Gary V. Zoccolante
CONTENTS
Validation, Verification, and Qualification
Foreign Pharmacopeial Requirements
Determine System Capacity Requirements
Determining the Optimum Generation System
Pharmaceutical Water System Design
USP Purified Water and Water for Injection Generation Systems
Storage and Distribution Systems
Distribution Storage Tank Design Considerations
Distribution Piping Design Considerations
Continuous Recirculating or Nonrecirculating Configuration
Distribution System Polishing Components
Trends and Future Developments
INTRODUCTION
The importance of process water to a pharmaceutical manufacturing facility cannot be overstated. Production of water used for drug manufacturing is a great challenge in every aspect of design, implementation, and maintenance. Water is the most widely used material in pharmaceutical manufacturing and is often the most costly. The percentage of water in finished products varies from zero to greater than 90%. A greater volume of water is used in cleaning and rinsing processes than in formulation in most facilities. Regardless of the water volume used in the actual drug, formulation of all pharmaceutical water is subject to current Good Manufacturing Practices (cGMPs) even when the water does not remain in the finished product.
Water treatment systems are often investigated in great depth by U.S. Department of Health and Human Services Food and Drug Administration (FDA) inspectors. Poor design and inadequate maintenance of water systems have led to countless FDA 483 citations, warning letters, and in certain cases, recalls of pharmaceutical products.
Optimization of pharmaceutical water systems is a risk management exercise that requires extensive utilization of good engineering practice (GEP). The design team must make decisions regarding water quality, method of generation and distribution, sanitization method, instrumentation and control, data acquisition, and other design details. Construction and maintenance specifications are all based on the impact of the consequences of water system success or failure. Optimization is a delicate balance of acceptable risk and available financial resources. Pharmaceutical companies are under intense pressure to reduce costs while maintaining maximum product safety with minimal patient risk. Competence in pharmaceutical water requires knowledge of pharmacopeial requirements, GMPs, GEP, chemistry, microbial control, sanitization strategies, many unit processes, generation options, storage and distribution options, and commissioning and qualification requirements.
This chapter provides readers with information in all of these areas that can be utilized in conjunction with the recommended related reading to be able to produce a conceptual design for a pharmaceutical water system. Practical options are provided for the generation and distribution of pharmaceutical water so that the reader can know what has been done in existing facilities. Advantages and disadvantages are presented to aid in the selection of desirable configurations.
EXECUTIVE SUMMARY
Sound water system design requires knowledge of both external and internal quality requirements. External requirements are set by pharmacopeial groups worldwide. Internal requirements are typically set by quality groups and often exceed external requirements. Pharmacopeial groups set the therapeutic drug standards for a country or region, as well as standards for drug ingredients. Water standards are set by these pharmacopeial groups. Required water quality and methods of manufacture often vary with different regulatory groups. The required water quality is determined by use and product destination. Use can be for product manufacturing, active ingredient production, cleaning, medical device manufacturing, ophthalmics, topicals, consumer products, and many other applications. The water design team must properly specify the correct water quality. The products and product destinations must be defined to understand what regulatory requirements must be met. Parenteral products require a minimum of water for injection (WFI) quality. Oral dosages require a minimum of purified water (PW) quality.
Inspection agencies such as the U.S. FDA work in conjunction with pharmacopeial groups to enforce regulations and set requirements for GMPs. GMPs are rarely specific regarding water and must be interpreted to be properly applied. Groups such as the FDA are likely to find more issues with water system GMP compliance than with water quality compliance.
All water systems must comply with GMPs, and system design is a risk assessment exercise. The variation in system design and cost is extreme, as risk aversion and company standards vary significantly. Pharmaceutical companies assess many factors, including product dosage form, water use, destination, and product name recognition. Large cost discrepancies occur from differences in materials of construction, instruments and control, sanitization methods, documentation, testing, and many other factors.
System maintenance is critical to proper operation. Regulatory agencies frequently monitor maintenance, standard operating procedures (SOPs), operator training, record keeping, and other related factors. In many cases, soundly designed systems have been cited by regulators for improper maintenance and poor operator compliance with SOPs.
