FIGURE 15-1. Schematic diagram of hematopoiesis.
In the fetus and children, blood cell formation or hematopoiesis occurs in the marrow of virtually all bones. With maturation, the task of hematopoiesis shifts to flat bones of the axial skeleton such as the cranium, ribs, pelvis, and vertebrae. The long bones, such as the femur and humerus, do not produce a large amount of blood cells in adulthood as the marrow is gradually replaced by fatty tissue. Radiation directed to large portions of hematopoietic bones can lead to deficient hematopoiesis in patients treated for cancerous lesions. Similarly, preparation for a bone marrow transplant will often include total body irradiation (TBI) to destroy the hematopoietic cells of the recipient in the bone, spleen, and other sites so that the grafted cells will not be destroyed by residual host defenses.
Although the majority of hematopoiesis occurs in the marrow, modern methods of identifying cellular characteristics have demonstrated that pluripotential cells—identified by a cellular expression of the surface marker CD34—also normally circulate in the blood.3,4
Although the majority of this chapter discusses laboratory analysis of blood obtained from the vein (peripheral venipuncture), an analysis of the bone marrow itself may be needed to diagnose or monitor various disease states, most commonly the leukemias discussed later in the chapter. Bone marrow specimens are usually obtained from the posterior iliac crest of the pelvis or, less commonly because of increased risk, from the sternum. Bone marrow sampling can involve an aspirate, a core biopsy, or both. After penetrating the bone cortex with the bone marrow needle and entering the medullary cavity inside the bone containing the marrow, a heparinized syringe is attached to the needle and 1–2 mL of bone marrow is aspirated. The contents of the syringe are smeared on a series of slides that are stained and examined microscopically. If special studies such as flow cytometry or cytogenetics are requested, additional heparinized syringes are aspirated and submitted to the laboratory. The bone marrow biopsy is obtained with the same needle by advancing it further past the site of aspiration through the bone marrow to cut a sample of the bone marrow matrix for removal and examination. The biopsy provides the advantage of examining the structure of the marrow stroma, as well as the spatial relationship of the various hematopoietic cells.5
Blood stem cells become increasingly differentiated in the bone marrow until they are committed to develop further into erythrocytes, platelets, or various leukocytes (Figure 15-1). Many regulatory proteins are involved in the differentiation and proliferation phases of hematopoiesis, but their functions and interrelationships are not yet fully understood. In addition to the colony-stimulating factors mentioned above, proteins that stimulate hematopoiesis include erythropoietin, thrombopoietin, and various interleukins. Inhibitors of hematopoiesis are not as well-defined but include interferons and lymphotoxins. In considering the response of neutrophils or erythrocytes to exogenously administered hematopoietic stimulants (e.g., filgrastim or erythropoietin), it is important to recall that normal physiologic hematopoietic regulation is more complex than the effect of one therapeutic protein would suggest. White blood cell formation involves local production of a combination of signaling proteins by cells of the hematopoietic microenvironment (e.g., macrophages, T lymphocytes, osteoblasts, fibroblasts, and endothelial cells). Leucocyte-stimulating proteins such as granulocyte colony-stimulating factor (G-CSF) and granulocyte-macrophage colony-stimulating factor (GM-CSF) are normally directed toward adjacent or closely approximated differentiating hematopoietic cells.4 In contrast, erythropoietin is formed and released to the systemic circulation by the kidney, affecting erythrocyte precursors in the blood-forming areas of bone marrow.
Committed blood precursor cells undergo further differentiation in the bone marrow until they develop into mature cells. These developmental stages can be identified by differing morphological or immunochemical staining characteristics. These same imaging techniques are used to identify the developmental phenotype of the cancerous white cells of leukemia and lymphoma. Generally, only mature cellular forms are found in the circulating blood, and it is from this blood that clinical specimens are usually taken. As discussed below, the presence of immature forms of white or red cells in the blood typically indicates the presence of a pathologic process.
COMPLETE BLOOD COUNT
The complete blood count (CBC) is a frequently ordered laboratory test. It supplies useful information regarding the concentration of the different cellular and noncellular elements of blood and applies to multiple disorders. Complete blood count is a misnomer because concentrations of cells/µL, not counts, are measured and reported. Functionally, the CBC can be thought of as a complete blood analysis because a series of tests are performed. Moreover, information besides concentrations is reported.
