Chapter 12 Plant growth regulators
AUXINS
In the plant, oxidative degradation of IAA to give a number of products is controlled by IAA oxidase. Some substances such as the orthodiphenols (e.g. caffeic and chlorogenic acids and quercetin) inhibit the action of the enzyme and, hence, stimulate growth themselves. Conversely, monophenols such as p-coumaric acid promote the action of IAA oxidase and so inhibit growth. IAA may also be conjugated in the plant with aspartic acid, glutamic acid, glycine, sugars and cyclitols; such bound forms may represent a detoxication mechanism or are inactive storage forms of the hormone.
GIBBERELLINS
The biogenetic pathways of the gibberellins appear to be similar in both higher plants and Gibberella. They arise at the C20 geranylgeranyl pyrophosphate level of the isoprenoid mevalonic acid pathway (q.v.) with cyclizations giving the C20 tetracyclic diterpernoid entkaurenoic acid, which by a multistep ring contraction furnishes the gibbane ring system as exemplified by the key intermediate GA12-aldehyde. Several pathways diverge from GA12-aldehyde to give the known 90 or so gibberellins. All GAs have either the ent-gibberellane (C20 GAs) or the ent-20-norgibberellane (C19 GAs) (loss of C-20) carbon skeleton. Both types are modified by the position and number of OH groups, oxidation state of C-18 and C-20, lactone formation, presence and position of double bonds on ring A, epoxide formation, the presence of a carboxyl group and hydration of the C16–C17 double bond.
< div class='tao-gold-member'>
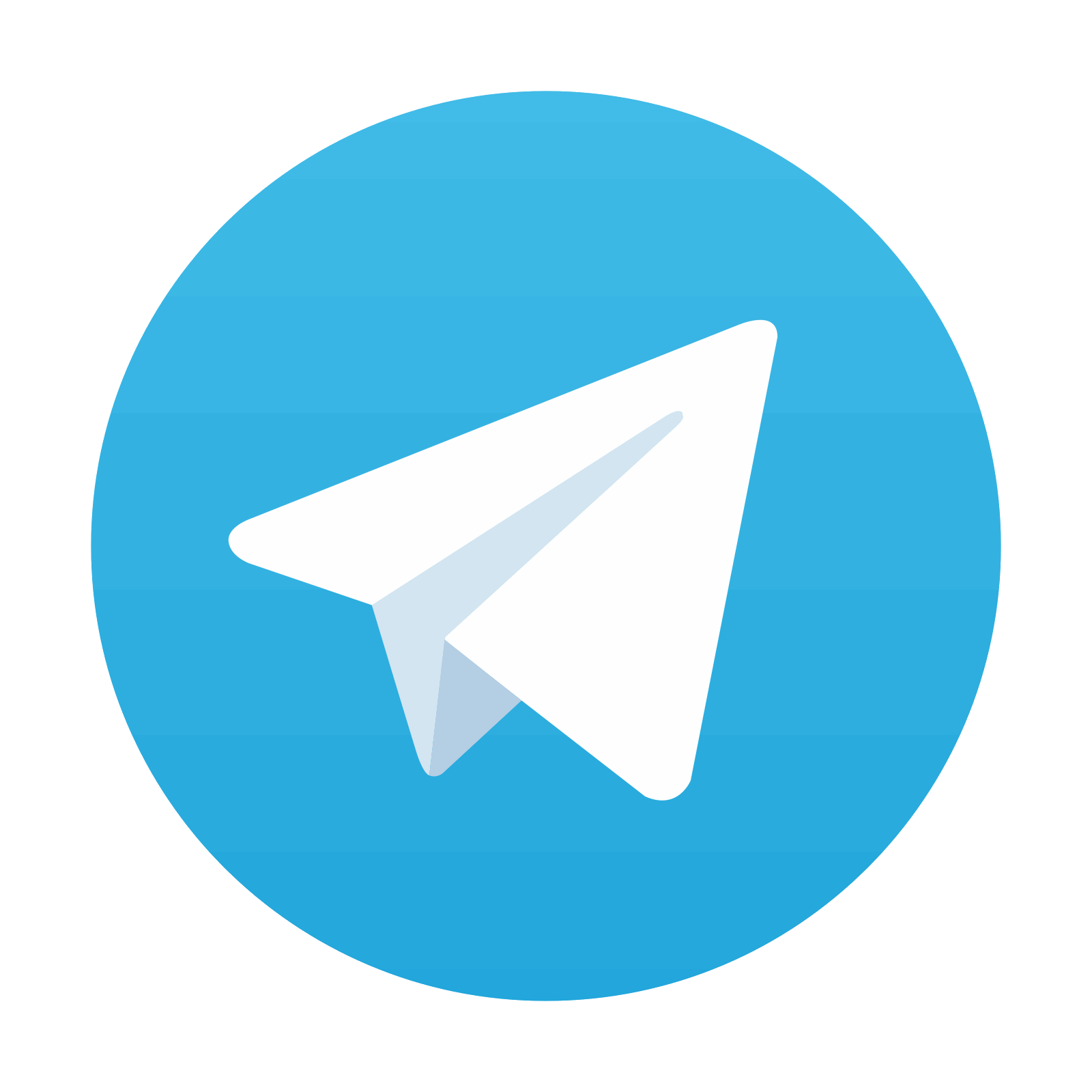
Stay updated, free articles. Join our Telegram channel

Full access? Get Clinical Tree
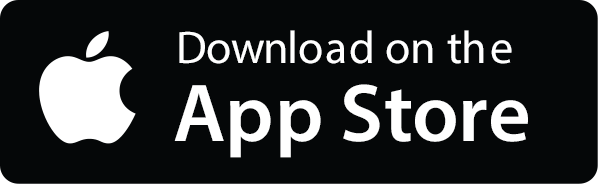
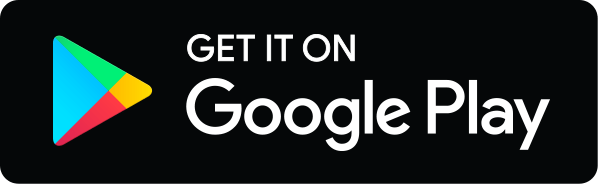