Systems usually comprise generation equipment and storage and distribution equipment. Water quality requirements must be met at both locations, and proper sanitization of both system segments is critical. Sanitization of generation equipment is either chemical or thermal, and distribution sanitization is chemical, heat, or ozone. The sanitizer choice impacts cost, microbial control effectiveness, and system uptime availability. Selection of sanitization methods for generation and distribution is one of the most important decisions that water system designers must make. The decisions significantly impact microbial control, risk, and costs. Both capital cost and operating cost are affected.
Pharmaceutical companies have historically produced WFI by distillation. Distillation has historically been a required method in all countries complying with the European Pharmacopoeia. Recently the European Pharmacopoeia Commission posted a press release regarding adoption of a revision of its monograph for Water for Injections (0169). “Up to now, the production of Water for Injections (WFI) had been limited to distillation only. The revision allows for production of WFI by a purification process equivalent to distillation such as reverse osmosis, coupled with appropriate techniques. The revised monograph will be published in the Ph. Eur. Supplement 9.1 and will become effective in April 2017.” This revision aligns the EP WFI requirements closely with the USP and JP WFI requirements and will likely increase the implementation of robust membrane based alternative systems in the future. Distillation will certainly remain in operation in many facilities and be implemented in many new facilities, but the overwhelming domination of distillation for WFI will likely change significantly. Many other locales, including the United States, allow alternative methods of production, but if a product is manufactured for worldwide distribution, distillation must be used until the EP WFI revision becomes effective in April 2017. PW can be produced by any method. Chemically regenerated ion exchange (IX) units dominated PW production decades ago, as membrane-based production was new and unproven. Membrane-based production is dominant now, as most companies try to minimize chemical discharge. Chemical discharge is also eliminated with use of off-site regenerated IX systems.
GOOD MANUFACTURING PRACTICES
One of the most significant issues in water system design and operation is that although the GMP requirements are well documented in writing, they are very general and subject to continually tightening interpretation as cGMPs. The FDA establishes cGMP requirements beyond those that are documented in legal compendia, but rarely publishes written guidelines with any level of detailed engineering guidance.
Most of the GMP requirements for water are derived from broad statements in 21 CFR 211. These general statements relate to the requirement for water used in production or cleaning processes to not “alter the safety, identity, strength, quality or purity of the drug product.” The statements directly open all water system unit operations, contact surfaces of equipment and piping, installation, and maintenance to FDA scrutiny. All materials must be proven to be compatible with the product and process and must not contribute objectionable contaminants.
Additional 21 CFR 211 GMP requirements for verification of proper cleaning and sanitization procedures mandate written records and procedures for these steps. All rinse and cleaning water qualities must be proven to be appropriate.
Most of the engineering details that are considered to be cGMP requirements have evolved over decades of system development since the birth of the concept of GMP manufacturing. Several key concepts of cGMP production of water have been adopted from the long considered, but never adopted “Good Manufacturing Practices for Large Volume Parenterals,” 21 CFR 212. This legislation was proposed in 1976 and finally removed from consideration in 1994. Although the “GMPs for LVPs” document was never approved, many concepts proposed in the document have become commonplace in pharmaceutical systems. Some of these concepts include storage tank vent filters, minimal piping dead legs, sloped and fully drainable distribution systems, flushed pump seals, double-tube sheet heat exchangers, and elimination of use point filters. These concepts and others will be discussed in more detail in pharmaceutical water system design section.
Due to the perceived ambiguity of cGMP regulations, great disparity exists in both individual and corporate views regarding what constitutes a cGMP-compliant water system. System costs may vary by more than an order of magnitude from company to company, with all groups believing that each system is optimized for cGMP construction and good design practice. The proper materials of construction, surface finishes, level and accuracy of instrumentation, automation level, data acquisition and trending, sanitization methods, system and component draining, use of microbially retentive filters, and many other factors are open to interpretation. The decisions made by the design team in these areas of design and construction have great impact in capital cost, operating costs, and risk management. The team may solicit input from consultants’ vendors and construction contractors to help in completion of the design specifications. Significant capital and operating cost savings are available to those who properly interpret the cGMP requirements and do not overdesign the system.
