8 General Neuroanatomy
8.1 Development of the Central Nervous System (CNS)

A Development of the brain
a Embryo with a greatest length (GL, see p. 4) of 10 mm, at the beginning of the 2nd month of development. Even at this stage we can see the differentiation of the neural tube into segments that will generate various brain regions (see C):
• Medulla oblongata (gray)
• Pons (gray)
• Cerebellum (light blue)
• Midbrain (mesencephalon, dark blue)
• Interbrain (diencephalon, yellow)
• Forebrain (telencephalon, red)
b Embryo with a GL of 27 mm, near the end of the 2nd month of development (end of the embryonic period). The olfactory bulb is developing from the telencephalon, part of the pituitary anlage (neurohypophysis) from the diencephalon.
c Fetus with a GL of 53 mm, in approximately the 3rd month of development. By this time the telencephalon has begun to overgrow the other brain areas. The insula is still on the surface but will subsequently be covered by the cerebral hemispheres (compare with d).
d Fetus with a crown–rump length (CRL, see p. 4) of 27 cm (270 mm), in approximately the 7th month of development. The brain has begun to develop conspicuous gyri and sulci.
Note: The telencephalon grows over all the other brain structures as development proceeds.

B Brain vesicles and their derivatives
The cranial end of the neural tube expands to form three primary brain vesicles: the forebrain (prosencephalon), the midbrain (mesencephalon), and the hindbrain (rhombencephalon). The forebrain (telencephalon) and interbrain (diencephalon) develop from the prosencephalon. The mesencephalon gives rise to the superior and inferior colliculi and related structures. The rhombencephalon differentiates into the pons, cerebellum, and medulla oblongata. The pons and cerebellum are also known collectively as the metencephalon. Some important structures of the adult brain are listed at far right to illustrate the derivatives of the brain vesicles. They can be traced back in the diagram to their developmental precursors.
C Development of the nervous system: cross section through the neural tube, neural crest, and dorsal ectoderm
During development, the neural groove folds away from the overlying dorsal ectoderm and closes to form the neural tube. Cells migrate from the lateral portions of the neural groove to form the neural crest on each side. The central nervous system (brain and spinal cord) develops from the neural tube, while the peripheral nervous system develops from derivatives of the neural crest (see p. 76).

D Differentiation of the neural tube in the spinal cord region during development
Cross section, cranial view.
a Early neural tube, b intermediate stage, c adult spinal cord.
The neurons that form in the basal plate are efferent (motor) neurons, while those that form in the alar plate are afferent (sensory) neurons.
The area between them—the future thoracic, lumbar, and sacral cord—is another zone that gives rise to preganglionic autonomic neurons. The roof plate and floor plate do not form neurons. A knowledge of how these neuron populations are distributed is helpful in understanding the structure of the hindbrain (rhombencephalon, see E).

E Embryonic migratory movements of neuron populations and their effect on the location of the cranial nerve nuclei
Cross section, cranial view. (Visual aid: If we compare the spinal cord to a book, it would be closed in a and open in b and c.)
a In the initial stage, the motor neurons are ventral (anterior), and the sensory neurons are dorsal (posterior). The arrows indicate the directions of migration.
b In the early embryonic stage, the neurons of the alar plate migrate laterally and ventrally (anteriorly).
c In the adult brain (medulla oblongata and pons, derivatives of the rhombencephalon), we can distinguish four nuclear columns (after His and Herrick) that contain functionally distinct cranial nerve nuclei (from medial to lateral):
1. Somatomotor column (lilac)
2. Visceromotor column (orange and green stripes)
3. Viscerosensory column (light blue)
4. Somatosensory column (dark blue)
8.2 Neural Crest Derivatives and the Development of the Peripheral Nervous System (PNS)

A Development of the neural crest cells
At 3 weeks’ development, the notochord induces surface ectoderm in the medial embryonic disc to thicken and form the neural plate (neuroectoderm). The neural plate differentiates to form the primordia of the nervous system. Neural folds are raised on each side of the neural plate, and a median groove develops between them—the neural groove. This groove subsequently deepens and closes to form the neural tube, which sinks below the ectoderm. Portions of the folds that do not contribute to neural tube formation differentiate to form the neural crest. While neural crest cells in the future head region start to migrate even before the neural tube is closed, crest migration in the trunk is delayed until tube closure. Cells destined to form the neural crest detach from the ectoderm at the fusing margins of the neural tube and undergo an epithelio-mesenchymal transition, diving into underlying mesoderm, where they begin a long and tortuous migration. As the neural tube differentiates into the central nervous system, migrating neural crest cells settle in different locations and develop into sensory and autonomic ganglia, endocrine glands, melanocytes, cartilage, and other structures (see B and C) (after Wolpert).