Most clinical laboratories utilize automated hematology analyzers to determine the CBC. Results are usually accurate, reproducible, and rapidly obtained. Numerous measured and calculated values are included in a CBC (Table 15-1). These results traditionally include
Hct = hematocrit; Hgb = hemoglobin; MCHC = mean corpuscular hemoglobin concentration; MCV = mean corpuscular volume; MPV = mean platelet volume; RBC = red blood cell; RDW = RBC distribution width; WBC = white blood cell.
aModified from references 8 and 9.
- Leukocyte (WBC) count
- Erythrocyte (RBC) count
- Hgb
- Hct
- RBC indices (mean corpuscular volume [MCV], mean corpuscular hemoglobin concentration [MCHC]), and RBC distribution width (RDW)—the RBC indices are also commonly referred to as Wintrobe indices
- Platelet count and mean platelet volume (MPV)
- Reticulocyte count
When a “CBC with differential” is ordered, the various types of WBCs are also analyzed (White Blood Cell Count and Differential section). The reliability of the results can be doubtful if (1) the integrity of the specimen is questionable (inappropriate handling or storage), or (2) the specimen contains substances that interfere with the automated analysis. Grossly erroneous results are usually flagged for verification by another method. Manual microscopic review of the blood smear is used to resolve unusual automated results.1
Mature erythrocytes have a median lifespan of 120 days under normal conditions. They are removed from the circulation by macrophages in the spleen and other reticuloendothelial organs. The erythrocytes are tested for flexibility, size, and integrity in these organs as the cells pass through areas of osmotic, pH, or hypoxic stress.6
Variability in the size of red cells is termed anisocytosis, and any variation in the normal biconcave disc shape is termed poikilocytosis. Such abnormalities are seen with iron deficiency or periods of increased erythrocyte production and/or red cell damage.7
Normal adult range:
males: 4.5–5.9 × 106 cells/µL or 4.5–5.9 × 1012 cells/L;
females: 4.1–5.1 × 106 cells/µL or 4.1–5.1 × 1012 cells/L
The red blood cell (RBC) count is the number of red corpuscles in a given volume of blood. The International Unit for reporting blood cells is for a 1-liter volume, but it is still common to see values reported in cells/microliter (µL) or less commonly in cells/cubic millimeter (mm3). After puberty, females have slightly lower counts (and Hgb) than men, partly because of their menstrual blood loss and because of higher concentrations of androgen (an erythropoietic stimulant) in men. The RBC count in all anemias is by definition below the normal range. This decrease causes a proportionate decrease in Hct and Hgb.
The reticulocyte is the cell form that precedes the mature RBC or erythrocyte. During the entire maturation process, Hgb is produced, gradually filling the cytoplasm. The reticulocyte does not contain a nucleus but possesses nucleic acids that can be considered remnants of the nucleus or endoplasmic reticulum. The mature erythrocyte contains neither an organized nucleus nor nucleic acids. Reticulocytes persist in the circulation for 1–2 days before maturing into erythrocytes.2
White Blood Cell Count
Normal range: 4.4–11.0 × 103 cells/mm3 or 4.4–11.0 × 109 cells/L
The WBC count is an actual count of the number of leukocytes in a given volume of blood. Unlike RBCs, leukocytes have a nucleus and normally represent five different mature cell types. The various percentages of the five mature and WBC types comprise the WBC differential, which is discussed later in this chapter.
Hemoglobin
Normal range: males: 14–17.5 g/dL or 140–175 g/L;
females: 12.3–15.3 g/dL or 123–153 g/L
The hemoglobin (Hgb) value is the amount of this metalloporphyrin-protein contained in a given volume (100 mL or 1 L) of whole blood. The Hgb concentration provides a direct indication of the oxygen-transport capacity of the blood. As the major content of the RBCs, Hgb is proportionately low in patients with anemia.
Normal range: males: 42% to 50% or 0.42–0.50;
females: 36% to 45% or 0.36–0.45
Hematocrit (Hct) is the percentage volume of blood that is composed of erythrocytes. It is also known as the packed cell volume. To manually perform the Hct test, a blood-filled capillary tube is centrifuged to settle the erythrocytes. Then, the percentage volume of the tube that is composed of erythrocytes is calculated.5 The Hct is usually about 3 times the value of the Hgb, but disproportion can occur when cells are substantially abnormal in size or shape. Like Hgb, Hct is low in patients with anemia.
