Fig. 16.1
A wound response signature of genes differentially expressed between tissue from reduction mammoplasty and histologically normal tissue of cancer patients. The dendrogram in cancer-adjacent normal samples (red) and reduction mammoplasty samples (green) in (a). The clusters show that various biological processes involved in wound repair are differentially expressed between the two groups, including ECM alterations (b, c), immediate early (IE) genes involved in angiogenesis (d), reepithelialization and cellular adhesion (e), and cellular adhesion and chemotaxis (f). (Adapted from Clinical Cancer Research, 2009, 15/22, 7020–8, Troester et al., “Activation of Wound Host Responses in Breast Cancer Microenvironment,” with permission)
Finak and colleagues applied tissue laser-capture microdissection (Fig. 16.2a) to assess the gene expression pattern of tumor stroma in primary breast cancer [43]. Several gene expression signatures identified in this series associated with disease course. The 26-gene signature denoted “stroma-derived prognostic predictor” pointed to contrasting immune responses and angiogenic and hypoxic responses in different tumors. This signature also predicted prognosis, as validated in multiple breast cancer data sets (Fig. 16.2b, c). Based on clustering of the 26-gene signature, the authors suggested stroma-dependent breast cancer subtypes. The stroma signature of Finak predicted clinical outcome independent of other signatures, which were also associated with prognosis, indicating that the “stroma-derived prognostic predictor” mirrors specific biological processes taking part in directing the clinical disease course. In this study, Finak and colleagues demonstrated an independent stromal impact within the tumor, showing that genes of their “stroma-derived prognostic predictor” did not predict prognosis when assessed in the epithelial component.


Fig. 16.2
Laser-capture microdissection of breast carcinomas to assess gene expression pattern of tumor stroma (a). The “stroma-derived prognostic predictor” predicted relapse and metastatic disease and pointed to contrasting immune responses and angiogenic and hypoxic responses in different tumors (b). The signature also predicted prognosis, as validated in multiple breast cancer data sets (c). (Figure 16.2a is reprinted from Breast Cancer Research, copyright 2006, 8:R58, Finak G. et al., with permission from BioMed Central Ltd. Figure 16.2b, c are reprinted by permission from Macmillan Publishers Ltd.: Nature Medicine 14(5):518–27, copyright 2008)
When combining the stroma signatures identified by Finak with other signature scores of prognostic value, the prediction of metastatic disease improved, suggesting an even better reflection of the stroma-related processes when merging signatures developed in different analytical approaches.
How do the stromal and epithelial cells communicate? Are we able to reflect the interplay between these two compartments by the use of gene expression data? To address these questions, Casey and colleagues examined the transcriptomic expression pattern of epithelial and stromal cells, both in normal breast tissue and in invasive breast cancer [44]. Cell type-specific interactions were also assessed. A “motile phenotype ” was identified in the epithelial compartment and a “reactive phenotype” in the stromal compartment, with genes reflecting remodeling of the extracellular matrix in a proteolytic manner in the invasive cancer. Also, genes promoting epithelial-mesenchymal interaction, such as fibroblast activation protein alpha (FAP), were identified. This study interestingly supports a molecular crosstalk between the epithelial and stromal cell compartments and suggests that alterations facilitating invasion are one of the features of cancer-associated stroma.
By examining global gene expression alterations relating to specific tumor microenvironment elements that are microscopically assessable, it might be possible to identify underlying alterations of the histopathologic phenotype. Van den Eynden examined fibrotic tumor foci and associated gene expression patterns [45] and demonstrated Ras signaling and HIF1α-pathway activation along with other hypoxia- and angiogenesis-related genes in the large fibrotic foci. Also, fibrotic foci correlated with an activated wound healing signature [46] and with earlier development of distant metastases.
What would be the model system best fit to capture ongoing microenvironmental processes promoting tumor progression? Marchini and colleagues examined the transcriptomic alterations in A17 mouse mammary carcinoma cells [47]. Three gene expression signatures reflecting stroma-related features and processes were identified: One “stemness signature,” one “angiogenesis signature,” and one “signal transduction signature.” These signatures are associated with mesenchymal stem cell signatures, ER-negative breast cancer, a basal-like phenotype, and breast cancer bone metastases. In post treatment assessment of breast cancer xenograft models, the A17 angiogenesis and signal transduction signatures were more highly expressed after hormonal therapy. This study indicates a linkage between mesenchymal features, tumor progression, and therapy resistance, directing an interpretation of these findings toward epithelial-mesenchymal transition (EMT) . EMT is regarded as having critical importance in tumor progression [48], and recent studies indicate that epithelial-mesenchymal plasticity contributes to stemlike tumor features and generates cancer stem cells [49–51].