B Main migratory pathways and derivatives of the neural crest (after Christ and Wachtler)
Neural crest cells originated in different regions have different migration paths and fates. Those from cranial levels contribute to cartilage and bone in the head and neck, and to cranial parasympathetic ganglia (see C). Those from thoracolumbar levels do not become skeletal cells, but generate peripheral neurons, endocrine cells, melanocytes, and Schwann cells. This diagram shows the migration of the neural crest cells in the trunk of the early embryo (4 weeks’ development, see p. 7). They follow three main migratory pathways:
① Dorsolateral pathway (melanoblasts, which differentiate into melanocytes)
② Ventrolateral pathway (ganglioblasts, which differentiate into sensory nerve cells in the dorsal (posterior) root [spinal] ganglia)
③ Ventral pathway (cells differentiate into neurons and associated cells of the paravertebral sympathetic ganglia, into chromaffin cells of the adrenal medulla, and into autonomic plexuses in the gastrointestinal tract)
Thus, the neural crest can develop into a variety of seemingly unrelated nonneuronal cells as well as peripheral ganglion cells. These unusual characteristics of the neural crest—its pluripotential capacity and wide-ranging migration—have consequences when its differentiation or migration is defective. Disruption of neural crest development may deprive organs of their autonomic innervation (Hirschsprung disease). Tumors derived from neural crest cells tend to be highly malignant and difficult to treat. (See D.)
C Neural crest derivatives in the head and neck region
Besides the equivalents of the structures named in B (e.g., melanocytes), other structures in the head and neck region that originate from the cranial neural crest are skeletal and cartilaginous muscles and muscles of facial expression.
a Cranial neural crest derivatives in the adult skeleton: facial bones, hyoid bone, portions of the thyroid cartilage.
b Most of the facial skin is derived from the neural crest.
D Diseases of neural crest derivatives (selected examples)
Neural crest | Disease |
Parasympathetic visceral ganglia | Neuroblastoma (malignant childhood tumor) |
Enteric nervous system | Hirschsprung disease (aganglionic colon) |
Glial cells (Schwann cells, satellite cells) | Neurofibromatosis (Recklinghausen disease) |
Melanocytes | Malignant melanoma, albinism |
Adrenal medulla | Pheochromocytoma (adrenal gland tumor) |
Endocrine cells of the lung and heart | Carcinoids (malignant tumors with endocrine activity) |
Parafollicular cells (C cells) of the thyroid gland | Medullary thyroid carcinoma |

E Development of a peripheral nerve
Afferent (blue) and efferent (red) axons sprout separately from the neuron cell bodies during early development (a). Primary afferent (sensory) neurons develop in the dorsal (posterior) root (spinal) ganglia, and primary motor neurons develop from the basal plate of the spinal cord (b). Interneurons (black), which connect sensory ganglia and motor neurons, develop later.

F Structure of a peripheral nerve
A peripheral nerve consists entirely of axons (also called neurites) and sheath tissue (Schwann cells, fibroblasts, blood vessels). The axons transmit information either from the periphery to the CNS (afferents) or in the opposite direction from the CNS to the periphery (efferents). Axons may be myelinated or unmyelinated. The latter have a much slower conduction velocity and are usually fibers of the autonomic nervous system (see p. 97). Among the investing layers of the nerve, the perineurium ensheaths the nerve fascicles and provides an important tissue barrier (see p. 95).
8.3 Topography and Structure of the Nervous System