Red Blood Cell Indices
Because the following laboratory tests specifically assess RBC characteristics, they are called RBC indices. These indices are useful in the evaluation of anemias, polycythemia, and nutritional disorders. Essentially, they assess the size and Hgb content of the RBC. The MCV is measured directly while the MCHC and mean corpuscular hemoglobin (MCH) are calculated from the Hgb, MCV, and RBC count using predetermined formulas. Due to its dependence on cell size, MCH is not used clinically, but rather the MCHC is used to assess RBCs for their Hgb concentration and color.
Mean Corpuscular Volume
Normal range: 80–96 fL/cell
The mean corpuscular volume (MCV) is an estimate of the average volume of RBCs and is the most clinically useful of the RBC indices. It can be calculated by dividing the Hct by the RBC count, but it is ordinarily determined by averaging the directly measured size of thousands of RBCs.
Abnormally large cells have an increased MCV and are called macrocytic. Vitamin B12 and/or folate deficiency cause the formation of macrocytic erythrocytes, which corresponds to a true increase in MCV. In contrast, a false increase in MCV may be observed when a patient has reticulocytosis, an increase in the number of reticulocytes in the peripheral blood, since reticulocytes are larger than mature erythrocytes.5 The MCV may also be falsely increased in hyperglycemia due to osmotic expansion of the erythrocyte. When erythrocytes are mixed with diluting fluid to perform the test, the cells swell because the diluent is relatively hypotonic compared to the patient’s hyperglycemic blood. Abnormally small cells (with a decreased MCV) are called microcytic. A decrease in the MCV implies some abnormality in Hgb synthesis. The most common cause of microcytic erythrocytes (microcytosis) is iron deficiency.8
Mean Corpuscular Hemoglobin Concentration
Normal range: 33.4–35.5 g/dL or 334–355 g/L
The mean corpuscular hemoglobin concentration (MCHC) is the Hgb divided by the Hct. As mentioned previously, this calculation is usually around 33 g/dL (330 g/L) because the Hct is usually 3 times the Hgb. Iron deficiency is the only anemia in which the MCHC is routinely low, although it can also be decreased in other disorders of Hgb synthesis.8,9 In this case, RBCs are described as hypochromic (pale). It can be falsely elevated in hyperlipidemia. It must be considered that the Wintrobe indices are averages for the patient’s blood and that normal values may be reported by automated methods even in the presence of a mixed (normal + abnormal) erythrocyte population.
Red Blood Cell Distribution Width
Normal range: 11.5% to 14.5% or 0.115–0.145
The RBC distribution width (RDW) is an indication of the variation in red cell size, termed anisocytosis.8 The RDW is reported as the coefficient of variation of the MCV (standard deviation/mean value). This value is used primarily with other tests to differentiate iron deficiency anemia from thalassemias. The RDW increases in macrocytic anemias and in early iron deficiency, often before other tests show signs of this kind of anemia. However, it is not specific for iron deficiency anemia. Mild forms of thalassemia often are microcytic but have a normal or only slightly elevated RDW.
Reticulocyte Count
Normal range: 0.5% to 2.5% of RBCs or 0.005–0.025
In anemia, the reticulocyte count or reticulocyte index (RI) reflects not only the level of bone marrow production but also a decline in the total number of mature erythrocytes that normally dilute the reticulocytes. Therefore, the reticulocyte count would double in a person whose bone marrow production is unchanged, but whose Hct has fallen from 46% to 23%. The RI was used with hand-counting methods to correct for a low Hct, but it is not necessary with modern, automated cell counters.11
In persons with anemia secondary to acute blood loss or hemolysis, even the corrected reticulocyte count is increased.6 This increase reflects an attempt by the bone marrow to compensate for the lack of circulating erythrocytes. Because RBC production is increased to far above basal activity, more reticulocytes escape into the circulation earlier than normal. In contrast, persons with untreated anemia secondary to iron, folate, or vitamin B12 deficiency are unable to increase their reticulocyte count appropriate to the degree of their anemia. Similarly, appropriate treatment of an anemia should be accompanied by an increase in the reticulocyte count.