PHARMACOPEIA GROUPS
It is important to understand the roles of the FDA and the U.S. Pharmacopeial Convention (USPC). The USPC is a private not-for-profit organization established to promote public health. The USPC works closely with the FDA and the pharmaceutical industry to establish authoritative drug standards. These standards are enforceable by the FDA. More than 4,700 standards monographs are published in the United States Pharmacopeia (USP) and National Formulary (NF). The monographs for water used in pharmaceutical manufacturing for products used in the United States are published in the USP.
Other pharmacopeial regulations, such as the requirements of the European Pharmacopoeia (PhEur), the Society of Japanese Pharmacopoeia (JP), and the Chinese Pharmacopoeia (CP), may need to be considered in the water system design and water quality testing for products that are exported from the United States. The ultimate destination of drug products or drug substances determines the regulatory requirements that must be satisfied.
WATER QUALITY REQUIREMENTS
The types of water defined in the pharmacopeial monographs, such as PW and WFI, are known as compendial waters. Other quality waters used in manufacturing, not defined by USP or other recognized compendia, are known as noncompendial waters. Noncompendial waters can be used in many applications, such as production of many active pharmaceutical ingredients (APIs) and in many cleaning and rinsing steps.
Noncompendial waters are not necessarily lower quality than compendial waters. Noncompendial waters range from water that is only required to meet the U.S. Environmental Protection Agency (EPA) National Primary Drinking Water Requirements (NPDWRs) to water that is specified to exceed the requirements for WFI. Noncompendial water systems are not necessarily less tested, maintained, or validated than compendial waters, and are subject to the same cGMP requirements.
The water quality specification required for manufacturing is a function of several factors. Where will the product be shipped? If production is for the United States only, the water specification will be principally based on USP requirements. Shipment to Europe will require compliance with PhEur requirements, and shipment to China will require compliance with CP requirements. Many other countries utilize USP, PhEur, CP, or JP regulations or have their own requirements. In addition to pharmacopeial requirements, water specifications reflect product and process requirements and corporate views toward FDA and cGMP regulations.
Microbial control methods for water systems frequently impact the total cost of water production more than attainment of the chemical attributes of water outlined in USP and other appropriate compendia. The chemical attributes of compendial water listed in the monographs of the governing pharmacopeial groups are generally easily met with a properly designed and maintained system.
The microbial requirements are not stated in the USP monographs, as of this writing, but the maximum action levels are documented in the USP 38 general information chapter <1231> and have been defined by the FDA in the 1993 FDA “Guide for Inspections of High-Purity Water Systems.” Although the chemical quality of water must be met consistently at points of use, proper microbial control is the focus of many FDA inspections.
SAMPLING
A sampling and testing plan must be developed for every pharmaceutical water system. This is a cGMP requirement, certainly GEP, and necessary for monitoring system operation and control. It is important to design sampling points into the unit processes to be able to monitor each process for validation, normal operation, and troubleshooting. Test protocols and frequency must be established for each unit process, as well as every use point.
Samples for quality control purposes, as opposed to process control purposes, must be collected in an appropriate manner. As an example, use point samples for hose connections must be collected from actual production hoses using the same flush cycle used in production to prove proper water quality. Regulators do not require sample collection to be done in an unsafe manner, but expect sampling to be done as close to the use point as practical.
Unit process tests should be based on the expected unit performance (e.g., effluent chlorine level for an activated carbon unit employed for dechlorination). Use point testing must be sufficient to prove compliance with both chemical and microbial requirements. Most of the chemical requirements may be proven with online or laboratory conductivity and total organic carbon (TOC) monitoring from a single distribution system sample location. Periodic use point testing is required to verify the single-loop sample location.