Are the tumor microenvironmental changes in cancer progression common or specific across tumor types? Planche and colleagues examined this question by laser microdissecting stromal cells of invasive breast and prostate carcinoma. These two tumor types displayed distinctly different stromal gene expression patterns [52]. The expression alterations of the cancer type-specific stromal genes clustered both breast and prostate cancer samples into groups with different disease courses. Of note, genes of extracellular matrix constituents and proteolytic enzymes were upregulated in the invasive breast cancer stroma, in line with the observations done on the tumor histology sections.
Most mRNA expression studies extract RNA from tissue that is snap frozen in the surgical theater, as the RNA is best preserved for quantitative analyses in this manner, as compared to that extracted from formalin-fixed paraffin-embedded (FFPE) tissue. However, as of the writing of this chapter, FFPE patient-derived tissue is generally more widely available, as it is stored in pathology labs worldwide. Winslow and colleagues made a critical step forward in this field when they succeeded in studying gene expression alterations from laser-dissected tumor epithelial and stromal compartments from FFPE-invasive breast cancer samples. This study found that stroma-specific gene expression signatures segregated into three major thematic groups: (1) extracellular matrix- and fibroblast-related genes, (2) vascular-related genes, and (3) immune cell-related genes. Strikingly, the immune-related signature is associated with basal-like breast cancer subtype [53]. As the results from this study were in line with other similarly designed studies on fresh frozen tissue, the study gave new hope for RNA studies on FFPE tissue.
A few studies have related global gene expression data to specific molecular microenvironmental alterations. Specifically, a relationship between CD10+ stromal cell expression and breast cancer progression was previously reported [39], and Desmedt and colleagues followed up on this by exploring gene expression alterations related to CD10+ stromal cells [54]. A “CD10+ stroma signature ” of 12 genes was generated by comparing the gene expression patterns of CD10+ cells isolated from breast carcinomas and normal breast tissue. In co-culture experiments, the CD10+ cells were characterized as specific cell populations: fibroblasts, myoepithelial cells, and mesenchymal stem cells. As seen in many of the stroma- and CAF-derived signatures, the CD10+ signature was composed of genes related to matrix remodeling. Interestingly, genes related to osteoblast differentiation (e.g., osteopontin) were also upregulated in the CD10+ signature. All the different CD10+ cell types contributed to this stroma-related signature; however, the highest CD10+ stroma signature score was found in mesenchymal stem cells. Of clinical value, the signature was able to differentiate in situ and invasive breast cancer lesions. Also, the CD10+ signature demonstrated a potential to predict response to chemotherapy, and high CD10+ stroma score associated with reduced survival in HER2-positive breast cancer cases. This study is a good example of how to combine in vivo and in vitro studies, specifically with respect to validating the functionality of a gene expression signature.
In another study describing gene expression alterations reflecting specific molecular alterations, Rajski and colleagues identified a signature associated with IGF-I-stimulated stromal cells [55]. Among the IGF-I signature genes, there was enrichment of proliferation-associated genes. This signature clustered the cancer samples in two major groups: those with upregulated IGF-I and those without. Cases in the cluster with genes upregulated by IGF-I experienced shorter survival.
An example of a signature related to specific histopathologic tumor features is one necrosis-related signature derived from gene expression alterations between endometrial carcinomas with and without tumor necrosis [56]. In this case, tumor necrosis was found to be associated with gene expression programs of hypoxia, angiogenesis, and inflammatory responses.
