Genetics and Deoxyribonucleic Acid-Based Technology in Clinical Biochemistry
Genetic disorders fall into three main categories:
Chromosomal disorders due to the absence, or abnormal arrangement, of chromosomes affecting many genes and therefore many gene products. Examples include Down’s syndrome (trisomy for chromosome 21), Turner’s syndrome (45,XO) and Klinefelter’s syndrome (47,XXY).
Monogenic disorders due to an abnormality of a single gene, which is the primary determinant of the disorder and which is inherited in a predictable pattern, such as phenylketonuria.
Multifactorial or polygenic disorders due to the interaction of multiple genes with environmental or other exogenous factors, such as diabetes mellitus.
GENERAL PRINCIPLES
Genes, located on chromosomes, comprise a sequence of bases on deoxyribonucleic acid (DNA) and code for the synthesis of proteins by ribonucleic acid (RNA) in its ‘messenger’ form (mRNA). All nucleated cells contain an identical complement of genes, but only about 1 per cent is expressed.
A genotype is the actual genes present in an individual; phenotype is the physical expression of that genotype, usually via production of a polypeptide or protein. Genes differ in length, sometimes containing many thousands of bases. However, only 10 per cent of the genome incorporates the protein-coding sequences (exons) of genes. Interspersed within many genes are intron sequences, which have no coding function.
The rest of the genome consists of other non-coding regions (such as control sequences and intergenic regions). Humans can synthesize about 100 000 varying polypeptides or proteins, which are usually composed of about 20 different kinds of amino acids. Genes contain specific sequences of three DNA bases (codons) instructing the cell’s protein-synthesizing apparatus to add specific amino acids.
In disorders of single genes (monogenic), the abnormalities may be of:
a structural gene, with production of an abnormal protein: in this case all the biochemical abnormalities can be explained by defective synthesis of a single peptide;
a controlling (enhancing) gene, which, by altering the rate at which one or more structural genes function, affects the amounts of one or more structurally normal peptides.
The affected protein may be an enzyme, but in other conditions it may, for example, be a receptor, a transport or a structural protein, a peptide hormone, an immunoglobulin or a coagulation factor. With the Human Genome Project, more and more genes and their functions are being discovered.
PATTERNS OF INHERITANCE
Every inherited characteristic is governed by a pair of genes on homologous chromosomes, one gene being received from each parent. Different genes governing the same characteristic are called alleles. An individual with two identical alleles is homozygous for that gene or inherited characteristic; an individual with two different alleles is heterozygous. Genes may be carried on the autosomes (similar in both sexes) or on the sex chromosomes (X and Y); the patterns of inheritance differ.
Autosomal inheritance (Fig. 28.1)
If one parent (Parent 1 in the example in Fig. 28.1a) is heterozygous for an abnormal gene (A) and the other parent is homozygous for the normal gene (N), the possible combinations in the offspring are shown in the square in this figure.
On a statistical basis, half the offspring will be heterozygous (AN) for gene A, like Parent 1. None will be homozygous for the abnormal gene (AA).
If both parents are heterozygous (AN; Fig. 28.1b), a quarter of the offspring will be homozygous (AA) and half will be heterozygous (AN).
If one parent is homozygous (AA) and the other normal (NN), all the offspring will be heterozygous.
The metabolic consequences of an abnormal gene depend on the effectiveness of that gene compared with the normal one.
Autosomal dominant inheritance
Dominant abnormal genes affect both heterozygotes (AN) and homozygotes (AA), although homozygotes may be more severely affected. In the first example, Parent 1 (AN) and half the offspring will be affected, and in the second example both parents and three out of four of the offspring (AA and AN) will be affected. Characteristically, if there is autosomal dominant inheritance:
every affected individual has at least one affected parent,
offspring in successive generations are affected,
clinically normal offspring are not carriers of the abnormal gene,
statistically, three in four children are affected if both parents are heterozygous – if one parent is heterozygous, there is a one in two chance of an offspring being the same.