The reticulocyte count can be useful in identifying drug-induced bone marrow suppression in which the percentage of circulating reticulocytes should be close to zero. It can also be used to monitor an anemic patient’s response to vitamin or iron therapy. In such patients, supplementation of the lacking factor causes rapid (5–7 days) elevation of the reticulocyte count.
ERYTHROCYTE SEDIMENTATION RATE
Normal range: males: 1–15 mm/hr; females: 1–20 mm/hr (increases with age)
Numerous physiological and disease states are associated with the rate at which erythrocytes settle from blood, termed the erythrocyte sedimentation rate (ESR). Erythrocytes normally settle slowly in plasma but settle rapidly when they aggregate because of electrostatic forces. Each cell normally has a net negative charge and repels other erythrocytes because like charges repel each other. Many plasma proteins are positively charged and are attracted to the surface charge of one or more erythrocytes, thereby promoting erythrocyte aggregation.12 Nonmicrocytic anemia, pregnancy, multiple myeloma, and various inflammatory diseases (including infections) can elevate the ESR (Table 15-2). Sickle cell disease, high doses of corticosteroids, liver disease, microcytosis, carcinomas, and congestive heart failure can decrease the ESR.8
ESR = erythrocyte sedimentation rate.
Although the ESR may be used to confirm a diagnosis supported by other tests, it is rarely used alone for a specific diagnosis. Rather, the ESR is useful for monitoring the activity of inflammatory conditions (e.g., temporal arteritis, polymyalgia rheumatica, rheumatoid arthritis, and osteomyelitis).12 The ESR is often higher when the disease is active due to increased amounts of circulating proteins, termed acute phase reactants (e.g., fibrinogen), and falls when the intensity of the disease decreases.
The ESR is usually measured using either the Wintrobe or the Westergren method. Anticoagulated blood is diluted and placed in a perfectly vertical glass tube of standard size. After 1 hour, the distance from the plasma meniscus down to the top of the erythrocyte column is recorded as the ESR in millimeters per hour.12 A corrected sedimentation rate, called the zeta-sedimentation rate or ratio, has been developed to eliminate the effect of anemia on the ESR.13 This value is called the zetacrit, and its normal range is 40% to 52%.8 Elevations above the normal range are interpreted in the same manner as an elevated ESR by traditional methods.
PLATELET COUNT AND MEAN PLATELET VOLUME
Normal ranges: 150,000–450,000 cells/µL
The platelet count, often included routinely in the CBC with differential, is discussed with other coagulation tests in Chapter 16: Hematology: Blood Coagulation Tests.
LABORATORY ASSESSMENT OF ANEMIA
The functions of the erythrocyte are to transport and protect Hgb, the molecule used for oxygen and carbon dioxide transport. Anemia can be caused as a decrease in either the HCT or the Hgb concentration below the normal range for age and gender. Anemia is not a disease in itself but one manifestation of an underlying disease process. Appropriate treatment of the anemic patient depends on the exact cause of the condition. Signs and symptoms of anemia depend on its severity and the rapidity with which it has developed. Severe, acute blood loss results in more dramatic symptoms than an anemia that took months to develop because with chronic loss some compensatory adaptation may occur. Patients with mild anemia are often asymptomatic (i.e., absence of pallor, weakness, and fatigue), but severely symptomatic patients manifest shortness of breath, tachycardia, and palpitations even at rest. This contrast should be kept in mind when interpreting test results.
Anemia can be caused by decreased production and/or increased destruction of erythrocytes as well as acute blood loss. The first two situations can often be differentiated by the reticulocyte count, which is decreased in the former and increased in the latter.
Use of erythrocyte morphology is one common method to characterize the possible etiology of anemia. This method is useful because different causes of anemia lead to different erythrocyte morphology. Figure 15-2 outlines this approach. Only a few common causes of anemia are included, but others can fit into this outline. Other laboratory tests that are useful in differentiating the anemias are described below. Usual laboratory findings are also included in each section (Table 15-3).