The cancer biology underlying phenotypic features of various cancer types may be cancer specific but also share commonalities with other diseases and noncancerous conditions. West and colleagues exploited the potential of approaching the research question from a different angle, when postulating that fibroblasts present with different activation states. Their approach to this question was to distinguish fibroblast populations in noncancerous samples [57]. They found that solitary fibrous tumors and desmoid-type fibromatosis exhibited different expression patterns. In particular, the expression of growth factors and extracellular matrix genes were differentially expressed. When assessing the gene signature separating solitary fibrous tumor from desmoid-type fibromatosis in a series of invasive breast cancer, two groups of breast carcinomas were identified, and patients in these two groups presented with different survival. The cases with an expression pattern similar to the desmoid-type fibromatosis showed more favorable outcome, while the other group showed variable expression of genes enriched in solitary fibrous tumors and was observed with poorer prognosis. These findings supported the hypothesis that tumor stromal response varies among carcinomas of different aggressiveness.
Gene Expression Signatures Reflecting Cancer-Associated Fibroblasts
Most of the studies mentioned above have investigated tissue stroma and thereby potentially reflect expression contribution from the combination of different stromal cell types. Many of the stromal signatures correlate with clinicopathologic features and disease course, and seem to reflect underlying stroma biology. Still, it is tempting to ask: What is the contribution to the signatures from each of the specific stromal cell types?
Chang and colleagues were among the first to generate a pure fibroblast signature, where the expression alterations were generated by exposure of fibroblasts to serum [46]. The signature was denoted a “core serum response.” Functional analyses revealed involvement of the signature genes in myofibroblast activation, matrix remodeling, and cell motility. All these processes contribute to wound healing. Based on the expression of the “wound signature,” breast cancer samples segregated into two groups. The group with activated signature pattern was associated with increased risk of metastatic disease and death from breast cancer. Further, the signature pattern was consistent in paired samples of locally advanced breast carcinomas, biopsied before and after chemotherapy, indicating stability of the biological program reflected in this signature [46]. Interestingly, the basal-like molecular breast cancer subtype significantly associated with the expression pattern of the “wound healing signature,” suggesting that the signature points to intrinsic properties of the basal-like phenotype. The signature was also examined in gene expression data sets of various tumor types, and the findings were striking: The expression pattern of this signature separated the cases in two groups, with significantly increased risk of metastatic disease in the group with the activated signature pattern. Harold F. Dvorak suggested in a review in 1986 the wound as an analog to the stromal processes observed in tumors [58]. The gene expression signature by Chang might have captured some of the alterations observed by Dvorak.
Also, Tchou and colleagues demonstrated subtype-specific stromal gene expression patterns in breast cancer [59]. In this analysis, the expression profiles of CAFs from breast cancer samples of the HER2-positive subtype, triple-negative cases, and ER-positive cases were distinctly different. In particular, pathways linked to the cytoskeleton and integrin signaling were differentially enriched in the different CAF groups. The results from this study add to the arguments of specific “stroma subtypes” in breast cancer and support the hypothesis that fibroblasts participate to the disease biology underlying clinically relevant breast cancer subtypes.
Two projects, exploring transcriptional alterations in tumor-associated fibroblasts compared to normal mammary fibroblasts, demonstrated an increased expression of genes involved in tumor progression in the CAFs. Cytokines, genes related to remodeling of the extracellular matrix, and genes reflecting paracrine or intracellular signaling as well as cell-matrix interactions were upregulated in the tumor-associated fibroblasts [60, 61]. In the study by Singer, it was noted that these gene expression alterations take place also in the isolated cell culture state, in the absence of adjacent malignant epithelium [61]. In the study by Bauer, the CAF-associated genes were incorporated into a 31-gene signature that was validated by qPCR. Some of the genes upregulated in CAFs were validated by immunohistochemistry, with respect to location and quantitation [60]. Taken together, the findings from these two studies are supportive with respect to indicating a fibroblastic subpopulation of the tumor stroma that facilitates tumor progression.
By comparing global gene expression patterns of platelet-derived growth factor (PDGF)- stimulated human fibroblasts and resting fibroblasts, Frings and colleagues identified a 113-gene expression signature reflecting PDGF-activated fibroblasts [62]. This signature had the potential to identify breast cancers with a stroma of PDGF-stimulated fibroblasts. The signature correlated with high expression of the PDGF receptor β (PDGFRβ) and its ligands, and was enriched for genes related to angiogenesis and regulation of the extracellular matrix. Signature analyses in several breast cancer data sets demonstrated associations between the PDGF signature score and clinicopathologic features reflecting aggressive tumors, such as large tumor size, high histologic grade, and HER2-positive and ER-negative tumors. Moreover, signature activation correlated with the HER2-positive, basal-like, and luminal B subtypes of breast cancer. In line with these observations, the signature demonstrated a robust association with survival. High signature score correlated with reduced survival, specifically in multivariate analyses when adjusted for other stroma signatures and a proliferation signature.
