Glaucoma
KEY CONCEPTS
Primary open-angle glaucoma (POAG) or ocular hypertension is more prevalent outside Asia than closed- or narrow-angle glaucoma.
In any form of glaucoma, reduction of intraocular pressure (IOP) is essential.
IOP is a very important risk factor for glaucoma, but the most important considerations are progression of glaucomatous changes in the back of the eye (optic disk and nerve fiber layer) and visual field changes when diagnosing and monitoring for POAG or ocular hypertension.
Optic nerve changes often occur before visual field changes are exhibited.
Recent studies demonstrate that reduction in IOP prevents progression or even onset of glaucoma.
Newer medications simplify treatment regimens for patients. Prostaglandin analogs are considered the most potent topical medications for reducing IOP and flattening diurnal variations in IOP.
Local adverse events are common with topical glaucoma medications, but patient education and reinforcing adherence are essential to prevent glaucoma progression.
The glaucomas are a group of ocular disorders that lead to an optic neuropathy characterized by changes in the optic nerve head (optic disk) that is associated with loss of visual sensitivity and field. Increased intraocular pressure (IOP), a traditional diagnostic criterion for glaucoma, is thought to play an important role in the pathogenesis of glaucoma, but it is no longer a diagnostic criterion for glaucoma.1–10 Two major types of glaucoma have been identified: open angle and closed angle. Open-angle glaucoma accounts for the great majority of cases in North America, while closed-angle glaucoma (CAG) is more prevalent in Asia. Either type can be a primary inherited disorder, congenital, or secondary to disease, trauma, or drugs and can lead to serious complications.1,11–16 Both primary and secondary glaucomas may be caused by a combination of open-angle and closed-angle mechanisms (Table 75–1).
TABLE 75-1 General Classification of Glaucoma
BASIC CONCEPTS
Aqueous Humor Dynamics and Intraocular Pressure
An understanding of IOP and aqueous humor dynamics will assist the reader in understanding the drug therapy of glaucoma.1,2,17–19
Aqueous humor is formed in the ciliary body and its epithelium (Figs. 75-1 and 75-2) through both filtration and secretion. Because ultrafiltration depends on pressure gradients, blood pressure and IOP changes influence aqueous humor formation. Osmotic gradients produced by active secretion of sodium and bicarbonate and possibly by other solutes such as ascorbate from the ciliary body epithelial cells into the aqueous humor result in movement of water from the pool of ciliary stromal ultrafiltrate into the posterior chamber, forming aqueous humor. Carbonic anhydrase (primarily isoenzyme type II), α– and β-adrenergic receptors, and sodium- and potassium-activated adenosine triphosphatases are found on the ciliary epithelium and appear to be involved in this secretion of the solutes sodium and bicarbonate.
FIGURE 75-1 Anatomy of the eye.
FIGURE 75-2 Anterior chamber of the eye and aqueous humor flow.
Receptor systems controlling aqueous inflow have not been elucidated fully. Pharmacologic studies suggest that β-adrenergic agents increase inflow, whereas α2-adrenergic blocking, β-adrenergic blocking dopamine-blocking, carbonic anhydrase-inhibiting, and adenylate cyclase-stimulating agents decrease aqueous inflow. Aqueous humor produced by the ciliary body is secreted into the posterior chamber at a rate of approximately 2 to 3 μL/min. The pressure in the posterior chamber produced by the constant inflow pushes the aqueous humor between the iris and lens and through the pupil into the anterior chamber of the eye (see Fig. 75–2).1,2,17–22
Aqueous humor in the anterior chamber leaves the eye by two routes: (a) filtration through the trabecular meshwork (conventional outflow) to the Schlemm’s canal (80% to 85%) and (b) through the ciliary body and the suprachoroidal space (uveoscleral outflow or unconventional outflow). Cholinergic agents such as pilocarpine increase outflow by physically opening the meshwork pores secondary to ciliary muscle contraction. The uveoscleral outflow of aqueous humor is also increased by prostaglandin analogs and β– and α2-adrenergic agonists. Constant inflow of aqueous humor from the ciliary body and resistance to outflow result in an IOP great enough to produce an outflow rate equal to the inflow rate (see Fig. 75–2).