A single distribution loop sample for microbial performance is not acceptable. Each use point must be tested at a sufficient frequency to prove that the system is in microbial control. The 1993 FDA “Guide for Inspections of High-Purity Water Systems” suggests microbial testing for a minimum of at least one use point per day, and that all use points are tested at least once weekly. Several recent industry guides have suggested that use point microbial tests can be conducted at reduced frequencies relative to the FDA guide text.
VALIDATION, VERIFICATION, AND QUALIFICATION
It is accepted that all pharmaceutical water systems will be validated or qualified. Validation was the term used for decades for the completion of test and inspection protocols to prove that the system was appropriate for the intended purpose. Other terms, such as verification and qualification, are also used today for this process. Verification and qualification are often used in risk-based qualification of systems. Validation is used for the purpose of the following discussion. The validation plan must be completed to some degree prior to specification of the water system. All equipment suppliers, contractors, commissioning agents, and other implementation parties must be aware of the requirements for documentation, automation life cycle, commissioning and validation overlap, and many other factors to ensure a successful validation. Critical information, such as proper life cycle methodology, instrument certifications, material certifications, weld documentation, and so forth, often cannot be created after the fact if the requirements were not known prior to manufacturing and installation. The most successful validations generally occur when the validation group has been involved throughout the project design phase.
Many groups, including the FDA, International Society for Pharmaceutical Engineering (ISPE), American Society for Testing and Materials (ASTM), and International Conference for Harmonisation (ICH), have promoted a change to a risk-based qualification process. The process of system qualification may be referred to as validation, qualification, or verification, dependent upon company preference and process. The ISPE Baseline Pharmaceutical Engineering Guide, Volume 5, Commissioning and Qualification, provides a practical approach to traditional system qualification. The ISPE Good Practice Guide: Approaches to Commissioning and Qualification of Pharmaceutical Water and Steam Systems and ASTM E2500 provide guidance on risk-based qualification. See Chapter 7 for further discussion.
RELATED READING
The ISPE Baseline Guide Water and Steam Systems (Volume 4, Second Edition) provides an excellent overview of all aspects of pharmaceutical water. ISPE Approaches to Commissioning and Qualification of Pharmaceutical Water and Steam Systems provides insight into risk-based and traditional qualification methods. The 1993 FDA “Guide to Inspections of High-Purity Water Systems” provides readers with insight into areas that inspectors may pursue.
MONOGRAPH REQUIREMENTS
USP 38 (as of this writing) includes monographs for seven types of pharmaceutical water. Three types of bulk water are defined, as well as five types of packaged waters. The three bulk waters are USP purified water (PW), USP water for injection (WFI), and USP water for hemodialysis. The packaged waters are bacteriostatic WFI, sterile water for inhalation, sterile WFI, sterile water for irrigation, and sterile PW.
Most pharmaceutical products are manufactured with either PW or WFI. PW and WFI have the same chemical purity requirements. The monographs require that the water purity is proven by conductivity and TOC. The conductivity requirement using USP <645> can be met with online testing (Stage 1) or in laboratory testing (Stages 1, 2, or 3). The Stage 1 conductivity test requires measurement of conductivity and water temperature. The conductivity limit varies from 0.6 μS/ cm at 0°C to 3.1 μS/cm at 100°C. Intermediate values include 1.3 μS/cm at 25°C and 2.7 μS/cm at 80°C.
Stage 1 conductivity requirements can be reliably attained with a variety of system configurations using common water purification processes. Most pharmaceutical water systems are designed to meet Stage 1 conductivity to take advantage of online testing to provide significant data for trending and minimize laboratory testing. Point-of-use testing generally requires laboratory analysis. Pharmaceutical water that does not meet the Stage 1 conductivity limit can be laboratory tested to meet the Stage 2 or 3 limits.
The TOC test is a limit response test with a theoretical limit of 500 ppb. The test is designed to accommodate virtually any TOC analyzer that meets the USP suitability requirements. Most manufacturers go beyond the pass–fail 500 ppb response test and record and trend values well below the 500 ppb limit. The FDA has been promoting process analytical technology (PAT) for pharmaceutical manufacturing. PAT requires online or at-line instrumentation with recording and trending of critical aspects of systems. Alert and action levels are much closer to normal operating levels than prior periods and well below specification limits. Online TOC and conductivity testing are encouraged with PAT thinking.