Siletz and colleagues assessed transcription factor signatures and activity (by array method) specific for mammary CAFs versus normal mammary fibroblasts [63]. A transcription factor activity signature included activation of reporters for ELK1, GATA1, retinoic acid receptor, serum response factor, and vitamin D receptor (VDR). An increased activation of reporters for HIF1, and several STAT and proliferation-related transcription factors, was seen after induction of fibroblasts by conditioned medium from breast cancer cell lines. These transcription factor activity profiles indicate CAF subtype-specific signaling that promotes tumor progression through a pro-invasive stroma.
Validating the potential functionality of gene expression signatures is a challenging task. A study by Navab and colleagues analyzed how the gene expression patterns in matched CAFs and normal fibroblasts promote tumor progression in lung cancer cell lines [64]. A 46-gene signature was enriched for genes encoding proteins regulated in particular by the TGF-β signaling pathway. The involvement of TGF-β was confirmed by additional network analyses. Additionally, a subset of the signature genes was induced by TGF-β. Eleven of the signature genes predicted prognosis in several non-small cell lung cancer data sets. This study demonstrates one example of functional validation with good study design.
Woelfle and colleagues derived a signature of 86 genes differentially expressed between primary tumors with and without bone marrow metastases [65]. Although the tumor microenvironment was not the focus of this study when deriving this signature, the majority of the signature genes were related to extracellular matrix remodeling, cytoskeleton plasticity, and cell adhesion. Also, RAS- and HIF1α signaling were enriched in tumors with bone marrow metastases. The many similarities between this signature and the stroma- and CAF-related signatures described above lead to an intriguing perspective on this signature. In addition to facilitate invasive growth and tumor progression, perhaps the tumor stroma is heavily involved in directing tumor metastases to different locations? Another interesting perspective of this signature was that 77 of the 86 signature genes were downregulated in primary tumors with bone marrow metastases, indicating transcriptional repression as part of the picture in tumor progressive processes.
A few studies have examined transcriptional alterations related specifically to the extracellular matrix in breast cancer. Bergamaschi and colleagues set out to classify breast carcinomas based on constituents of the extracellular matrix (ECM) , selecting 278 ECM-related genes from the literature [66]. These ECM-related genes segregated the breast cancer samples into four ECM classes with different clinical courses. The ECM group that associated with best survival showed upregulation of protease inhibitors of the serpin family. The ECM group associated with poorest survival presented with overexpression of integrins and metallopeptidases and low expression of laminin chains. In a follow-up study, Triulzi and colleagues demonstrated that one of the ECM groups consistently predicted one cluster in several independent breast cancer data sets [67]. The 58-gene signature of this ECM-subset contained 43 genes encoding structural ECM proteins. Investigation of gene expression data sets on separate cancer epithelial and stromal cells demonstrated that genes of this ECM signature were expressed both by the epithelial and stromal compartments. In vitro experiments showed induction of signature genes, in particular in fibroblasts and in ER-negative breast cancer cells. Single genes and gene sets reflecting EMT were significantly associated with this ECM signature.
Gene Expression Signatures Reflecting Vascular Biology
Various measures of histologically verified tumor vasculature (e.g., mean vessel density, vascular proliferation) are related to tumor progress and metastatic disease in solid cancer types. The vasculature is viewed as a target for therapy, as exploited in therapeutic programs in several tumors. Studies on genomic programs measuring the transcriptional alterations have a strong potential to reveal novel aspects of vascular biology in malignant tumors.
With this in mind, Wallgard and colleagues sought to elucidate the transcriptome and molecular processes specific to endothelial cells [68]. Fifty-eight genes specifically linked to microvascular expression were identified, many of them not previously described in relation to functions of endothelial cells. Wallgard suggested several genes, in particular those expressed on the cell surfaces, to be further explored in relation to drugs targeting the microvasculature.