Hct = hematocrit; Hgb = hemoglobin; MCHC = mean corpuscular hemoglobin concentration; MCV = mean corpuscular volume; RBC = red blood cell; RDW = RBC distribution width; TIBC = total iron-binding capacity.
aSome tests with no change (↔) are left empty for clarity.
bAutoantibodies positive for antibody-mediated immune hemolysis.
FIGURE 15-2. Use of erythrocyte morphology in differential diagnosis of anemia.
Macrocytic Anemia
Macrocytic anemia is a lowered Hgb value characterized by abnormally enlarged erythrocytes. The two most common causes are vitamin B12 and/or folic acid deficiencies. Drugs that cause macrocytic anemia mainly interfere with proper utilization, absorption, and metabolism of these vitamins (Table 15-4).
Vitamin B12 Deficiency
Vitamin B12 is also known as cobalamin. The normal daily requirement of vitamin B12 is 2–5 mcg.14 It is stored primarily in the liver, which contains approximately 1 mcg of vitamin/g of liver tissue. Overall, the body has B12 stores of approximately 2–5 mg. Therefore, if vitamin B12 absorption suddenly ceased in a patient with normal liver stores, several years would pass before any abnormalities occurred due to vitamin deficiency.
The absorption of vitamin B12 is complex, and the mechanisms responsible are still being defined. Cobalamin (vitamin B12) is ingested in meats, eggs, and dairy products. Strict vegans, who avoid all such foods in their diet, may over time develop vitamin B12 deficiency if supplements are not ingested. Some forms of B12, such as those made by the blue–green algae Spirulina, are active vitamins in bacterial assays but are not active vitamins for humans. Other cobamides structurally related to cobalamin are found in plasma after ingesting other animal and plant-based foods. Only the cobamide with an attached 5,6-dimethylbenzimidazole group is correctly termed cobalamin and is active in humans.14
Dietary B12 or cobalamin is usually bound nonspecifically to proteins, and gastric acid and pepsin are required to hydrolyze the vitamin from the protein. Aging patients with decreasing stomach acid production may be less able to free vitamin B12 from meat protein. Freed B12 is bound with very high affinity to protein R, which is a large protein secreted in saliva. The cobalamin-protein R complex moves to the duodenum where pancreatic proteases denature protein R and allow the freed vitamin to bind to intrinsic factor, which is secreted in the stomach and is resistant to the intestinal proteases. Patients may develop autoantibodies to intrinsic factor, and, thereby, develop vitamin B12 deficiency. The cobalamin-intrinsic factor complex is transported into the ileal epithelium and dissociates, and the cobalamin enters the circulation on the basolateral side of the cell bound to transcobalamin, which is largely homologous with intrinsic factor. Clearly, there are several steps in the absorption of vitamin B12 that may be responsible for a deficiency.
A deficiency of cobalamin (vitamin B12) may arise from inadequate intake of the vitamin, or a deficiency of the intrinsic factor required for the effective ileal absorption of the vitamin. Inadequate dietary intake is a rare cause of vitamin B12 deficiency, usually occurring only in vegans who abstain from all animal food including milk and eggs.14 On the other hand, defective production of intrinsic factor is a common cause of the deficiency.16 The gastric mucosa can fail to secrete intrinsic factor because of atrophy, especially in the elderly.
Clinical and laboratory diagnosis. Vitamin B12 is necessary for deoxyribonucleic acid (DNA) synthesis in all cells, for the synthesis of neurotransmitters, and for metabolism of homocysteine. Therefore B12 deficiency leads to signs and symptoms involving many organ systems.14,15 The most notable symptoms involve
- Gastrointestinal (GI) tract (e.g., loss of appetite, smooth and/or sore tongue, and diarrhea or constipation)
- Central nervous system (CNS) (e.g., paresthesias in fingers and toes, loss of coordination of legs and feet, tremors, irritability, somnolence, and abnormalities of taste and smell)
- Hematopoietic system (anemia)
Nuclear maturation retardation occurs in the developing cells in the bone marrow due to slowed DNA synthesis. The morphological result is cells with immature enlarged nuclei (megaloblasts) but cytoplasm that matures normally, resulting in mature cells larger than normal. The resulting anemia is called a macrocytic, megaloblastic (both morphological characteristics of nuclear maturation retardation) anemia.15 Visual inspection of smears of both peripheral blood and bone marrow reveals characteristic megaloblastic changes in the appearance of erythrocytes (oval macrocytes) and WBCs. The development of neutrophils is also affected, resulting in large cells with hypersegmentation (>3 nuclear lobes). A mild pancytopenia—decreased numbers of all blood elements—also occurs. The usual laboratory test results found with vitamin B12 deficiency are listed in Table 15-3.