An example is familial hypercholesterolaemia (Chapter 13).
Autosomal recessive inheritance
Recessive abnormal genes only affect homozygous offspring (AA). In the first example, neither parent nor the offspring will be affected, and in the second example both parents will appear normal, but statistically one in four of the offspring will be affected. Therefore, in autosomal recessive inheritance:
heterozygous parents are not usually clinically affected,
clinical consequences may miss offspring in succeeding generations,
clinically normal children may be heterozygous and therefore may be carriers of the abnormal gene,
on average, one in four children of heterozygous parents is clinically affected.
Disorders inherited as a recessive trait have a lower expression frequency in affected families than dominant disorders, but tend to be more severe. An example is cystic fibrosis (Chapter 16).
Sex-linked inheritance
Some abnormal genes are carried only on the sex (almost always the X) chromosomes.
X-linked recessive inheritance
Women have two X chromosomes and men have one X and one Y chromosome. In X-linked recessive
inheritance, an abnormal X chromosome (Xa) is latent when combined with a normal X chromosome, but active when combined with a Y chromosome. If the mother carries Xa, she will appear to be normal, but statistically half her sons will be affected (XaY). Half her daughters will be carriers (XaX), but all her daughters will be clinically unaffected (Fig. 28.1c).
inheritance, an abnormal X chromosome (Xa) is latent when combined with a normal X chromosome, but active when combined with a Y chromosome. If the mother carries Xa, she will appear to be normal, but statistically half her sons will be affected (XaY). Half her daughters will be carriers (XaX), but all her daughters will be clinically unaffected (Fig. 28.1c).
If the father is affected and the mother carries two normal genes, none of the sons will be affected, but all the daughters will be carriers (Fig. 28.1d).
Inherited disease manifesting in male offspring and carried by females is typical of X-linked inheritance. Females are only clinically affected in the extremely rare circumstance when they are homozygous for the abnormal gene. This will occur only if the female inherited the abnormal genes from an affected father and a carrier mother. Haemophilia is the classic example of an X-linked recessive disorder.
X-linked dominant inheritance
In this type of very rare inheritance, both XaX women and XaY men are affected. An example of this very rare type of disorder is familial hypophosphataemia.
Multiple alleles
Occasionally there may be several alleles governing the same characteristic. In such cases, different pair combinations may produce different disease patterns, for example some of the haemoglobinopathies, or the variant may be detectable only by biochemical testing, such as, for example, some of the plasma protein variants.
In rare cases, spontaneous new mutations may occur and may produce dominant disorders in unaffected families. The terms ‘dominant’ and ‘recessive’ are relative. A dominant gene may fail to manifest itself (incomplete penetrance) and may therefore appear to skip a generation. A gene may vary in its degree of expression, and therefore in the degree of abnormality that it produces. A recessive gene, which produces disease only in homozygotes (AA), may be detectable by laboratory tests in clinically unaffected heterozygotes.
GENETIC DIAGNOSIS
In recent years there has been a huge growth in DNA technology and research, such that clinical diagnosis can now be made in certain conditions using small tissue samples. This chapter gives a brief outline of such technology; for a more detailed exposition, the reader is advised to refer to a specific molecular biology textbook. However, in some disorders, especially those with multiple polymorphisms or mutations or with incomplete penetrance, a biochemical or phenotypic diagnosis is still preferable. Epigenetics is the inheritance of a characteristic not by a change in DNA sequence, such as may occur by histone acetylation or DNA methylation, which modify gene expression. See Wenham PR. DNA-based techniques in clinical biochemistry: a beginner’s guide to theory and practice. Ann Clin Biochem 1992; 29: 598-624.