The vasculature is regarded as the main route for breast cancer metastases, and comparing the global transcription pattern of primary tumors and distant metastases might point to vascular-related biology. Hu and colleagues applied this approach and identified an in vivo hypoxia signature reflecting VEGF activation and also predicting poor clinical outcome in breast cancer and other tumor types [69]. This 13-gene signature was composed of several angiogenesis-related genes, and 8 of the 13 genes contained binding sites for the hypoxia-related transcription factor HIF1α and had been demonstrated to be regulated by HIF1α.
Pepin and colleagues identified two tumor vasculature types by analyzing global transcription patterns across laser-capture microdissected tumor-associated and matched normal vasculature [70]. The two tumor vasculature types demonstrated specific gene expression signatures. One of these was related to anti-angiogenic signaling. Samples enriched for this signature demonstrated lower mean vessel density as compared to the group enriched for the gene signature associated with active vascular remodeling and reduced vascular shear stress. Reduced vascular shear stress is suggested to reflect reduced vessel flow rate and may reflect an inappropriate tumor perfusion. Significantly, several therapeutic targets with potential relevance in anti-angiogenic treatment (e.g., MET, PDGFRβ, ITGAV) were differentially expressed between the vasculature subtypes.
When studying alterations in vascular gene expression, different study design may reveal different layers of the full picture. In a supervised manner, in an analysis of genes known to be angiogenesis related, Bender and colleagues demonstrated that the gene expression of the VEGF and semaphorin families was altered in pro-angiogenic manners [71]. A signature of these genes was associated with triple-negative breast cancer and also with reduced survival in this tumor subtype.
Wallace and colleagues approached angiogenesis-related biology in a more indirect manner. In an analysis of genes and pathways mediating fibroblast contribution in cancer progression [72], the authors studied how Ets2 function varied between mammary stromal fibroblasts and epithelial cells. In HER2-positive breast cancer mouse models, Ets2 inactivation in fibroblasts reduced tumor growth. The same effects were not seen when inhibiting Ets2 in epithelial cells. An Ets2-dependent gene signature was derived, enriched in genes related to remodeling of the extracellular matrix, cell migration, and angiogenesis. Supportive to these functional interpretations, Wallace found fewer functional blood vessels in tumors lacking Ets2 in the fibroblasts. The Ets2-dependent gene expression signature was able to segregate human breast cancer stroma and normal stroma. This study indicated a link between Ets2 and the fibroblast-endothelial cross talk and points to a contribution of Ets2 in the angiogenic process.
Xiao and colleagues [73] have developed in vitro models studying breast cancer-specific endothelial cells and have identified multiple subpopulations of tumor-associated endothelial cells, each population with distinct gene expression patterns. For several of the genes, a relationship with tumor-associated endothelial cells had not previously been established. For example, the genes Irx2 and Zfp503 were highly upregulated in tumor endothelial cells but had no previous known relevance to vascular biology. These genes are known to regulate neuronal patterning and developmental differentiation [74, 75] and may point to new information on vascular-related mechanisms and co-regulatory circuits in vascular biology.
Mannelqvist and colleagues published an 18-gene expression signature related to vascular invasion in endometrial carcinomas, also relating to features of aggressive disease and disease outcome [76]. In a follow-up study on multiple breast cancer gene expression data sets, the vascular invasion signature was associated with tumor progression and clinical course in breast cancer [77]. Also, a high signature score associated with the basal-like phenotype and response to neoadjuvant chemotherapy. The signature was composed of genes related to angiogenesis, immune response, and extracellular matrix biology. Further, the vascular invasion signature identified by Mannelqvist correlated with other gene expression profiles of vascular biology, hypoxia, EMT, immune response, and tumor progression.
The same research group later published a 32-gene signature reflecting tissue-based vascular proliferation. Microvessel proliferation was assessed by dual endothelial immunostaining of factor VIII/Ki67, and global gene expression data as well as copy number information were explored in supervised manners [78]. Several genes in the signature had previously been linked to processes such as neovascularization, endothelial cell migration, and adhesion, supporting this signature as relevant for tumor angiogenesis. Also, amplification of the region 6p21, potentially harboring VEGF, associated with high microvessel proliferation.