The median IOP measured in large populations is 15.5 ± 2.5 mm Hg (2.1 ± 0.3 kPa); however, the distribution of pressures around the mean is skewed to the right (toward higher readings). IOP is not constant and changes with pulse, blood pressure, forced expiration or coughing, neck compression, and posture. The amount of caffeine in 1 cup of caffeinated coffee (182 mg) increases IOP by about 1 mm Hg after 90 minutes in one study. The authors concluded that the impact of this increase in IOP was not clinically relevant.9 IOP is measured by tonometry: indentation tonometry, applanation tonometry, or a noncontact method using an air pulse. Newer methods of tonometery include the Pascal tonometer, Icare™ rebound tonometer, and a contact lens-based investigational device that can remotely monitor 24-hour IOP changes from baseline.10–12 These methods may result in slightly different pressure readings. IOPs consistently greater than 21 mm Hg (2.8 kPa) are found in 5% to 8% of the general population. The incidence increases with age, such that “abnormal” (i.e., >22 mm Hg [>2.9 kPa]) IOP is found in 15% of those 70 to 75 years of age. Intermittently high IOP (>40 mm Hg [>5.3 kPa]) is found in patients with CAG. The increased IOP in all types of glaucoma results from the decreased facility for aqueous humor outflow through the trabecular meshwork. Aqueous humor production in primary open-angle glaucoma (POAG) is normal.1,2,17–19
IOP demonstrates considerable circadian variation (often referred to as diurnal IOP or the IOP during the daily 24-hour cycle) primarily because of changes in the rate of aqueous humor formation. This circadian variation results in a minimum IOP at approximately 6 PM and a maximum IOP at awakening, although some studies suggest that both healthy and glaucoma patients may have their highest IOP at night after falling asleep.20 Low systemic blood pressure in conjunction with high IOPs (decreased ocular perfusion pressure) at night can result in optic nerve head damage.20 Generally, the circadian IOP variation is usually less than 3 to 4 mm Hg (0.4 to 0.5 kPa); however, it may be greater for patients with glaucoma. This circadian variation and the poor relationship of IOP with visual loss make measurement of IOP a poor screening test for glaucoma.
Although increased IOP within any range is associated with a higher risk of glaucomatous damage, it is both an insensitive and nonspecific diagnostic and monitoring tool. Of individuals with IOP between 21 and 30 mm Hg (2.8 and 4.0 kPa), only 0.5% to 1% per year will develop optic disk changes and visual field loss (i.e., glaucoma) over 5 to 15 years. However, more subtle retinal damage, such as alteration of color vision or decreased contrast sensitivity, occurs in a higher percentage of patients with IOPs greater than 21 mm Hg (2.8 kPa), and the incidence of visual field defects increases to as high as 28% in individuals with IOPs above 30 mm Hg (4.0 kPa). For a given abnormal IOP, the incidence of glaucoma increases with age. For patients with preexisting optic nerve damage, the worse the existing damage, the more sensitive the eye is to a given IOP. As many as 20% to 30% of patients with glaucomatous visual field loss have an IOP of less than 21 mm Hg (2.8 kPa) (called normal-tension glaucoma, referring to the normal IOP). Thus the absolute IOP is a less-precise predictor of optic nerve damage. More direct measurements of therapeutic outcome, such as optic disk examination and visual field evaluation, also must be used as monitors of disease progression.1–7,17–24 Taking the above factors into consideration, glaucoma medications that provide maximal reduction of IOP over 24 hours and have minimal influence on blood pressure may be advantageous in treating glaucoma patients.