A Topography of the nervous system
a Posterior view, b right lateral view.
The central nervous system (CNS), consisting of the brain (encephalon) and spinal cord, is shown in pink. The peripheral nervous system (PNS), consisting of nerves and ganglia, is shown in yellow. The nerves arising from the spinal cord leave their bony canal through the intervertebral foramina and are distributed to their target organs. The spinal nerves are formed in the foramina by the union of their dorsal (posterior) roots and ventral (anterior) roots (see p. 83). The small spinal ganglion in the intervertebral foramen appears as a slight swelling of the dorsal root (visible only in the posterior view; its function is described on p. 83).
In the limbs, the ventral rami of the spinal nerves come together to form plexuses. These plexuses then give rise to the peripheral nerves that supply the limbs.
B Spinal nerves and cranial nerves
Anterior view. Thirty-one pairs of spinal nerves arise from the spinal cord in the peripheral nervous system, compared to 12 pairs of cranial nerves that arise from the brain. The cranial nerve pairs are traditionally designated by roman numerals.
Note: The first two cranial nerves, the olfactory (II) and optic (I) nerves, are not peripheral nerves in the strict sense, but outpouchings from the brain, which means they are pathways of the central nervous system. They are enclosed within the meninges and contain cells that are exclusively found in the central nervous system, oligondendrocytes and microglial cells.

C Terms of location and direction in the CNS
Midsagittal section, right lateral view.
Note two important axes:
① The almost vertical brainstem axis (corresponds approximately to the body axis).
② The horizontal axis through the diencephalon and telencephalon. Keep these reference axes in mind when using directional terms in the CNS.

D Schematic representation of information flow in the nervous system
The information encoded in nerve fibers is transmitted either to the CNS (brain and spinal cord) or from the CNS to the periphery (PNS, including the peripheral parts of the autonomic nervous system, see p. 96). Fibers that carry information to the CNS are called afferent fibers or afferents for short; fibers that carry signals away from the CNS are called efferent fibers or efferents.
8.4 Cells of the Nervous System

A The nerve cell (neuron)
The neuron is the smallest functional unit of the nervous system. Neurons communicate with other nerve cells through synapses. The synapses that end at nerve cells usually do so at dendrites (as seen here). The transmitter substance that is released at the synapses to act on the dendrite membrane may have an excitatory or inhibitory action, meaning that the transmitter either increases or decreases the local action potential at the nerve cell membrane. All of the excitatory and inhibitory potentials of a nerve cell are integrated in the axon hillock. If the excitatory potentials predominate, the stimulus exceeds the excitation threshold of the neuron, causing the axon to fire (transmit an impulse) according to the all-or-nothing rule.

B Electron microscopy of the neuron
Neurons are rich in rough endoplasmic reticulum (protein synthesis, active metabolism). This endoplasmic reticulum (known also as Nissl substance) is easily demonstrated by light microscopy using cationic dyes, which bind to the phosphodiester backbone of the ribosomal RNAs. The distribution pattern of the Nissl substance is used in neuropathology to evaluate the functional integrity of neurons. Neurotubules and neurofilaments are referred to collectively as neurofibrils in light microscopy, as they are too fine to be identified as separate structures under a light microscope. Neurofibrils can be demonstrated in light microscopy by impregnating the nerve tissue with silver salts. This is of interest in neuropathology because the clumping of neurofibrils is an important histologic feature of Alzheimer disease.

C Basic forms of the neuron and its functionally adapted variants
The horizontal line marks the region of the axon hillock, which represents the initial segment of the axon. (The structure of a peripheral nerve, consisting only of axons and sheath tissue, is shown on p. 77.)
a Multipolar neuron (multiple dendrites) with a long axon (= long transmission path). Examples are projection neurons such as α-motoneurons in the spinal cord.
b Multipolar neuron with a short axon (= short transmission path). Examples are interneurons like those in the gray matter of the brain and spinal cord.
c Pyramidal cell: Dendrites are present only at the apex and base of the tridentate cell body, and the axon is long. Examples are efferent neurons of the cerebral motor cortex.
d Purkinje cell: An elaborately branched dendritic tree arises from a circumscribed site on the cell body. The Purkinje cell receives many synaptic contacts from afferents to the cerebellum and is also the efferent cell of the cerebellar cortex.
e Bipolar neuron: The dendrite branches in the periphery. Examples are bipolar cells of the retina.
f Pseudounipolar neuron: The dendrite and axon are not separated by a cell body. An example is the primary afferent (= first sensory) neuron in the spinal ganglion (see p. 91).
D Synaptic patterns in a small group of neurons
Axons can terminate at various sites on the target neuron and form synapses there. The synaptic patterns are described as axodendritic, axosomatic, or axoaxonal. Axodendritic synapses are the most common (see also A).