Harrell and colleagues sought to determine whether tumor-associated vascular properties could identify mechanisms contributing to the different risks of metastatic disease across the intrinsic subtypes of breast cancer [79]. They found that claudin-low and basal-like tumors were enriched for transcriptional programs reflecting vascular quantity, vascular proliferation, and a VEGF/hypoxia signature. Incorporating several of the vascular gene signatures described above added information about risk of metastatic disease. Furthermore, experimental studies demonstrated that claudin-low cells exhibited endothelial-like morphology, and claudin-low xenograft tumors were highly perfused through intercellular spaces and nonvascular tumor cell-lined channels. This study combines the transcriptional studies with experimental validation in an interesting manner and demonstrates both endothelial-like characteristics of cancer cells and how the vasculature in conceptually new manners may contribute to breast cancer progression. Also, the gene expression signatures were suggested as predictive markers to anti-angiogenic therapy.
Pitroda and colleagues explored how vascular inflammation influences cancer prognosis [80]. A gene expression signature reflecting inflammation in tumor-associated endothelial cells was developed. The endothelial-derived 6-gene inflammatory signature predicted reduced overall survival in breast cancer and other tumor types. Also, inflammatory pathways activated in endothelial cells linked to tumor progression in mice, supporting a vasculo-immunogenic link contributing to tumor progression in breast cancer.
Gene Expression Signatures Reflecting Immune-Related Alterations
The role of the immune system is one of the emerging areas in cancer research and has become important in many cancer-related discussions: its role in cancer prevention, as part of therapeutic strategies, as a prognosticator and predictive marker, and not least its role in tumor progression. The last part is not yet well understood. It is probably more realistic to state that per today (2016) we have likely reached the beginning of this field, seeing the picture as T.S. Eliot reflected: “What we call the beginning is often the end. And to make an end is to make a beginning. The end is where we start from.”
Perou and colleagues had in their early breast cancer classification study touched upon the transcriptional heterogeneity of ER-negative breast cancer [17]. Teschendorff and colleagues followed up on this and demonstrated transcriptional alterations associated with the clinical course of ER-negative breast cancer [81]. Distinct subclasses among ER-negative tumors were shown based on transcriptional patterns. One of the classes consisted of basal-like tumors with upregulation of genes related to immune response and complement activation. This subset of ER-negative samples demonstrated better survival pattern as compared to the rest of ER-negative tumors. Based on this study, a seven-gene immune response signature was derived. Downregulation of this module associated with increased risk of advanced disease.
Rody and colleagues followed up on this study and focused on the clinically and prognostically heterogeneous triple-negative breast cancer subtype [82]. The basal-like and claudin-low subtypes were described by metagenes reflecting angiogenesis, inflammation, and nonneoplastic cell types like immune cells, adipocytes, and fibroblasts. High immune cell score associated with improved survival, and high inflammation and angiogenesis scores correlated with reduced survival. Rody defined a ratio of the B-cell and IL-8 metagenes, and a subgroup (32%) of triple-negative cases with high B-cell and low IL-8 scores experienced improved outcome.
Further, two other breast cancer studies have underpinned the association between an immune response and tumor subsets with milder disease courses [83, 84]. In the study by Alexe and colleagues, a HER2-positive subtype with low recurrence rate associated with high expression of lymphocyte-associated genes [83]. Also, a prominent lymphocytic infiltration was seen by histologic examination of these tumor cases. In the study by Schmidt and colleagues, high B-cell metagene score associated with metastasis-free survival in node-negative cases with high proliferation, as validated both in high-grade cases and in young breast cancer patients [84].
Schmidt and colleagues [85] followed up on this study and aimed to identify one single immune system marker for cancer progression. Immunoglobulin κC (IGKC) demonstrated similarly predictive and prognostic value as the entire B-cell metagene [84], (Fig. 16.3). IGKC gene expression associated with improved survival across different molecular subtypes in node-negative breast cancer. Also, levels of IGKC measured by immunostaining in a series of FFPE breast cancer tissues correlated with clinical outcome. Tumor-infiltrating plasma cells were identified as the source of the protein. The findings suggest further exploration of the humoral immune response and its relevance in the therapeutic setting.