Basics principles of molecular biology
Chromosomes consist of both protein and DNA; chromosomal DNA contains an average of 100-200 million bases. DNA is a nucleoside polymer arranged in two strands as a double helix. There are four bases in DNA, namely adenine (A), cytosine (C), guanine (G) and thymine (T). These nucleotides consist of ester-linked phosphate residues at the 5′-hydroxyl residue of a deoxyribose molecule that itself is linked to a purine or pyrimidine base by its 1′-hydroxyl group. Each of the DNA strands has polarity, namely 5′ and 3′ ends with the two opposite-running strands in opposite directions (anti-parallel).
The two DNA strands are complementary, so that C on one strand bonds by hydrogen bonds with G on another strand and A pairs with T. These bases are always numbered from the 5′ to the 3′, with letters indicating the nucleotide bases. Genetic material can copy itself (replication) during the S phase of the cell cycle. DNA polymerase needs a pre-existing DNA template and moves along the two strands and adds the appropriate nucleotides, following the base pairing rules, to the growing chain. Two new double strands of DNA are formed, identical to the original double strand and the strands are anti-parallel. DNA polymerase always moves from 3′ to 5′ along the template strand, i.e. the reverse of the newly forming strand. Variable number tandem repeats (VNTR) are short and repetitive repeats distributed throughout the genome and that are grouped near telomeres.
Transcription is the process whereby the polypeptide or protein-coding instructions from the genes are transcribed indirectly through mRNA; the latter moves from the nucleus to the cellular cytoplasm, serving as the template for protein synthesis. Translation is the process whereby the ribosomal system then translates the codons into an amino acid chain that will constitute the polypeptide or protein molecule for which it codes. A gene is a unique sequence of nucleotides that codes for
a particular protein or peptide and can be represented by one or more alleles. The genetic code is thus a series of codons that instruct which amino acids are needed to synthesize specific polypeptides or proteins.
a particular protein or peptide and can be represented by one or more alleles. The genetic code is thus a series of codons that instruct which amino acids are needed to synthesize specific polypeptides or proteins.
Deoxyribonucleic acid technologies
Deoxyribonucleic acid can easily be prepared from very small amounts of biological samples, often blood. This essentially involves three steps: leucocyte lysis, chloroform/phenol extraction to remove contamination by proteins, followed by proteinase and RNAase treatment to remove remaining protein and RNA contamination.
Samples of DNA after digestion are separated by electrophoresis due to its strong negative charge at neutral pH and, by running the DNA through agarose gels, it can be separated into discrete DNA molecules of 100-10 000 base pairs. Smaller DNA pieces can be separated by polyacrylamide gels, which are a tighter molecular sieve than agarose. DNA probes can be a piece of DNA of variable length that can be inserted into the DNA of a plasmid. These circular pieces of double-stranded DNA can be artificially introduced into bacteria and incorporated into their DNA by a process called transformation. By adding divalent cations the bacteria are made permeable to DNA and a selectable marker such as antibiotic resistance is then inserted, and only those bacteria containing this will grow in media containing the antibiotic. The probes can be labelled to locate them in DNA experiments, using either radiolabelled phosphorus-32 or, for example, non-radiolabelled horseradish peroxidase-enhanced chemiluminescence or digoxigenin.