E Electron microscopy of synapses in the CNS
Synapses are the functional connection between two neurons. They consist of a presynaptic membrane, a synaptic cleft, and a postsynaptic membrane. In a spine synapse (1), the presynaptic knob (bouton) is in contact with a specialized protuberance (spine) of the target neuron. The side-by-side synapse of an axon with the flat surface of a target neuron is called a parallel contact or bouton en passage (2). The vesicles in the presynaptic expansion contain the neurotransmitters that are released into the synaptic cleft by exocytosis when the axon fires. From there the neurotransmitters diffuse to the postsynaptic membrane, where their receptors are located. A variety of drugs and toxins act upon synaptic transmission (antidepressants, muscle relaxants, toxic gases, botulinum toxin).

F Cells of the neuroglia in the CNS
Neuroglial cells surround the neurons, providing them with structural and functional support (see G). Various staining methods are available in light microscopy for selectively demonstrating different portions of the neuroglial cells:
a Cell nuclei demonstrated with a basic stain.
b Cell body demonstrated by silver impregnation.
G Summary: cells of the CNS and PNS and their functional importance
Type of cell | Function |
Neurons (CNS and PNS) | 1. Impulse formation 2. Impulse conduction 3. Information processing |
Glial cells |
|
Astrocytes (CNS only) | 1. Maintain a constant internal milieu in the CNS 2. Contribute to the structure of the blood–brain barrier (see p. 95) 3. Phagocytize dead synapses 4. Form scar tissue in the CNS (e.g., in multiple sclerosis or following a stroke) |
Microglial cells (CNS only) | Phagocytosis (“macrophages of the brain”) |
Oligodendrocytes (CNS only) | Myelin sheath formation in the CNS |
Schwann cells (PNS only) | Myelin sheath formation in the PNS |
Satellite cells (PNS only) | Modified Schwann cells; surround the cell body of neurons in PNS ganglia |
8.5 Structure of a Spinal Cord Segment

