowsky-Giemsa staining of cell nuclei (Horobin 2011).
Some unusual possibilities
Sometimes stains are taken into live creatures, in ways that reflect the biochemical composition and physiological activities of the living cell or organism. Traditionally termed vital staining or supravital staining, this is now usually described as the use of fluorescent probes. Over the past 20 years this methodology has undergone a renaissance; for a recent overview see Celis (2006).
Solubility, a related property
A little-discussed, but nevertheless important, property of stains is their solubility. For instance, when staining fat with Sudan dyes, an upper limit of staining intensity is set by the solubility of the dyes in the target substance, and is also influenced by the solubility in the staining bath solvent. Solubility is also involved in dye retention after staining: see below. The solubility of a staining reagent has complex causes. Put simply, the stronger the reagent-reagent interactions, the lower the solubility. For a general discussion of solubility see physicochemical texts such that of Letcher (2007).
Why is stain retained in tissue after its removal from the staining bath?
This contrasts with routine basic (cationic) dyes such as crystal violet or methylene blue, which freely and rapidly dissolve in the lower alcohols. Routine acid (anionic) dyes, such as eosin Y or orange G, are often less soluble in alcohols, as indeed are hydrophilic basic dyes with large aromatic systems, such as alcian blue. Non-ionic dyes such as Sudan fat stains are soluble in common dehydrating agents and clearing solvents, and in resin mountants. Note: structures of exemplar hydrophilic and lipophilic basic dyes are shown in Figure 9.1.
Why are the stains not taken up into every part of the tissue?
Numbers and affinities of binding sites
Practical staining conditions maximize selective affinities. Basic dyes are applied from neutral or acidic solutions, since under alkaline conditions proteins carry an overall negative charge and so may also bind basic dyes. Affinities are also influenced by varying the concentration of inorganic salt present. The various aluminum-hematoxylin, for instance, differ substantially in this regard. The critical electrolyte concentration methodology (Scott 1973) and several other empirical procedures are based on control of electrolyte content. However, staining that distinguishes two structures is still possible even when stain-tissue affinities and the number of stain-binding sites are the same. This is because rate of reagent uptake, or rate of subsequent reaction, or rate of loss of reagent or product, may not be the same in the two structures.
Rates of reagent uptake
Progressive dyeing methods may be rate controlled, for instance mucin staining using alcian blue or colloidal iron. Selectivity requires short periods of dyeing during which only fast-staining mucins acquire color (Goldstein 1962, Goldstein & Horobin 1974a). If staining is prolonged, additional basophilic materials such as nuclei and RNA-rich cytoplasms can also stain. Dyes used in this way are often large in size, and hence diffuse slowly, maximizing the control possible via differential rate effects.
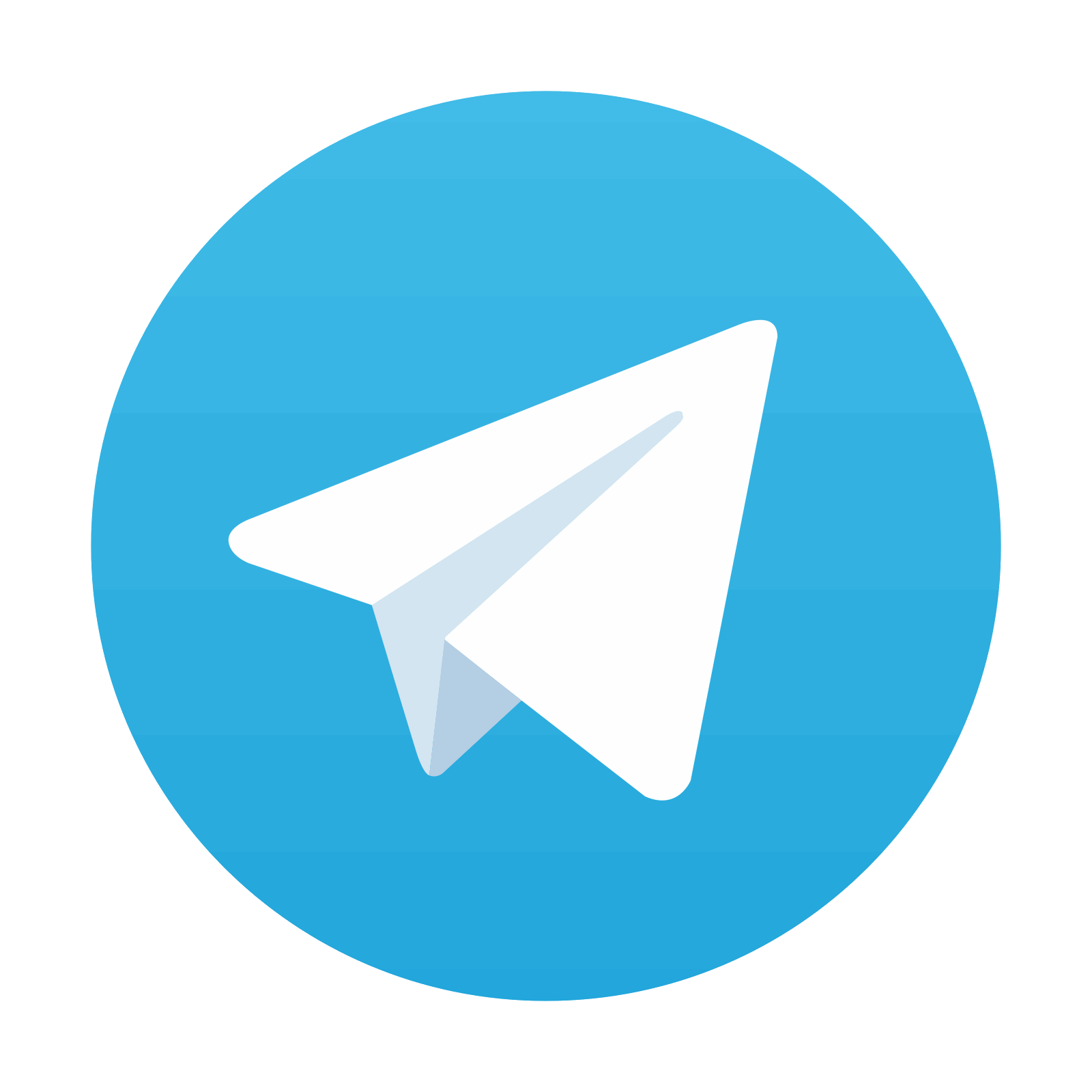
Stay updated, free articles. Join our Telegram channel

Full access? Get Clinical Tree
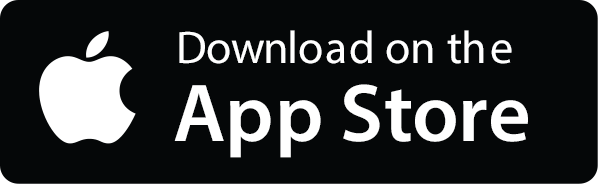
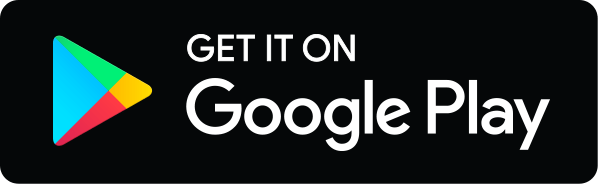