6 Robert C. James and Stephen M. Roberts The liver is one of the most important target organs for drug and chemical toxicity. The potential for hepatoxicity is often the principal concern in setting occupational and environmental standards for chemical exposure, and the discovery of hepatotoxicity is the most common cause for a drug to be removed from the market. This chapter will familiarize the reader with The liver is the largest organ in the body, accounting for about 5% of the total body mass. It is often the target organ of chemical-induced tissue injury, a fact recognized for over 100 years. While the chemicals toxic to the liver and the mechanisms of their toxicity are numerous and varied, several basic factors underlie the liver’s susceptibility to chemical attack. First, the liver maintains a unique position within the circulatory system. As Figure 6.1 shows, the liver effectively “filters” the blood coming from the gastrointestinal tract and abdominal space before this blood is pumped through the lungs and into the general circulation. This unique position in the circulatory system aids the liver in its normal functions, which include (i) carbohydrate storage and metabolism; (ii) metabolism of hormones, endogenous wastes, and foreign chemicals; (iii) synthesis of blood proteins; (iv) urea formation; (v) metabolism of fats; and (vi) bile formation. When drugs or chemicals are absorbed from the gastrointestinal tract, virtually all of the absorbed dose must pass through the liver before being distributed through the bloodstream to the rest of the body. Once a chemical reaches the general circulation, regardless of the route of absorption, it is still subject to extraction and metabolism by the liver. The liver receives nearly 30% of cardiac output and, at any given time, 10–15% of total blood volume is present in the liver. Consequently, it is difficult for any drug or chemical to escape contact with the liver, an important factor in the role of the liver in removing foreign chemicals. Figure 6.1 The liver maintains a unique position within the circulatory system. The liver’s prominence causes it to have increased vulnerability to toxic attack. The liver can particularly affect, or be affected by, chemicals ingested orally or administered intraperitoneally (i.e., into the abdominal cavity) because it is the first organ perfused by blood containing the chemical. As discussed in Chapter 2, rapid and extensive removal of the chemical by the liver can drastically reduce the amount of drug reaching the general circulation—termed the first-pass effect. Being the first organ encountered by a drug or chemical after absorption from the gastrointestinal tract or peritoneal space also means that the liver often sees potential toxicants at their highest concentrations. The same drug or chemical at the same dose absorbed from the lungs or through the skin, for example, may be less toxic to the liver because the concentrations in blood reaching the liver are lower, from both dilution and distribution to other organs and tissues. A second reason for the susceptibility of the liver to chemical attack is that it is the primary organ for the biotransformation of chemicals within the body. As discussed in Chapter 3, the desired net outcome of the biotransformation process is generally to alter the chemical in such a way that it is (i) no longer biologically active within the body and (ii) more polar and water-soluble and, consequently, more easily excreted from the body. Thus, in most instances, the liver acts as a detoxification organ. It lowers the biological activity and blood concentrations of a