Serum cobalamin (vitamin B12) concentrations were in the past measured using a microbiologic assay and a cobalamin-dependent organism. This methodology assay has largely been replaced by a competitive displacement assay using radioactive cobalamin and intrinsic factor. Unfortunately, because of cross-reactivity with other cobamides, approximately 5% of patients will have cobalamin concentrations that appear to be within the normal range yet can be shown to have hematologic and/or neurologic signs of deficiency. There is increasing acceptance of the use of serum levels of the metabolic intermediates homocysteine and methylmalonate as more sensitive indicators of cobalamin deficiency. In the presence of inadequate cobalamin, these two compounds accumulate because of the cobalamin-dependence of their metabolizing enzymes, methionine synthase, and methylmalonyl-CoA-mutase, respectively. An elevated serum methylmalonate and plasma homocysteine concentration in the presence of normal RBC folate is strongly indicative of a pure deficiency of cobalamin.
Historically, the Schilling test was used to determine if impaired absorption is the reason for the cobalamin deficiency. In the Schilling test, cyano-(57Co)-cobalamin is administered orally and is allowed to be absorbed, if possible. An unlabelled, intravenous (IV) injection of cobalamin follows and will displace some of the absorbed radioactive 57Co-cobalamin from circulating transcobalamin, which is excreted and measured in the urine. If oral absorption is normal (e.g., the deficiency was caused by inadequate dietary intake), >8% of the radioactive cobalamin will be excreted in the urine. If abnormal, the test can be repeated at a later date (stage II test), adding exogenous intrinsic factor to the dose of oral labeled cyano(57Co)-cobalamin to determine if inadequate urinary excretion secondary to poor absorption was due to a relative lack of the protein.15 The availability of intramuscular injections of vitamin B12 obviates the need to specify the defect in B12 absorption and are favored in patients with impaired cobalamin absorption, regardless of the cause. Oral cobalamin can also be used in most patients with intrinsic factor deficiency, but regular monitoring is required to ensure efficacy and adherence to dosing. The availability of cobalamin supplementation and the complexity of the procurement and disposal of radioactive tracers discourage the use of the Schilling test.15
Folic Acid Deficiency
Folic acid is also called pteroylglutamic acid. Folates refer to folic acid or reduced forms of folic acid that may have variable numbers of glutamic acid residues attached to the folic acid molecule. The folates present in food are mainly in a polyglutamic acid form and must be hydrolyzed in the intestine to the monoglutamate form to be absorbed efficiently. The liver is the chief storage site. Adult daily requirements are approximately 50 mcg of folic acid, equivalent to about 400 mcg of food folates. Folate stores are limited, and anemia arising from a folate-deficient diet occurs in 4–5 months.14
Inadequate dietary intake is the major cause of deficiency. Folates are found in green, leafy vegetables such as spinach, lettuce, and broccoli. Inadequate intake can have numerous causes: alcoholics classically have poor nutritional intake of folic acid; certain physiological states such as pregnancy require an increase in folic acid; malabsorption syndromes (mentioned in the section on vitamin B12) can also lead to defective absorption of folic acid; and celiac sprue can lead to folate malabsorption.17
Certain medications (e.g., methotrexate, trimethoprim-sulfamethoxazole, and triamterene) can act as folic acid antagonists by interfering with the conversion of folic acid into its metabolically active form, tetrahydrofolic acid. Phenytoin and phenobarbital administration can interfere with the intestinal absorption or utilization of folic acid (see Table 15-4).19
Folic acid is required as the intermediate for one-carbon transfers in several biochemical pathways. After absorption, folate is reduced to tetrahydrofolate, and a carbon in one of several oxidation states is attached for transfer. Most transfer processes allow facile regeneration, but the majority of folate circulates as 5-methyl-tetrahydrofolate. Vitamin B12 is required as a cofactor for the removal of the 5-methyl group to regenerate tetrahydrofolate, and in this step methionine is formed from homocysteine. A deficiency of vitamin B12 will therefore lead to an accumulation of 5-methyl-tetrahydrofolate at the expense of other functional folate forms (the “folate trap”). Increased supplementation with folic acid cannot overcome the inability of a vitamin B12-deficient human to form methionine from homocysteine, leading to neurologic and vascular toxicities from insufficient methionine and excessive homocysteine.