Optic Disk and Visual Fields
The optic disk is the portion of the optic nerve ophthalmoscopically visible as it leaves the eye. It consists of approximately 1 million retinal ganglion nerve cell axons, blood vessels, and supporting connective tissue structures (lamina cribrosa). The small depression within the disk is termed the cup (Fig. 75–3). A normal physiologic cup does not extend beyond the optic nerve rim and has a varying diameter of less than one third to one half that of the disk (cup-to-disk ratio: 0.33 to 0.5). Table 75–2 lists the common alterations of the optic disk found in glaucoma. These disk changes result from optic nerve axonal degeneration and remodeling of the supporting structures. As the nerve axons die, the cup becomes larger in relation to the whole disk. A loss of retinal nerve fiber layer might be visualized in glaucoma patients with detectable visual field loss. This pattern of changes is consistent with visual field losses and loss of visual sensitivity seen in glaucoma.1,2,17–24 Damage to the optic nerve can be documented by optic disk photographs, and disease stability or progression may be monitored by examining sequential photographs. Newer methods of assessing damage to the retinal nerve fiber layer and optic disk have been described. These include scanning laser polarimetry (GDX), confocal laser ophthalmoscopy (Heidelberg retinal tomography, or HRT), and optical coherence tomography (OCT). These methods offer the ability to assess the damage to the optic nerve quantitatively.
FIGURE 75-3 Normal fundus of the eye and optic disk and cup.
TABLE 75-2 Optic Disk and Visual Field Findings
Determination of the visual field allows assessment of optic nerve damage and is an important monitoring parameter in treatment. However, visual field changes lag behind optic disk changes, and a loss of 25% to 35% of retinal ganglion cells is usually required before detectable visual field defects are noted. The peripheral visual field is measured using a visual field instrument called a perimeter. Characteristic visual field loss occurs in glaucoma (Fig. 75–4; see also Table 75–2), but loss of central visual acuity usually does not occur until late in the disease. Other indicators, such as color vision changes and contrast sensitivity, may allow earlier and more sensitive detection of glaucomatous changes.1,2
FIGURE 75-4 Schematic of the progression of visual field loss in glaucoma.
Genetics
Glaucoma is often inherited as a complex multifactorial disease, but it can also be inherited as a Mendelian autosomal-dominant or autosomal-recessive trait form. The common age-related adult-onset glaucoma, like POAG, although containing heritability of some significance, is more complex and is influenced by environmental factors. Genetic studies have more clearly defined the underlying molecular events responsible for the Mendelian forms of the disease. However, the chromosome locations identified may play some factor in the more complex forms. A number of major gene loci associated with POAG have been identified. The molecular mechanism of how mutations in any of these genes result in increased IOP with loss of visual field has not been elucidated. The future of genetic studies in glaucoma will include discovery of new glaucoma genes, determination of clinical phenotypes associated with these genes and mutations, understanding how environmental factors interact, and developing a database that can be used for further testing.
Genome-wide association studies have identified new loci that are associated with clinically relevant optic disc parameters, including the optic disc area and vertical cup–disc ratio.15 Genes associated with chronic angle closure glaucoma have also been identified.16 Improved understanding of the genetic origins of POAG may lead to new diagnostic tools and therapies that target the underlying causes of the disease.1,2,15,16,25,26
Epidemiology of Open-Angle Glaucoma
Open-angle glaucoma (OAG) is the second leading cause of blindness, affecting up to 3 million individuals in the United States and up to 60.5 million individuals worldwide. It is estimated that more than 135,000 persons in the United States and about 4.5 million in the world have glaucoma-related bilateral blindness. The prevalence rate varies with age, race, diagnostic criteria, and other factors. In the United States, OAG occurs in 1.5% of the population older than 30 years of age, 1.3% of whites and 3.5% of blacks. Recent study data have also suggested that the prevalence of OAG and ocular hypertension is also high among Latinos of Mexican ancestry, with approximately 4.74% and 3.56% of people affected, respectively.27 The incidence of OAG increases with increasing age. The incidence of the disease for patients 80 years of age is 3% in whites and 5% to 8% in blacks.