The microbial limits for USP PW are not defined in the legally binding monograph. The general information chapter <1231>, “Water for Pharmaceutical Purposes,” states that a maximum of 100 colony-forming units (cfu) per milliliter may be used as an action level, and this is also stated in the 1993 FDA “Guide to Inspections of High-Purity Water Systems.” The requirements of this general information section are not legally binding, but the FDA has stated publicly on many occasions that this is the maximum level acceptable for USP PW. The actual action level may be much lower than the maximum action level of 100 cfu per ml and is determined by the manufacturer (subject to FDA approval) as a function of product, process, and system performance. Some products and processes require an absence of certain objectionable species, such as Pseudomonas aeruginosa, as well as a low total viable plate count.
WFI has the same chemical requirements as PW and has a limit of 0.25 endotoxin units (EU) per milliliter. The microbial level for WFI is also absent from the monograph but is stated to be a maximum action level of 10 cfu/100 ml in USP Chapter <1231>. This is in agreement with FDA views.
The USP 38 PW monograph states, “Purified water is water obtained by a suitable process.” This essentially leaves the process selection open to all technologies. The USP 38 WFI monograph states, “Water for injection is water purified by distillation or a purification process that is equivalent or superior to distillation in the removal of chemicals and microorganisms.” Several prior volumes of USP limited WFI production to distillation or reverse osmosis (RO).
Distillation currently produces more than 99% of USP WFI. Other processes, such as a combination of RO, deionization (DI), and ultrafiltration, have a significant history of production of WFI quality water for rinsing, API production, and other uses as well as WFI where allowed.
WATER QUALITY SELECTION
The water quality or qualities selected for the pharmaceutical process must be consistent with the final product requirements. The final rinse water must be the same quality as the water used in manufacturing. Oral products must use a minimum of USP PW for manufacturing, and PW is normally used as final rinse water. Since the method of manufacture for PW is not stated by USP, there is little advantage to use of noncompendial water for final rinse water where PW is acceptable.
Parenteral products must use a minimum water quality of USP WFI for manufacturing, and WFI is used in most plants for final rinse water. It is acceptable to use WFI quality noncompendial water for final rinse in parenteral processes if practical. Production of noncompendial WFI quality water may or may not be less expensive than WFI.
The ISPE Baseline Guide Water and Steam Systems recommendations are shown in the water quality decision tree in Figure 6.1. Expanded views for laboratory, manufacturing, and cleaning are also shown (Figure 6.2).
The water quality requirements for API and bulk pharmaceutical chemicals (BPCs) are complex. The minimum water permitted in API or BPC manufacturing is water meeting the U.S. EPA NPDWRs or equivalent. APIs use a wide range of waters for manufacturing, initial rinses, and final rinses up to and including WFI. The ISPE Baseline Guide Water and Steam Systems water quality recommendations for API manufacturing are shown in Figures 6.3 and 6.4.
The FDA may expect WFI to be used in certain inhalation products depending on use. Water quality exceeding USP PW or WFI requirements may be required for some products, such as intrathecals. A large-volume parenteral product may have to be produced with water with endotoxin limits well below WFI limits, dependent upon the expected patient weight and dosage volume. The manufacturer is required to determine the appropriate water quality.
FIGURE 6.1 Laboratory water.
FIGURE 6.2 Water for manufacture.
FIGURE 6.3 API process water decision tree—minimum water quality.
FIGURE 6.4 API cleaning water decision tree.
FOREIGN PHARMACOPEIAL REQUIREMENTS
European Pharmacopoeia 8 has monographs for PW and WFI, as well as a third bulk water, highly PW. The EP 8 PW requirements are similar in many respects to USP 38 PW as of this writing. The chemical purity is defined by TOC and conductivity, but also by a traditional pass–fail test for nitrates.