Clinical and laboratory diagnosis. Since folic acid is necessary for DNA synthesis, a deficiency causes maturation retardation in the bone marrow similar to that caused by vitamin B12 deficiency. Folic acid deficiency is also characterized by a macrocytic, megaloblastic anemia.20 (See Minicase 1.) However, with folic acid deficiency, pancytopenia does not develop as consistently as it does with vitamin B12 deficiency.
Anemia with Increased MCV
ANDREW B., A 45-YEAR-OLD MALE ALCOHOLIC, was admitted to the hospital because of pneumonia. His physical exam revealed an emaciated patient with ascites, dyspnea, fever, cough, and weakness. No cyanosis, jaundice, or peripheral edema was evident. His peripheral neurologic exam was within normal limits as were his serum electrolytes, urea nitrogen, creatinine, and glucose. The following CBC was obtained:
TEST NAME | RESULT | REFERENCE RANGE |
RBC | 3.0 × 106 cells/µL | 4.1–5.1 × 106 cells/µL for females |
WBC | 4.6 × 103 cells/µL | 4.4–11.0 × 103 cells/µL |
Hgb | 12.3 g/dL | 12.3–15.3 g/dL for females |
Hct | 33.9% | 36% to 45% for females |
MCV | 110.8 fL/cell | 80–96 fL/cell |
RDW | 15.4% | 11.5% to 14.5% |
Platelet | 174,000 cells/µL | 150,000–450,000 cells/count µL |
Neutrophils | 68% | 45% to 73% |
Bands | 6% | 3% to 5% |
Monocytes | 11% | 2% to 8% |
Eosinophils | 2% | 0% to 4% |
Basophils | 2% | 0% to 1% |
Lymphocytes | 11% | 20% to 40% |
Question: What abnormalities are present? What is the likely cause?
Discussion: Andrew B. has anemia, evidenced by the low RBC, Hgb, and Hct. The increased MCV identifies this as a macrocytic anemia. The RDW is elevated, indicating variability in the size of the erythrocytes. These findings are typical of folic acid deficiency, a common finding in alcoholics due to poor nutrition. Folic acid deficiency is more common than vitamin B12 deficiency because body stores are not durable. However, vitamin B12 deficiency must also be ruled out as it may arise with or without a concurrent folate deficiency. Hypovitaminosis B12 may arise from poor nutrition but is more commonly caused by pathologies such as pernicious anemia. It is critical that both serum folate and vitamin B12 concentrations be drawn in Andrew B. to guide appropriate supplementation. Replenishment of folate in a patient with hypovitaminosis B12 may temporarily improve the values of the hemogram, but failure to appropriately replenish vitamin B12 can lead to irreversible brain and nerve damage.
Pernicious anemia is a separate disease characterized by atrophic gastritis associated with antibodies against intrinsic factor and gastric parietal cells. Gastrectomy, removal of all or part of the stomach, can also lead to vitamin B12 deficiency because the procedure removes the production site of intrinsic factor. Achlorhydria from gastrectomy or drugs can decrease the release of cobalamin from meat. Defective or deficient absorption of the intrinsic factor vitamin B12 complex can be caused by inflammatory disease of the small bowel, ileal resection, and bacterial overgrowth in the small bowel.17,18 Administration of colchicine, neomycin, and para-aminosalicylic acid can lead to impaired absorption of vitamin B12 (Table 15-4).