Etiology of Open-Angle Glaucoma
The specific cause of glaucomatous optic neuropathy is presently unknown. Previously, increased IOP was considered to be the sole cause of the damage; however, it is now recognized that IOP is only one of many factors associated with the development and progression of glaucoma.1–10 Increased susceptibility of the optic nerve to ischemia (a reduced or dysregulated blood flow), excitotoxicity, autoimmune reactions, and other abnormal physiologic processes are likely additional contributory factors. The final outcome of these processes is believed to be apoptosis of the retinal ganglion cells, which results in axonal degeneration and finally permanent loss of vision.11–16 Interestingly enough, there appears to be a fair amount of similarity between neuronal cell death by apoptosis in Alzheimer’s disease and glaucoma.13 Indeed, POAG may represent a number of distinct diseases or conditions that simply manifest the same symptoms. Susceptibility to visual loss at a given IOP varies considerably; some patients do not demonstrate damage at high IOPs, whereas other patients have progressive visual field loss despite an IOP in the normal range (normal-tension glaucoma).
Although IOP poorly predicts which patients will have visual field loss, the risk of visual field loss clearly increases with increasing IOP within any range. In fact, recent studies demonstrate that lowering IOP, no matter what the pretreatment IOP, reduces the risk of glaucomatous progression or may even prevent the onset to early glaucoma in patients with ocular hypertension.3–7
The mechanism by which a certain level of IOP increases the susceptibility of a given eye to nerve damage remains controversial. Multiple mechanisms are likely to be operative in a spectrum of combinations to produce the death of retinal ganglion cells and their axons in glaucoma. Pressure-sensitive astrocytes and other cells in the optic disk supportive matrix may produce changes and remodeling of the disk, resulting in axonal death. Vasogenic theories suggest that optic nerve damage results from insufficient blood flow to the retina secondary to the increased perfusion pressure required in the eye, dysregulated perfusion, or vessel wall abnormalities, and results in degeneration of axonal fibers of the retina. Another theory suggests that the IOP may disrupt axoplasmal flow at the optic disk.
Recently, focus on the mechanisms of the retinal ganglion cell apoptosis and the role of excessive glutamate and nitric oxide found in glaucoma patients has broadened the focus of drug therapy research to include evaluation of agents that act as neuroprotectants.12–15 Such agents may be particularly useful for patients with normal-pressure glaucoma, in whom pressure-independent factors may play a relatively larger role in disease progression. These agents would target risk factors and underlying pathophysiologic mechanisms of disease other than IOP.11–16
Pathophysiology of Open-Angle Glaucoma
As stated previously, optic nerve damage in POAG can occur at a wide range of IOPs, and the rate of progression is highly variable. Patients may exhibit pressures in the 20 to 30 mm Hg (2.7 to 4.0 kPa) range for years before any disease progression is noticed in the optic disk or visual fields. That is why POAG is often referred to as the “sneak thief of sight.”
CLINICAL PRESENTATION Glaucoma
Clinical Presentation of Open-Angle Glaucoma
POAG is a bilateral, often asymmetric, genetically determined disorder constituting 60% to 70% of all glaucomas and 90% to 95% of primary glaucomas (see Clinical Presentation of Glaucoma above). An increased IOP is not required for diagnosis of POAG. Symptoms do not present until substantial visual field constriction occurs. Central visual acuity typically is maintained, even in the late stages of the disease. Even though POAG is a bilateral disease, it may have greater IOP and progression and severity in one eye. As such, each eye is treated individually.