A Structure of a spinal cord segment with its spinal nerve
Superior view. The spinal cord is made up of 31 consecutive segments arranged one above the other (see B). An anterior root and a posterior root emerge from the sides of each segment. The anterior root consists of efferent (motor) fibers, while the posterior root consists of afferent (sensory) fibers. Both roots from one segment unite in the intervertebral foramen to form the spinal nerve. The afferent (sensory) fibers and efferent (motor) fibers intermingle at this junction, so that the branches into which the spinal nerve divides (see below) contain motor and sensory elements (except for the meningeal branch, which is purely sensory). This division into branches (rami) occurs shortly after the anterior and posterior roots unite to form the spinal nerve. Consequently, the spinal nerve itself is only about 1 cm long.
The principal branches of the spinal nerve have the following functions:
• The anterior ramus innervates the anterior and lateral body wall and the limbs.
• The posterior ramus innervates the skin of the back and the intrinsic back muscles.
• The meningeal branch reenters the spinal canal, providing sensory innervation to the spinal membranes and other structures.
• The white ramus communicans carries white (= myelinated) fibers to the ganglion of the sympathetic trunk.
• The gray ramus communicans carries gray (= unmyelinated) fibers from the sympathetic ganglion back to the spinal nerve (the functional significance of this is described on p. 97). The anterior and posterior rami of the spinal nerves subdivide into further branches.
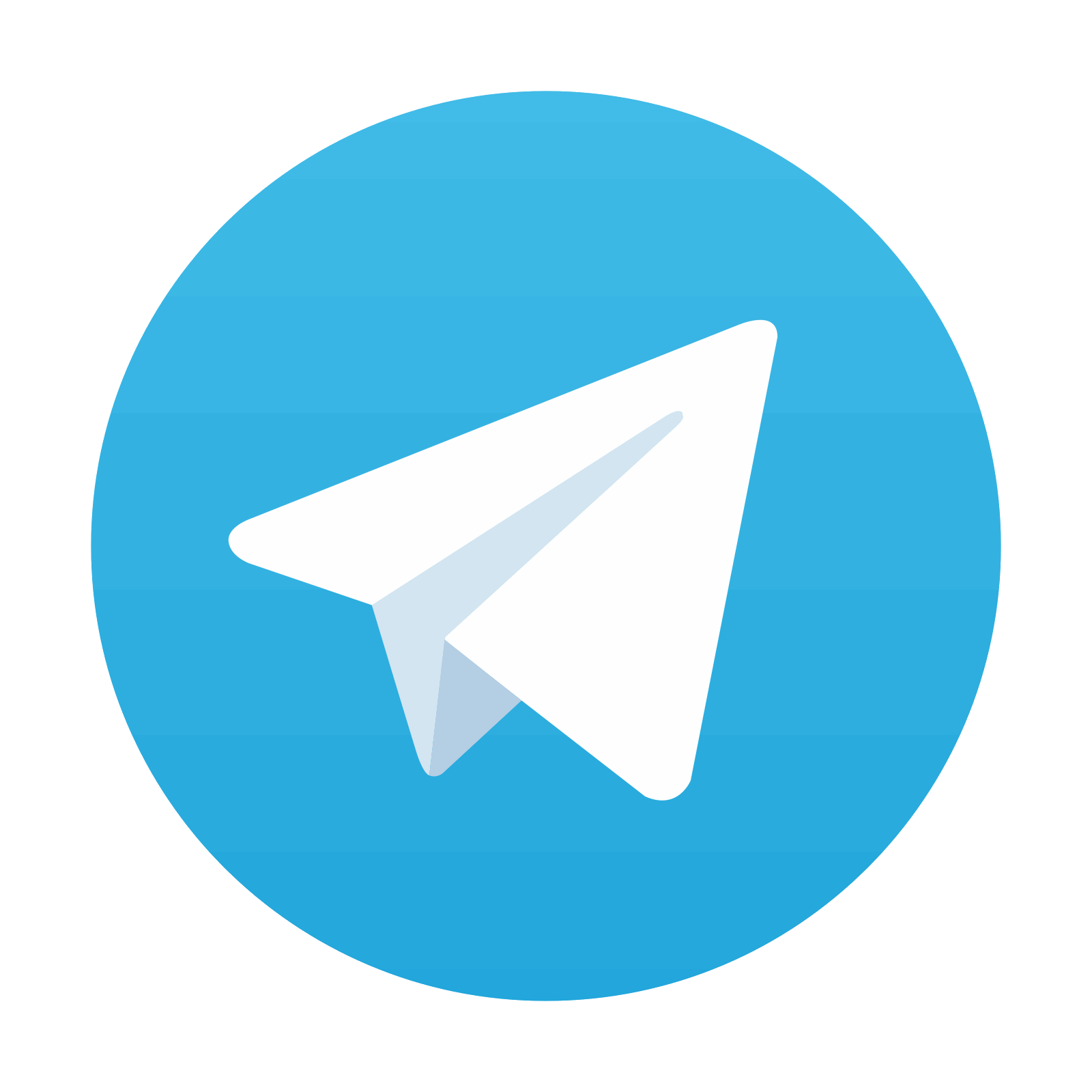
Stay updated, free articles. Join our Telegram channel

Full access? Get Clinical Tree
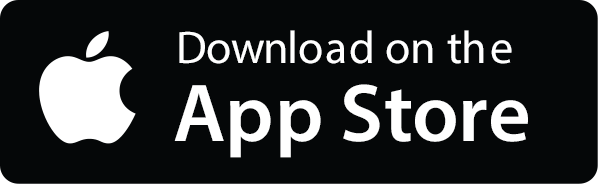
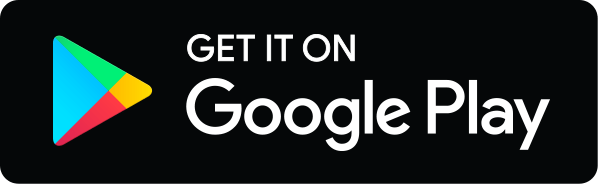