EP 8 requires WFI to be produced by distillation without exception until April 2017 when the new language that is similar to USP WFI language becomes effective. The chemical requirements are the same as for EP 8 PW, with the exception that the conductivity limit at 20°C is 1.1 μS/cm. The microbial requirements are the same as for USP WFI. The EP 8 endotoxin requirements are the same as those in USP, although the units are expressed as IU/ml rather than EU/ml. The Japanese Pharmacopoeia allows for membrane-based WFI systems. The Chinese Pharmacopoeia is similar to EP 8 in requiring distillation for WFI production.
DESIGN AND COST FACTORS
The capital and operating costs for pharmaceutical water systems can vary significantly as a function of the processes and materials of construction selected. WFI systems has fewer acceptable options for generation, storage, and distribution than PW systems. The microbiological requirements are much tighter for WFI than for PW, and WFI is generally utilized for the most critical pharmaceutical applications. Most WFI systems utilize distillation, are similar in construction, and tend to favor conservative approaches to system design, as detailed in this chapter.
The selection of an appropriate sanitization method for generation, storage, and distribution equipment can impact capital and operating costs significantly. Thermally sanitizable systems generally have higher capital costs due to a greater content of stainless steel components but usually require considerably less labor for sanitization and have less downtime. Thermal sanitization is easier to automate and validate and typically allows attainment of lower microbial levels.
Chemically sanitized equipment has been proven to be acceptable in many applications and may have a lower capital cost, but generally requires more labor to prepare chemicals, verify attainment of proper chemical level during sanitization, and prove proper removal of residual chemical in rinse steps. Implementation of chemical sanitization is not driven by superior performance, but rather by capital cost. Hot water sanitizable equipment is generally higher in capital cost, but lower in operating cost, as sanitization is typically automated where chemical sanitization is labor-intensive.
Future needs and system expansion should be considered at the time of system design. Some unit processes may be practically expanded with a reasonable capital investment, while others are extremely difficult to expand without additional space, equipment, and controls. RO units that are designed for expansion may have increased capacity within the original dimensions through addition of pressure vessels and membranes. Column-based processes such as softeners and activated carbon units are generally impractical to expand without additional unit implementation. Low-cost processes such as softeners are often best oversized initially to allow for anticipated expanded flows in the future. Expansion may be practical from a mechanical perspective, but it may not be practical when production and qualification are considered. Downtime for expansion work and qualification must be considered.
DETERMINE SYSTEM CAPACITY REQUIREMENTS
One of the most critical and difficult steps in the programming of a water system is determination of the optimum generation and storage and distribution system sizing. Optimization requires accurate information regarding individual use point demand and the total manufacturing cycle. Users must provide data regarding flow, pressure, and temperature for each use point over a daily and weekly schedule. At times, this information is estimated prior to confirmation of the production cycle. The water usage chart in Figure 6.5 is an example of projected water consumption on an hourly basis. These data can be used in conjunction with a generation production rate, tank size, and tank makeup levels, as shown in the storage-tank-level chart in Figure 6.6. This exercise predicts tank levels throughout the day to project sufficient or insufficient levels over the operating day. If an insufficient tank level is indicated, changes must be made. Generation output can be increased, tank volume can be increased, or peak draw volumes can be reduced.
All parties involved must resist the tendency to overestimate consumption, or the system may be significantly oversized. Significant system oversizing wastes capital, can lead to microbial issues during operation, and can needlessly increase wastewater generation. Future needs should be considered during system design. Systems can often be designed to run at low flows initially and to be operated at higher flows later, as production needs increase. GEP minimizes capital expenditure without incurring unacceptable risk.
DETERMINING THE OPTIMUM GENERATION SYSTEM
Good design practice can be applied in the selection of the pharmaceutical water generation system process and equipment specification. Generation system selection should be based on accurate source water information, proper water quality specifications, life cycle cost analysis, sanitization methods, reliability, maintenance requirements, and several other possible factors.
FIGURE 6.5 Water usage chart.