Detection and diagnosis involve evaluation of the optic disk and retinal nerve fiber layer, assessment of the visual fields, and measurement of IOP. The presence of characteristic disk changes and visual field loss with or without increased IOP confirms the diagnosis of glaucoma. Typical disk changes and field loss occurring at an IOP of less than 21 mm Hg (2.8 kPa) account for 20% to 30% of patients and are referred to as normal-tension glaucoma. Elevated IOP (>21 mm Hg [>2.8 kPa]) without disk changes or visual field loss is observed in 5% to 7% of individuals (known as glaucoma suspects) and is referred to as ocular hypertension. New technologies such as OCT, retinal nerve fiber analyzers, or confocal scanning laser tomography of the optic nerve head may allow early identification of signs of glaucomatous retinal changes in ocular hypertensives, thus allowing for earlier initiation of therapy.1–3,17
Secondary OAG has many causes, including exfoliation syndrome, pigmentary glaucoma, systemic diseases, trauma, surgery, ocular inflammatory diseases, and medications. A system for classifying secondary glaucomas into pretrabecular, trabecular, and posttrabecular forms has been proposed. This classification allows drug therapy to be chosen on the basis of the pathogenic mechanism involved. In pretrabecular forms, a normal meshwork is covered and does not permit aqueous humor outflow. Trabecular forms of secondary glaucoma result from either an alteration of meshwork or an accumulation of material in the intertrabecular spaces. The posttrabecular forms result primarily from disorders causing increased episcleral venous blood pressure.1,2,15–17
Prognosis of Open Angle Glaucoma
In most cases of POAG, the overall prognosis is excellent when it is discovered early and treated adequately. Even patients with advanced visual field loss can have continued visual field loss reduced if the IOP is maintained at low enough pressures (often <10 to 12 mm Hg [<1.3 to 1.6 kPa]). Progression of visual field loss still occurs in 8% to 20% of patients despite reaching standard therapy IOP goals. However, for untreated patients and for those who fail to achieve target IOP reduction, up to 80% have continued visual field loss. Estimates of progression to bilateral blindness in treated patients range from 4% to 22%. Thus, the keys to medical treatment of POAG are an effective, well-tolerated drug regimen, close monitoring of therapy, and adherence. Medications will control IOP successfully in 60% to 80% of patients over a 5-year period. Availability of newer, highly effective, well-tolerated agents may improve the prognosis further.1,2,5,17–19,23–28
Epidemiology of Closed-Angle Glaucoma
The incidence of CAG varies by the ethnic group, with a higher incidence in individuals of Inuit, Chinese, and Asian-Indian descent. Incidence rates of 1% to 4% have been reported in these populations.1,2 Because of the high frequency of CAG in populous Asia, CAG accounts for approximately one-third of glaucoma worldwide. CAG accounts for a disproportionately high proportion of blindness (estimated at up to 50%) worldwide.1
Etiology of Closed-Angle Glaucoma (Angle-Closure Glaucoma)
In North America, primary CAG accounts for a minority of primary glaucomas. When severe acute CAG occurs, it may need to be treated as an emergency to avoid visual loss.
CAG results from mechanical blockage of the (usually normal) trabecular meshwork by the peripheral iris. Partial or complete blockage of the meshwork occurs intermittently, potentially resulting in extreme fluctuations between normal IOP with no symptoms and very high IOP with symptoms of acute CAG. Between attacks of CAG, the IOP is usually normal unless the patient has concomitant POAG or nonreversible blockage of the meshwork with synechiae (“creeping” angle closure) that develops over time in the narrow-angle eye. Primary CAG occurs for patients with inherited shallow anterior chambers (often seen in small eyes), which produce a narrow angle between the cornea and iris or tight contact between the iris and lens (pupillary block). The presence of a narrow angle is determined mainly by visualization of the angle by gonioscopy. Other tests for CAG involve provocation of an angle-closure–induced IOP increase. These tests, which attempt to produce angle closure through mydriasis (darkroom test or mydriasis test) or gravity (prone test), are rarely performed in the clinical setting.
Two major types of classic, reversible primary CAG have been described: CAG with pupillary block and CAG without pupillary block. CAG with pupillary block results when the iris is in firm contact with the lens. This produces a relative block of aqueous flow through the pupil to the anterior chamber (pupillary block), resulting in a bowing forward of the iris, which blocks the trabecular meshwork. CAG with pupillary block occurs most commonly when the pupil is in middilation. In this position, the combination of pupillary block and relaxed iris allows the greatest bowing of the iris; however, angle closure may occur during miosis or mydriasis.