chemical that might otherwise accumulate to toxic levels within the body. For example, it has been estimated that the time required to excrete one-half of a single dose of benzene would be about 100 years if the liver did not metabolize it. The primary disadvantage of the liver’s role as the main organ metabolizing chemicals, however, is that toxic reactive chemicals or short-lived intermediates can be formed during the biotransformation process. As a result the liver is frequently the major target of the toxicity associated with the reactive or toxic chemical metabolites it generates while attempting to convert a chemical into a more water-soluble and more easily eliminated form. The liver can be described as a large mass of cells packed around vascular trees of arteries and veins (see Figure 6.2). Blood supply to the liver comes from the hepatic artery and the portal vein, the former normally supplying about 20% of blood reaching the liver and the latter about 80%. Terminal branches of the hepatic artery and portal vein are found together with the bile duct (Figure 6.2). In cross section, these three vessels are called the portal triad. Blood is collected in the terminal hepatic venules, which drain into the hepatic vein. The functional microanatomy can be viewed in different ways. In one view, the basic unit of the liver is termed the lobule. Figure 6.2 Hepatic architecture, showing arrangement of blood vessels and cords of liver cells. Reproduced with permission from Hamilton (1976). © 1976 Elsevier. Blood enters the lobule from the hepatic artery and portal veins, traverses the lobule through hepatic sinusoids, and exits through a hepatic venule. In the typical lobule view, cells near the portal vein are termed periportal, while those near the hepatic venule are termed perivenular. The hepatic venule is visualized as occupying the center of the lobule, and cells surrounding the venule are sometimes termed centrilobular, while those farther away, near the portal triad, are called peripheral lobular. Rappaport proposed a different view of hepatic anatomy in which the basic anatomical unit is called the simple liver acinus. In this view (Figure 6.3, left), cells within the acinus are divided into zones. The area adjacent to small vessels radiating from the portal triad is zone 1. Cells in zone 1 are first to receive blood through the sinusoids. Blood then travels past cells in zones 2 and 3 before reaching the hepatic venule. As can be seen in Figure 6.3, zone 3 is roughly analogous to the centrilobular region of the classic lobule, since it is closest to the central vein. Zone 3 cells from adjacent acini form a star-shaped pattern around this vessel. Zone 1 cells surround the terminal afferent branches of the portal vein and hepatic artery, and are often stated as occupying the periportal region, while cells between zones 1 and 3 (i.e., in zone 2) are said to occupy the midzonal region. A modification of the typical lobule and acinar models has been provided by Lamers and colleagues (Figure 6.3, right). Based on histopathological and immunohistochemical studies, they propose that zone 3 should be viewed as a circular, rather than star-shaped, region surrounding the central vein. Zone 1 cells surround the portal tracts, and the zone 1 cells from adjacent acini merge to form a reticular pattern. As with the Rappaport model, cells in zone 3 may be described as centrilobular (matching closely the classic lobular terminology), cells in zone 1 as periportal, and the cells in zone 2 in between as midzonal. Figure 6.3 Alternative views of the liver acinus. Reproduced with permission from Lamers