(1)
where the superscript i = c, m denotes collagen fiber and smooth muscle, respectively,



Indeed, the strain energy of constituent i at time t per unit reference area, W i (t), is given by:

where Ψ i denote strain energy of constituent i per unit mass, Q i is the mass fraction of constituent i generated at time zero and still exists at time t, and survival function q i is the mass fraction of constituent i generated at time τ and still survives at time t. The following equation is commonly used for survival function of constituent i in absence of ILT:
represents the maximum time that constituent i may survive and
is a function of mechanical contribution of the artery’s wall such as wall shear stress (WSS), circumferential stress, or stretch accounting for the rate of degradation of constituent i. The expression introduced in [26] is used such that:
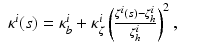
where ζ i and
denote tension in constituent i and its homeostatic value, respectively. In addition,
and
represent the basal rate of degradation of constituent i and the sensitivity of the rate of degradation to the stretch of constituent i, respectively.

(2)
![$$\displaystyle\begin{array}{rcl} q^{i}(\tau,t) = \left \{\begin{array}{@{}l@{\quad }l@{}} \exp \left [-\int _{\tau }^{t}\kappa ^{i}(s)ds\right ]\ \ \ \ \quad &t-\tau \leq a_{\max }^{i} \\ 0\ \ \ \ \quad &t-\tau> a_{\max }^{i}, \end{array} \right.& &{}\end{array}$$
” src=”/wp-content/uploads/2016/10/A321864_1_En_2_Chapter_Equ3.gif”></DIV></DIV><br />
<DIV class=EquationNumber>(3)</DIV></DIV>where <SPAN id=IEq4 class=InlineEquation><IMG alt=](/wp-content/uploads/2016/10/A321864_1_En_2_Chapter_IEq4.gif)

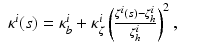
(4)



Aforementioned in the introduction, the ILT may lead to increased degradation and/or decreased production of smooth muscle cells and collagen fibers in the AAA wall. This process is done by the proteolytic enzymes released by the inflammatory cells. Here we divide this process into two categories: first, the inflammatory cells trapped in the luminal layer of the ILT release proteolytic enzymes up to a depth of about 1. 0 cm in the luminal side of the ILT (we call it primary proteolytic activity), and second, in case of forming a thick ILT, the oxygen content near the AAA wall decreases significantly and, as a result, neo-vessels are formed near that area. Neo-vessels are known as the sources of inflammatory cells which, by themselves, release proteolytic enzymes. Indeed, mast cells, which are found in the vicinity of the neo-vessels, activate the proteolytic enzymes (we account for this secondary proteolytic activity happening because of hypoxia).
The significance of the primary proteolytic activity depends on the concentration of active proteolytic enzymes in the AAA wall beneath the ILT (C p ). Similarly, the severity of proteolytic activity due to hypoxia depends on the oxygen amount (
) delivered to the AAA wall from lumen through the ILT such that the more loss in the oxygen content leads to the more proteolytic activity. Accordingly, the concentration of active proteolytic enzymes in an AAA wall is given by C h p and
in absence of the ILT. Beneath the ILT, the oxygen concentration in the lumen, the following equations give the modified versions of Eqs. (1) and (4) for the final rate of production and degradation of constituent i accounting for the effect of both primary and secondary proteolytic activity:


where
and
denote parameters representing the sensitivity of the production and degradation of constituent i to the primary proteolytic activity, respectively; where
and
represent the sensitivity of the production and degradation of constituent i to the secondary proteolytic activity (due to hypoxia), respectively.



(5)

(6)




Since the number of inflammatory cells reduces linearly with the ILT thickness in the luminal side of the ILT [1], we assume that C p also is reduced linearly in luminal layer such that it vanishes in depth of h 2 (see Fig. 1). Furthermore, assume that each ILT layer is made of a homogeneous material and
in the ILT changes linearly in the depth of ILT but the rate of change differs in each layer due to different permeabilities of the ILT layers. We assume the same material properties for the medial and abluminal layers. As a result, the changes of
versus the ILT depth takes the multi-line shape depicted in Fig. 1c regarding less permeability in the luminal layer due to its dense cross linked fibrillar matrix [1, 11, 19, 24, 25].
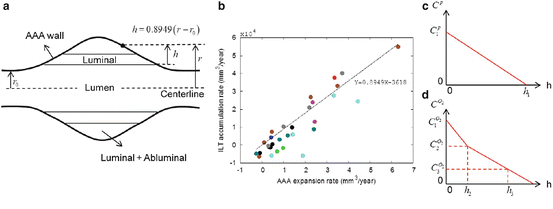


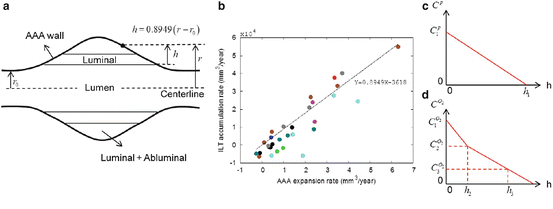
Fig. 1
(a) Schematic view of an AAA including ILT. (b) The plot for the correlation between the ILT accumulation rate and the rate of AAA expansion. These rates were defined as the changes of volumes between two consecutive scans over that time interval. Each color refers to longitudinal CT data of a different patient [33]. (c) The changes of active proteolytic enzymes in the ILT’s depth. (d) The changes of oxygen concentration in the ILT’s depth
Furthermore, the thickness of ILT at each point on the AAA wall is estimated based on the recent clinical study performed by Zambrano et al. [33] on studying the rate of ILT formation as a function of the AAA geometry from longitudinal CT studies of patients. They showed by calculating the changes of ILT and AAA outer volumes between two consecutive scans over time that there is a positive correlation between the ILT accumulation rate and the AAA expansion rate with α = 0. 8949, where α is the slope of the trendline (see Fig. 1b). Accordingly, for an axisymmetric model of AAA, the thickness of the ILT (h) at each point on the AAA wall located in the radial distance r is estimated by
, where r 0 is the initial radius of the (healthy) aorta. The latter equation is easily obtained by assuming that the stretch of the AAA wall in the axial direction is relatively negligible compared to the changes of the AAA radius r.
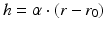
The values of the parameters used in the G&R model along with the reference they are taken from are summarized in Table 1.
![]() | ![]() | ![]() | ![]() | ![]() | ![]() | h 1 | h 2 | h 3 | K σ i | κ b i
![]() Stay updated, free articles. Join our Telegram channel![]() Full access? Get Clinical Tree![]() ![]() ![]() |
---|