CAG can occur without significant pupillary block for patients with an abnormality called a plateau iris. The ciliary processes in these cases are situated anteriorly, which indent the iris forward and cause closure of the trabecular meshwork, especially during mydriasis. The mydriasis produced by anticholinergic drugs or any other drug results in precipitation of both types of CAG glaucoma, whereas drug-induced miosis may produce pupillary block.
Pathophysiology of Closed-Angle Glaucoma
The mechanism of IOP elevation in CAG is clearer than that of POAG. In CAG, a physical blockage of trabecular meshwork is present. In many cases, single or multiple episodes of high IOP that in some patients may exceed 40 mm Hg [>5.3 kPa]) and result in optic nerve damage. Very high IOP (>60 mm Hg [>8.0 kPa]) may result in permanent loss of visual field within a matter of hours to days.
One type of CAG, known as “creeping” angle closure, occurs in patients with narrow angles in which the iris adheres to the trabecular meshwork and may result in continuously increased IOP in ranges more similar to those of POAG, and the clinical behavior is similar to POAG, with individuals differing in the degree and rapidity of visual loss from any given elevated IOP.1
Clinical Presentation of Closed-Angle Glaucoma
Most patients with untreated CAG typically experience intermittent nonsymptomatic or prodromal symptoms brought on by precipitating events (see Clinical Presentation of Glaucoma above). Increased IOP during such prodromal episodes is not great enough or long enough to produce the other symptoms of a full-blown attack. Such prodromal attacks last 1 to 2 hours, at which time pupillary block is broken by further mydriasis or miosis, or when miosis or mydriasis occurs in patients with plateau iris. The rate at which IOP increases may be a determinant of when full-blown symptoms occur. Visual fields demonstrate generalized constriction or typical glaucomatous defects as seen in POAG. In approximately 25% of patients, severe attacks may occur and if prolonged, total loss of vision may occur if the IOP is high enough. Tonometry reveals IOPs as high as 40 to 90 mm Hg (5.3 to 12.0 kPa). Patients who have developed adhesions between the iris and meshwork (anterior synechiae) may have chronic IOP elevation with intermittent spikes of high IOP when angle closure occurs.
Drug-Induced Glaucoma
A number of medications are associated with increased IOP or carry labeling that cautions against use of the medication in glaucoma patients. The potential for a medication to produce or worsen glaucoma depends on the type of glaucoma and whether the patient is treated adequately.25
Patients with treated, controlled POAG are at minimal risk of induction of an increase in IOP by systemic medications with anticholinergic properties or vasodilators; however, for patients with untreated glaucoma or uncontrolled POAG, the potential of these medications to increase IOP should be considered. Topical anticholinergic agents used to produce mydriasis may result in an increase in IOP. Potent anticholinergic agents such as atropine or homatropine are most likely to increase IOP. Weaker anticholinergics, such as tropicamide, that produce less cycloplegia are less likely to increase IOP and are favored, along with phenylephrine, when mydriasis is desired for POAG patients. Inhaled, nasal, topical, or systemic glucocorticoids may increase IOP for both normal individuals and patients with POAG.
Patients with POAG appear to be particularly susceptible to glucocorticoid-induced increases in IOP. Glucocorticoids reduce the facility of aqueous humor outflow through the trabecular meshwork. The decreased facility of outflow appears to result from the accumulation of extracellular material blocking the trabecular channels. The potential of a glucocorticoid to increase IOP is related to its antiinflammatory potency and intraocular penetration. Thus, patients should be treated with the lowest potency and dose and for the shortest time possible when steroids are indicated.
For patients predisposed to CAG (i.e., narrow anterior chambers), angle closure may be produced by any drug that causes mydriasis (e.g., anticholinergics). A wide range of sulfa compounds causes idiosyncratic reactions that result in anterior choroidal effusions with anterior movement of the iris and lens, resulting in angle closure. The topical use of anticholinergics or sympathomimetic agents most likely will result in angle closure. Systemic and inhaled anticholinergic and sympathomimetic agents also must be used with caution in such patients. As discussed previously, potent miotic agents such as echothiophate may produce angle closure by increasing pupillary block. Table 75–3 lists the drugs associated with potentiation of glaucoma.