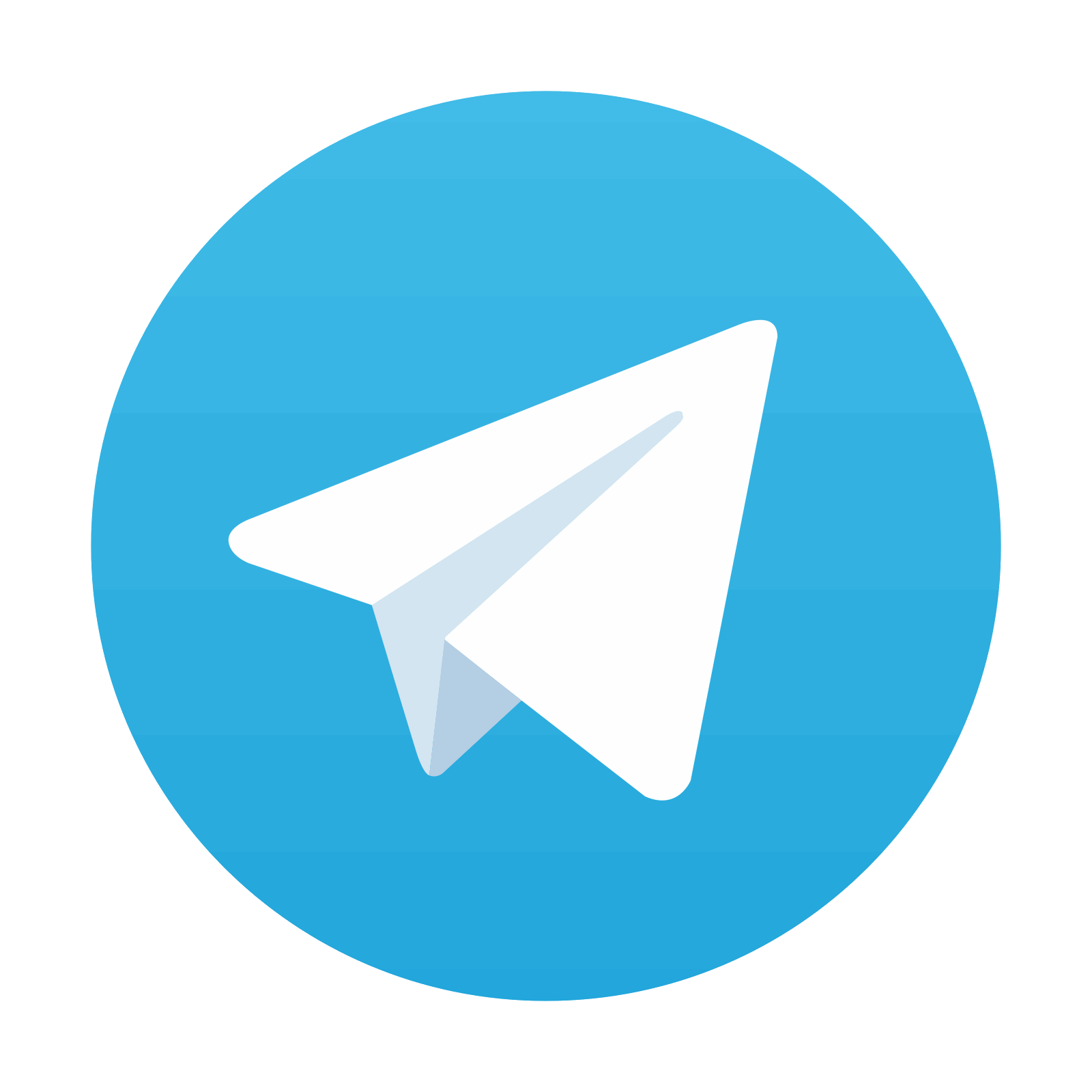
Stay updated, free articles. Join our Telegram channel

Full access? Get Clinical Tree
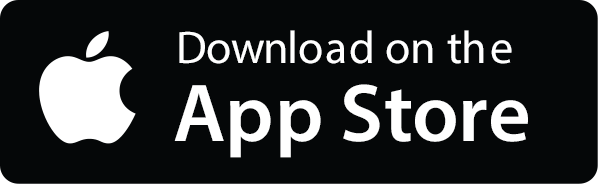
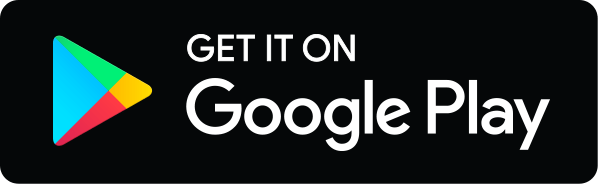