Fig. 16.3
IGKC immunostaining intensities in ductal breast carcinomas; note the expression within the desmoplastic stroma in-between tumor cell nests (a). Low IGKC immunostaining intensity associates with shorter metastasis-free survival (b). In (c), coexpression by immunofluorescence of IGKC with a marker for plasmablasts and plasma cells [MUM1/IRF4]; IgG coexpression illustrates the isotype switch; B-cell marker CD20; epithelial cell marker cytokeratin shows tumor cells. IGKC is visualized by green fluorescence, whereas MUM1/IRF4, IgG, CD20, and cytokeratin emit a red signal. (Reprinted from Clinical Cancer Research, 2012, 18/9, 2695–703, Schmidt et al., “A Comprehensive Analysis of Human Gene Expression Profiles Identifies Stromal Immunoglobulin kappa C as a Compatible Prognostic Marker in Human Solid Tumors,” with permission)
One such study specifically examined genes related to TH1-mediated adaptive immunity in breast cancer [86] and demonstrated that inflammation and immune suppression predicted tumor subsets with different clinical outcomes. Data sets on various tumor types were analyzed, and Hsu showed that upregulation of the TH1-mediated adaptive immunity genes correlated with good prognosis in young breast cancer patients (<45 years).
Two other studies demonstrated better survival in cases of high immune signature score in breast cancer [87, 88]. In the study by Bianchini, high expression of a B-cell/plasma cell signature associated with improved survival in ER-positive cases with high proliferation, specifically when adjusting for standard prognostic variables and other transcriptional scores [87]. In the study by Nagalla, a cluster of cases without distant metastases associated with genes related to immunological functions. These genes could be clustered into three major “immune metagenes,” one cluster reflecting B-cells and/or plasma cells, another cluster reflecting T-cells and natural killer cells, and a third cluster reflecting monocytes and/or dendritic cells [88]. In tumors of high proliferation, high immune metagene score associated with reduced risk of metastasis. Cases with low immune metagene scores associated with poorer outcome.
A few studies of immune-related signatures have suggested therapy strategies based on the findings. Ascierto and colleagues [89] elucidated how immune function networks related to tumor-infiltrating immune cells were more highly expressed in cases without recurrent disease. These network genes were related to B-cell development, interferon signaling, and autoimmune reactions as well as antigen presentation pathways. The results indicated cross talk between the adaptive and innate immune systems. Five B-cell response genes predicted relapse-free survival (>85% accuracy), also validated by qPCR. The authors thus suggested immunotherapy, in the neoadjuvant setting, to patients with high risk of recurrent disease, potentially by inducing genes of immune function.
Iglesia and colleagues aimed to elucidate transcriptional alterations related to the cancer immune response of breast and ovarian cancers with high lymphocyte infiltration and improved survival [90]. RNAseq data and a microarray data set were applied to identify signatures reflecting the adaptive immune response. The B-cell signatures predicted improved survival in the basal-like and HER2 subtypes. Further, analyses of B-cell receptor sequences were assessed through RNAseq data. It was previously shown that a clonal expansion of the B-cells and somatic hypermutations in B-cell tumor-infiltrating lymphocytes in breast tissue represent an antigen-directed response [91–93], and the response of antigen-specific B-cell populations actively demonstrate features of clonal expansion. A part of the basal-like and HER2-enriched cases with shorter survival showed upregulation of BCR gene segments with low diversity, indicating lack of B-cell clonal expansion, and was also indicative of an ineffective antigen-directed response in these cases, potentially contributing to their poorer prognosis. More and varied BCR segments with increased expression associated with improved prognosis. The results indicate a limited B-cell antitumor response in a subset of basal-like breast cancer. Also, immunomodulatory therapies were suggested, and supporting B-cell responses may be one relevant approach in B-cell-infiltrated carcinomas.