TABLE 75-3 Drugs That May Induce or Potentiate Increased Intraocular Pressure
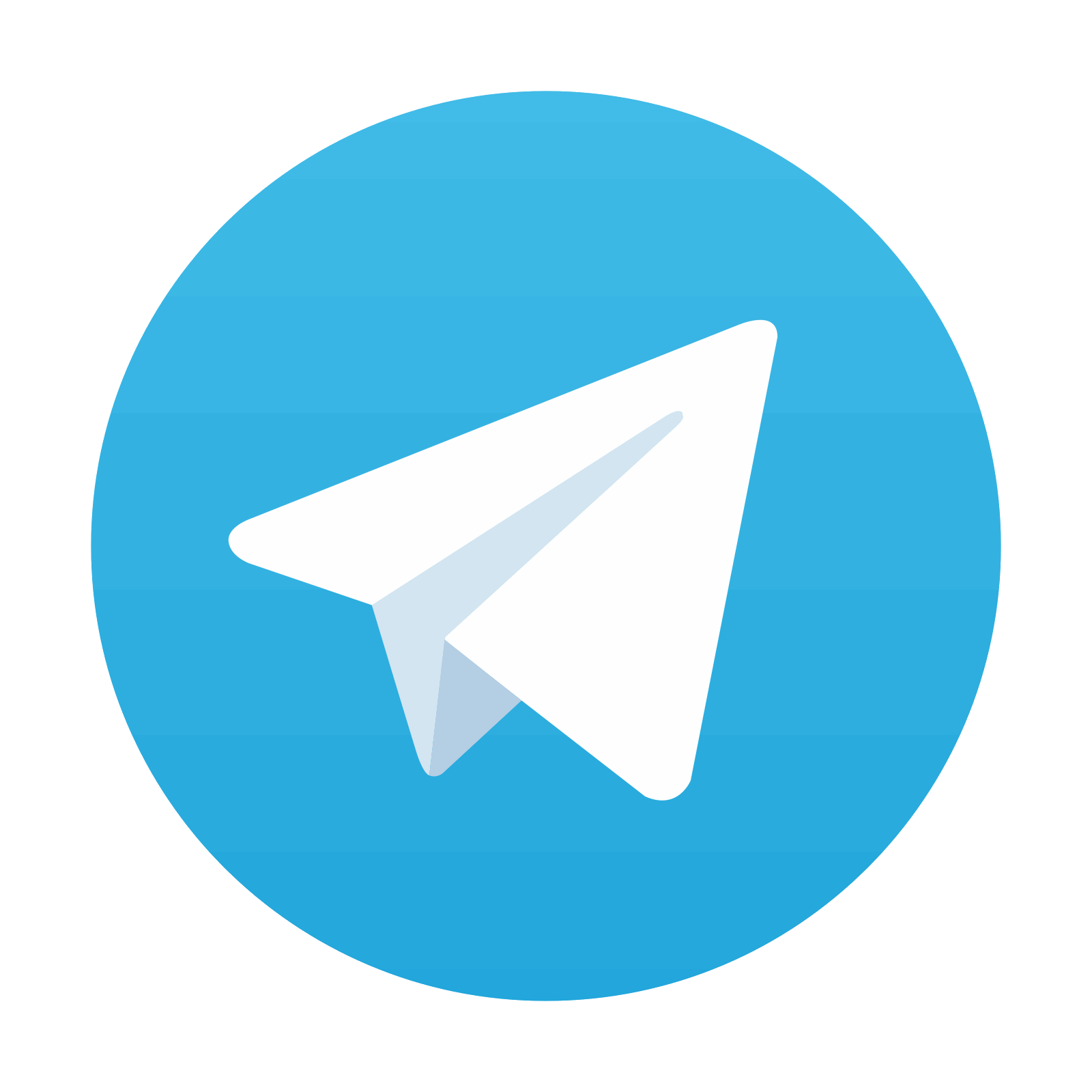