FIGURE 6.6 Tank-level chart.
PHARMACEUTICAL WATER SYSTEM DESIGN
Pharmaceutical facilities may utilize a single grade or multiple grades of water. The water requirements may include the compendial grades of USP WFI or PW or various noncompendial grades. The first decision to make is whether a single grade of water is the best regulatory and economic choice, or multiple grades provide more logical operation. A higher grade of water, such as USP WFI, can also serve as a lower grade, such as USP PW. USP PW, of course, cannot be used as USP WFI. The cost to produce USP WFI may be higher than the cost to produce USP PW, so significant analysis is usually required to optimize system design.
Consider a facility that requires both USP WFI and USP PW. The facility could be best served by production of WFI only to serve both WFI and PW if several factors exist. If the WFI quantity required significantly exceeds the PW requirement, if all or most of the water is used hot (>65°C), and if the WFI and PW use are reasonably congruent, a single WFI system with hot storage is probably the best choice. If the PW requirement is greater than the WFI requirement, the PW is used at ambient temperature, the WFI and PW use points are reasonably divergent, heating and cooling resources are limited or expensive, and separate systems to produce and distribute WFI and PW are probably more logical.
After the choice of single or multiple water systems is made, the systems must be optimized for generation method and storage and distribution method chosen. Generation systems will generally comprise several of the pretreatment, final treatment, and polishing components discussed later in this chapter.
USP PURIFIED WATER AND WATER FOR INJECTION GENERATION SYSTEMS
Proper design of USP water systems requires consideration of many factors. Major factors include USP specifications, cGMP requirements, feed water quality, required system availability, raw water cost, plant wastewater discharge limits and costs, labor availability, outside service availability and competence, chemical handling, utility availability, and cost and designs with prior successful history. Previously successful system designs should always be weighed against other viable options unless the prior system design is obsolete or not cGMP.
The ISPE Baseline Water and Steam Guide Committee, after meetings with FDA personnel, determined that the specified water quality for pharmaceutical use must be met at the outlet of the generation system, as well as at the use points. Although some water quality parameters (particularly microbial levels in hot or ozonated storage systems) may improve in storage, the water quality should not fail as generated and depend on improvement in storage to comply with the quality specifications.
System configurations based on RO, IX, and distillation will be reviewed. Each of these systems is discussed in detail later in this chapter. Distillation-based systems have an extensive history of production of both USP WFI and PW. The alternate designs have been primarily utilized for PW production, with a few WFI applications. All designs will be assessed for the capacity to produce both compendial waters.
Most high-volume USP PW systems utilize RO as the primary purification process, with varying additional polishing processes. A technology map shows the most common options for the basic RO-based USP PW systems (Figure 6.7). The number of process steps implemented is usually a function of feed water quality, finished water quality specification, and risk assessment. The addition of an appropriate final endotoxin and microbial reduction process allows production of WFI quality water if the process is proven to be equal or superior to distillation.
The first pretreatment purification step is primary filtration for reduction of coarse suspended solids. Multimedia filtration is selected when labor must be minimized or the expected suspended solids level is low. Disposable cartridge or bag filters minimize capital cost and are a good choice for low suspended solids or low-flow applications.
FIGURE 6.7 Reverse osmosis technology map. (Courtesy of Evoqua Water Technologies LCC, Warrendale, PA.)