et al. (1989). © 1989 American Association for the Study of Liver Diseases. Each of these viewpoints has in common a recognition that the cells closest to the arterial blood supply receive the highest concentrations of oxygen and nutrients. As blood traverses the lobule, concentrations of oxygen and nutrients diminish. Differences in oxygen tension and nutrient levels are reflected in differing morphology and enzymatic content between cells in zones 1 and 3. Consistent with their greater access to oxygen, hepatocytes in zone 1 are better adapted to aerobic metabolism. They have greater respiratory activity, greater amino acid utilization, and higher levels of fatty acid oxidation. Glucose formation from gluconeogenesis or from the breakdown of glycogen predominates in zone 1 cells, and the maximum secretion of bile acids occurs here. On the other hand, most forms of the biotransformation enzyme cytochrome P450 are found in highest concentrations in zone 3 cells. As the site of biotransformation for most drugs and chemicals, zone 3 cells have the greatest responsibility for their detoxification. This also means that zone 3 cells are often the primary targets for chemicals that are bioactivated by these enzymes to toxic metabolites in the liver. There are several types of liver cells. Hepatocytes, or parenchymal cells, constitute approximately 75% of the total cells in the human liver. They are relatively large cells and make up the bulk of the hepatic lobule. By virtue of their numbers and their extensive xenobiotic metabolizing activity, these cells are the principal targets for hepatotoxic chemicals. The sinusoids are lined with endothelial cells. These cells are small but numerous, making up most of the remaining cells in the liver. The hepatic microvasculature also contains resident macrophages, called Kupffer cells. Although comparatively few in number, these cells play an important role in phagocytizing microorganisms and foreign particulates in the blood. While these cells are a part of the liver, they are also part of the immune system. They are capable of releasing reactive oxygen species and cytokines, and play an important role in inflammatory responses in the liver. The liver also contains Ito cells (also termed fat-storing cells, parasinusoidal cells, or stellate cells), which lie between parenchymal and endothelial cells. These cells appear to be important in producing collagen and in vitamin A storage and metabolism. All chemicals do not produce the same type of liver injury. Rather, the type of lesion or effect observed is dependent on the chemical involved, the dose, and the duration of exposure. Some types of injury are the result of acute toxicity to the liver, while others appear only after chronic exposure or treatment. Basic types of liver injury include the anomalies described in the following paragraphs. Many hepatotoxicants are capable of injuring liver cells directly, leading to cellular degeneration and death. A variety of organelles and structures within the liver cell can be affected by chemicals. Principal targets include the following: Not all hepatocellular toxicity leads to cell death. Cells may display a variety of morphological abnormalities in response to chemical insult and still recover. These include cell swelling, dilated endoplasmic reticulum, condensed mitochondria and chromatin material in the nucleus, and blebs on the plasma membrane. More severe morphological changes are indicative that the cell will not recover, and will proceed to cell death, that is, undergo necrosis. Examples of morphological signs of necrosis are massive swelling of the