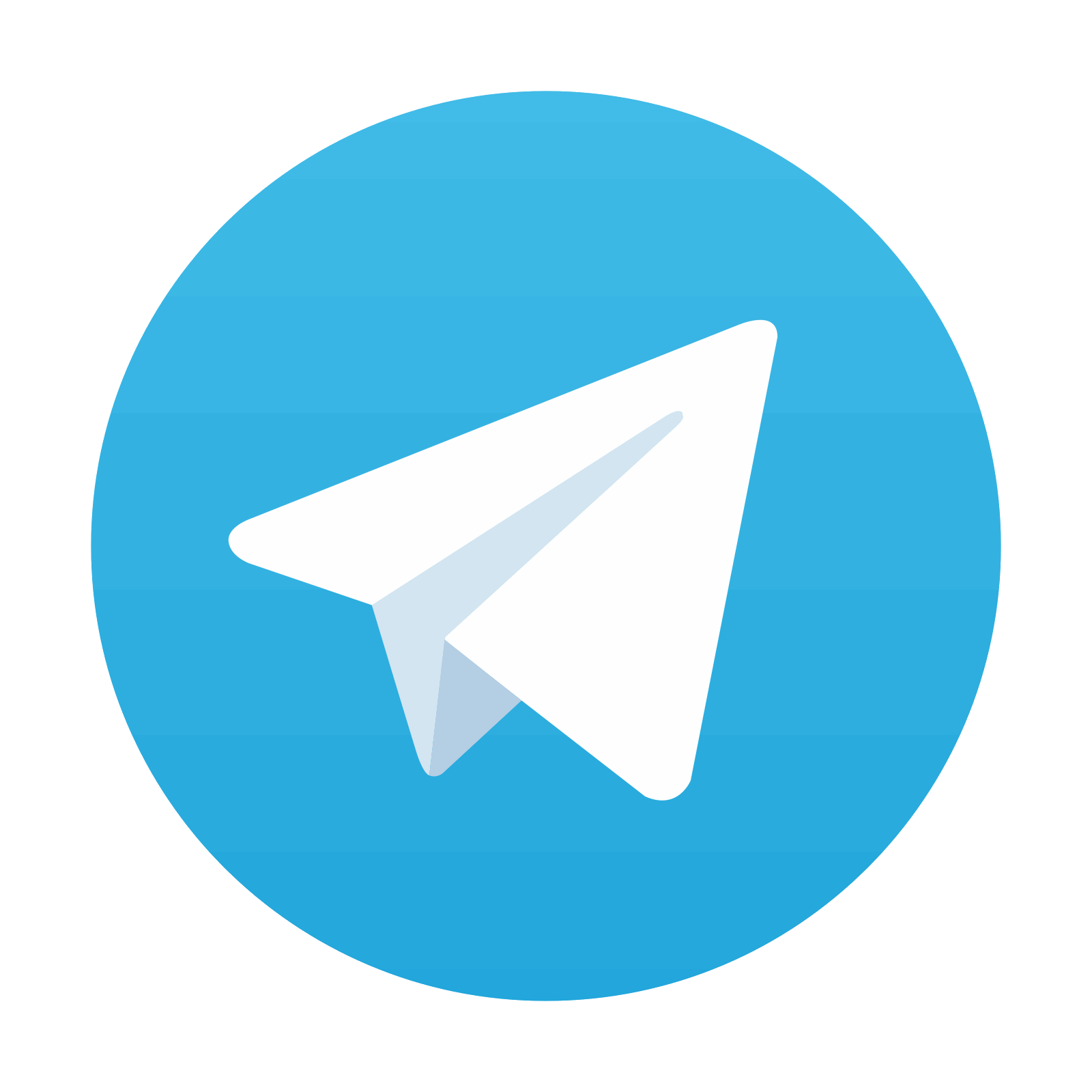
Stay updated, free articles. Join our Telegram channel

Full access? Get Clinical Tree
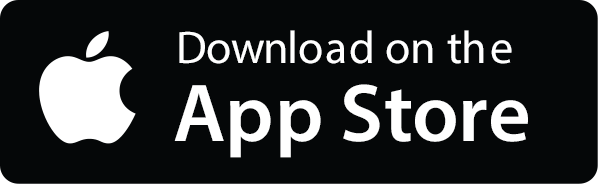
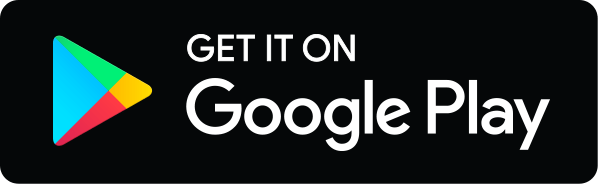