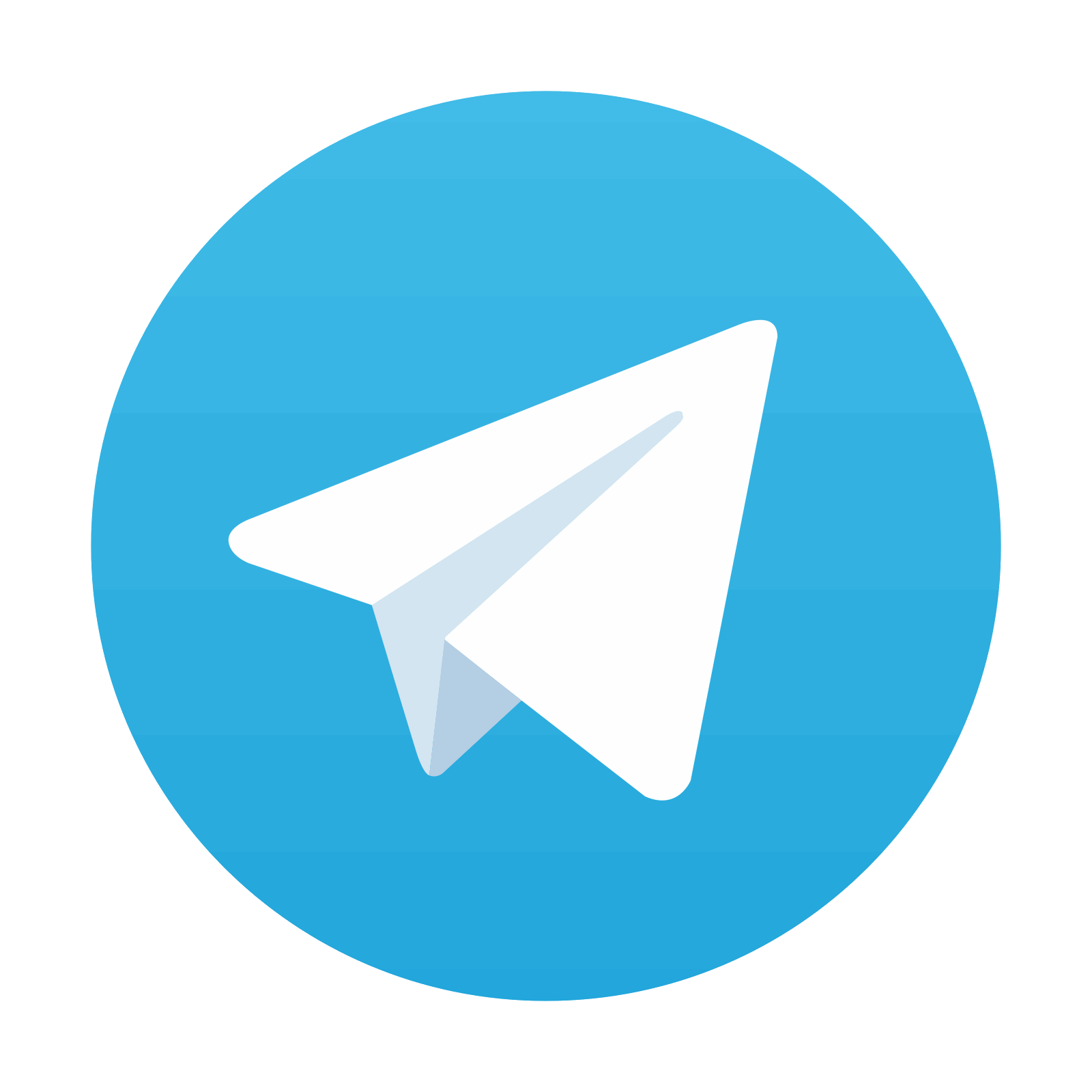
Stay updated, free articles. Join our Telegram channel

Full access? Get Clinical Tree
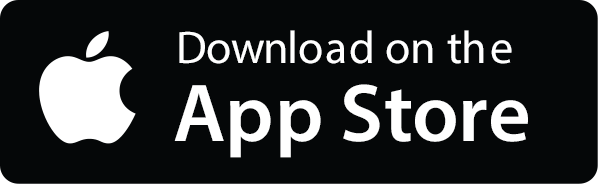
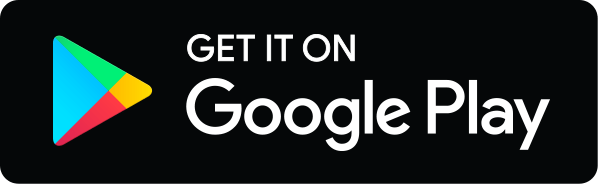