cell, marked clumping of nuclear chromatin, extreme swelling of mitochondria, breaks in the plasma membrane, and the formation of cell fragments. Necrosis from hepatotoxic chemicals can occur within distinct zones in the liver, be distributed diffusely, or occur massively. Many chemicals produce a zonal necrosis; that is, necrosis is confined to a specific zone of the hepatic acinus. Table 6.1 provides examples of drugs and chemicals that produce hepatic necrosis and the characteristic zone in which the lesion occurs. Figure 6.4 shows an example of zone 3 hepatic necrosis from acetaminophen. Confinement of the lesion to a specific zone is thought to be a consequence of the mechanism of toxicity of these agents and the balance of activating and inactivating enzymes or cofactors. Interestingly, there are a few chemicals for which the zone of necrosis can be altered by treatment with other chemicals. These include cocaine, which normally produces hepatic necrosis in zone 2 or 3 in mice, but in phenobarbital-pretreated animals causes necrosis in zone 1. Limited observations of liver sections from humans experiencing cocaine hepatotoxicity are consistent with this shift produced by barbiturates. The reason for the change in site of necrosis with these chemicals is unknown. Table 6.1 Drugs and Chemicals That Produce Zonal Hepatic Necrosis a Necrosis is shifted to zone 1 in phenobarbital-pretreated animals. Figure 6.4 Liver section from mouse given a hepatotoxic dose of acetaminophen. With acetaminophen, liver cell swelling and death characteristically occur in regions around the central vein (zone 3, arrow). Cells near the portal triad (arrow head) are spared. Necrotic cells produced by some chemicals are distributed diffusely throughout the liver, rather than being localized in acinar zones. Galactosamine and the drug methylphenidate are examples of chemicals that produce a diffuse necrosis. Diffuse necrosis is also seen in viral hepatitis and some forms of idiosyncratic liver injury. The extent of necrosis can vary considerably. When most of the cells of the liver are involved, this is termed massive necrosis. As the name implies, this involves destruction of most or all of the hepatic acinus. Not all the acini in the liver are necessarily affected to the same extent, but at least some acini will have necrosis that extends across the lobule from the portal triad to the hepatic vein, called bridging necrosis. Massive necrosis is not so much a characteristic of specific hepatotoxic chemicals as of their dose.
HEPATOTOXICITY: TOXIC EFFECTS ON THE LIVER
6.1 PHYSIOLOGICAL AND MORPHOLOGICAL BASES OF LIVER INJURY
Physiological Considerations
Morphological Consideration
6.2 TYPES OF LIVER INJURY
Hepatocellular Degeneration and Death
Chemical
Site of Necrosis
Zone 1
Zone 2
Zone 3
Acetaminophen
X
Aflatoxin
X
X
Allyl alcohol
X
Alloxan
X
α-Amanitin
X
Arsenic, inorganic
X
Beryllium
X
Botulinum toxin
X
Bromobenzene
X
Bromotrichoromethane
X
Carbon tetrachloride
X
Chlorobenzenes
X
Chloroform
X
Chloroprene
X
Cocainea
X
X
Dichlorpropane
X
Dioxane
X
DDT
X
Dimethylnitrosamine
X
Dinitrobenzene
X
Dinitrotoluene
X
Divinyl ether
X
Ethylene dibromide
X
Ethylene dichloride
X
Ferrous sulfate
X
Fluoroacetate
X
Iodobenzene
X
Iodoform
X
Manganese compounds
X
Methylchloroform
X
Naphthalene
X
Ngaione
X
Paraquat
X
Phalloidin
X
Pyridine
X
Pyrrolidizine alkaloids
X
Rubratoxin
X
Tannic acid
X
Thioacetamide
X
Urethane
X
Xylidine
X
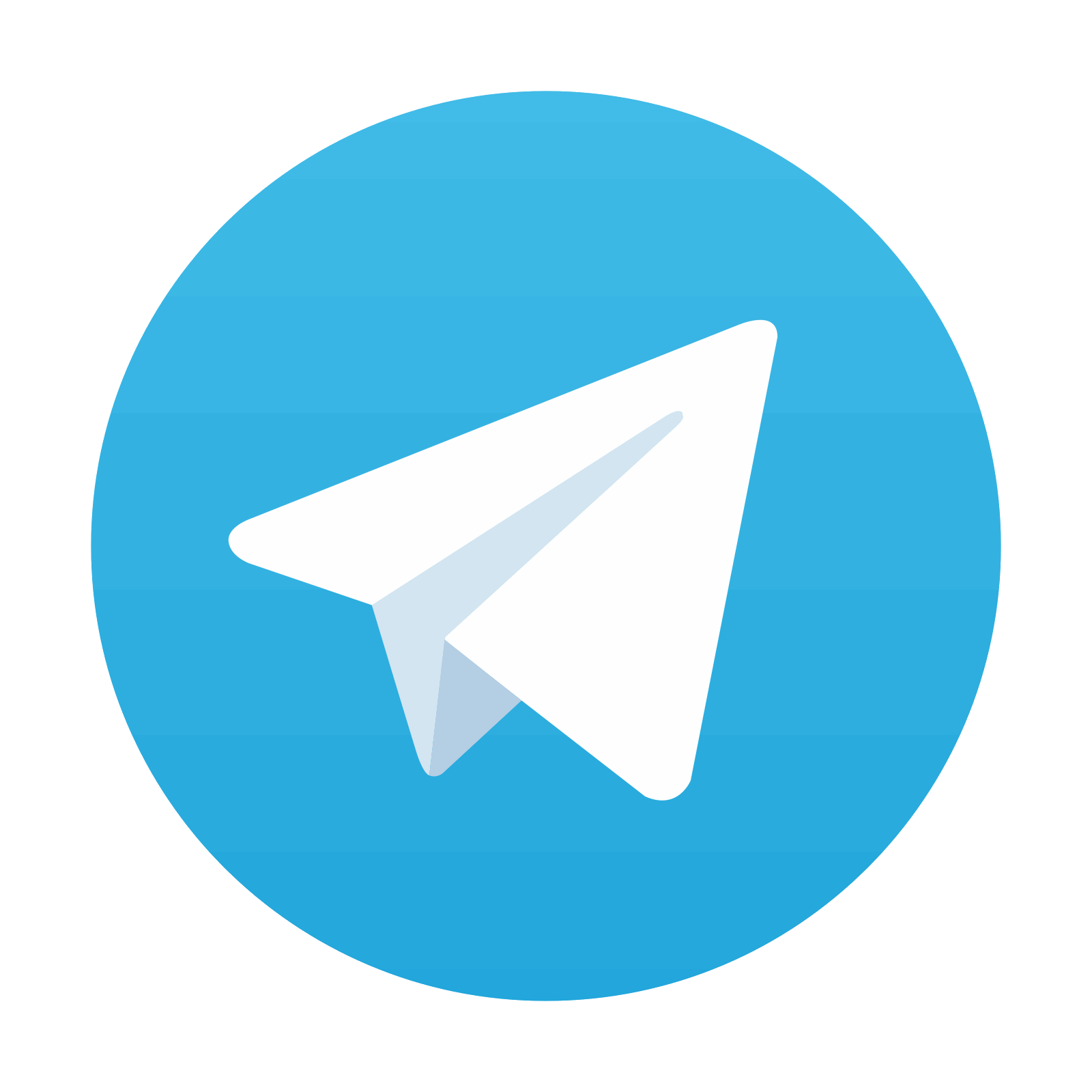
Stay updated, free articles. Join our Telegram channel

Full access? Get Clinical Tree
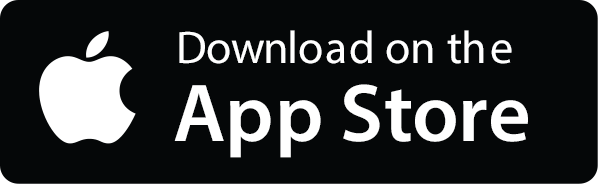
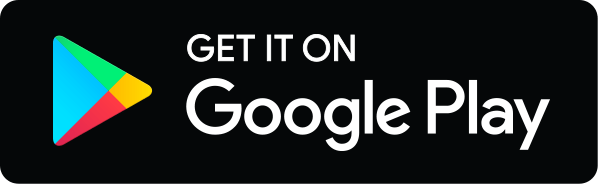