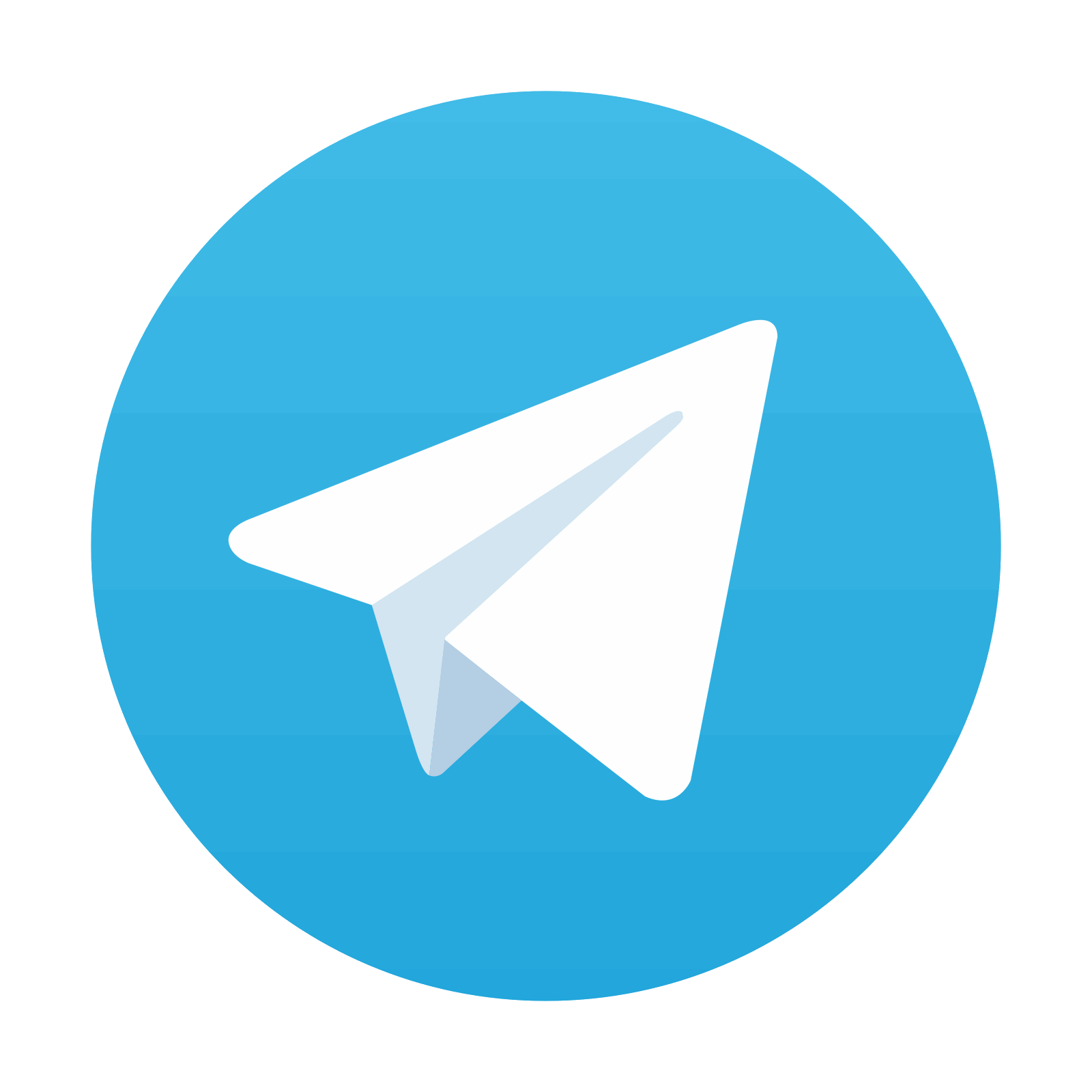
Stay updated, free articles. Join our Telegram channel

Full access? Get Clinical Tree
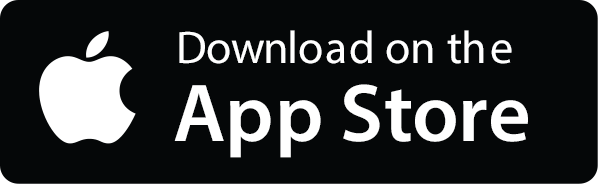
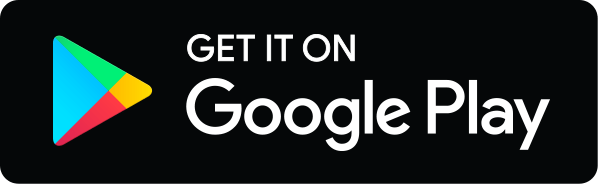