Chapter 6 Gastrointestinal and digestive physiology
6.1 Nutrition and appetite
Nutritional requirements
Vitamin and mineral requirements may also be specified but with even less certainty than energy needs. The dietary requirements of the vitamins folate and B12 and of the mineral iron are of particular clinical interest since deficiencies of these are relatively common and can lead to haemoglobin deficiency, i.e., anaemia (Section 2.3). The recommended daily requirements of folate (200 μg) and B12 (2 μg) are similar in adult men and women. Women have a higher iron requirement, at 15 mg day−1 compared with 10 mg for men. This reflects increased iron loss resulting from menstruation each month.
It may be that other aspects of the diet, e.g., fibre and lipid content, are also important for health. Studies to test such ideas unambiguously are, however, extremely difficult to design and carry out.
Control of eating
Following sustained growth during childhood and adolescence, body weight remains remarkably stable over many years of adulthood. This stability can only be achieved by matching energy intake to energy use. If the food we eat contains more energy than we need then the excess has to be stored, mainly in the form of fat, and weight increases. To maintain a stable weight, therefore, food intake has to be controlled. This is coordinated by the hunger centre and the satiety centre in the hypothalamus (Fig. 89). Following a meal, distension of the stomach and increased levels of circulating nutrients stimulate the satiety centre which inhibits feeding, while hunger develops as these inputs wane. Fat stores also release a protein hormone called leptin which acts on the hypothalamus to inhibit eating. This provides a feedback system tending to regulate the total lipid content (and thus the weight) of the body. Emotional factors also affect the hypothalamic centres, e.g., anxiety can increase or decrease appetite, and eating disorders, such as anorexia nervosa or bulimia, may reflect abnormalities in central nervous control of their activity.
6.2 Gastrointestinal motility
General features of gut motility
The propulsion of gastrointestinal contents depends on the contractile activity of the gastrointestinal smooth muscle, which is generally organized as an inner circular and an outer longitudinal layer in the intestinal wall (Fig. 90). The electrical activity in this muscle consists of spontaneous waves of depolarization lasting several seconds, which are known as slow waves (Fig. 91). These may have action potential spikes superimposed on their peaks. The electrical activity in smooth muscle is dependent on Ca2+, rather than Na+ as is the case in nerves and striated muscle. The frequency of the slow waves varies in different parts of the gastrointestinal tract, with average values of about 3 min−1 in the stomach, 12 min−1 in the upper small intestine, falling to 9 min−1 in the terminal ileum. Slow waves excite spontaneous contractility, which is modified by the action of nerves, hormones and local factors such as chemical stimulation and mechanical stretch. Nervous control comes both from extrinsic autonomic nerves and intrinsic nerves, with the latter forming the myenteric and submucosal nerve plexuses within the wall of the gut itself.
Mouth and oesophagus
Swallowing is divided into three phases. During the oral phase, a bolus of food is forced backwards voluntarily with the tongue until pressure on the pharyngeal wall initiates the swallowing reflex (Fig. 92). This reflex cannot subsequently be interrupted and is coordinated by the swallowing centre in the medulla oblongata. During the pharyngeal phase the soft palate is deflected upwards, sealing off the nasal passages from the pharynx. Muscle contraction pulls the larynx upwards, the glottis closes and the epiglottis deflects the food posteriorly, away from the laryngeal opening. These events protect against aspiration of food into the airways. A travelling wave of constriction (a peristaltic wave) drives the food through the relaxed oesophageal sphincters and along the oesophagus itself in the oesophageal phase of swallowing. The reflex is controlled via both somatic nerves, supplying the striated muscle in the pharynx, larynx and upper oesophagus, and parasympathetic nerves innervating smooth muscle in the mid and lower oesophagus. These nerves stimulate contraction of most of the muscles involved, but nerves also stimulate relaxation of the oesophageal sphincters.
Box 20 Clinical note: Hiatus hernia
Between meals, a functional sphincter at the lower end of the oesophagus protects it from damage caused by entry of gastric acid. This is assisted by the acute angle of entry between the oesophagus and stomach, which produces a functional flap valve (Fig. 93). Protection is diminished in a condition known as hiatus hernia, in which the gastro-oesophageal junction lies above the diaphragm. One of the main symptoms of this condition is pain (heartburn), which is made worse on bending or lifting. This is caused by acid reflux into the lower oesophagus.
Stomach
From the mechanical point of view, the stomach has two functions.
Mechanical activity in the stomach consists of regular, peristaltic waves generated within the stomach muscle at a rate of about 3 min−1. These spread from the body to the antrum, where the strongest contractions occur (Fig. 94). As the contractions reach the pylorus, the pyloric sphincter closes and prevents excessive emptying into the small intestine. So, although each wave forces some chyme into the duodenum, the bulk is mixed back into the body of the stomach.
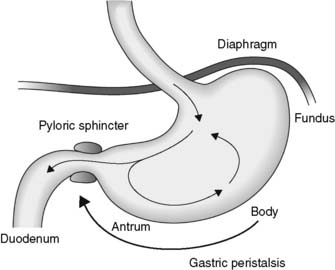
Fig. 94 Mechanical mixing in the stomach is promoted by gastric peristaltic waves and pyloric contraction.
Small intestine
Three patterns of contractile activity are commonly seen in the small intestine.
Large intestine
Mass movements force the colonic contents into the rectum, distending it and thereby initiating the defecation reflex (Fig. 96). The sensory output from stretch receptors in the rectal walls stimulates parasympathetic nerves in the sacral spinal cord which, in turn, increase the contraction of the colon while relaxing the smooth muscle of the internal anal sphincter. Somatic nerves to the striated muscle of the external anal sphincter are inhibited, allowing it to relax. Rectal distension also gives rise to a conscious awareness of the urge to defecate and in the early years of life we learn to respond to this by voluntarily contracting the external anal sphincter. This allows us to control defecation (faecal continence). When appropriate, however, the external sphincter is allowed to relax and defecation proceeds. Expulsion is often assisted by using the abdominal muscles and diaphragm to increase intra-abdominal pressure. This is referred to as abdominal straining.
6.3 Digestion and secretion
Digestion is the term given to the processes whereby the complex molecules in food are broken down into simpler subunits which can be absorbed from the gut and metabolized in the body. This largely depends on a variety of secretions produced by the gastrointestinal tract and associated organs. The whole of the gut is lined by exocrine glands and cells, secreting a total volume of about 7–8 L into the lumen each day. Each secretion is a mixture of aqueous and organic components. The aqueous component is derived from the extracellular fluid surrounding the secretory cells but differs from plasma because of modifications caused by the actions of a variety of ion pumps and carriers. Since different secretory cells contain different pumps, the composition of the aqueous component varies from gland to gland. Also, in glands with ducts, the secretion may be further modified by the duct epithelium (e.g., salivary glands and pancreas; Fig. 98). Digestive secretions usually also have an organic component, often in the form of one or more digestive enzymes manufactured by the secretory cells.
Box 21 Clinical note: Abnormal gastrointestinal motility
Vomiting
Vomiting is a complex set of motor functions coordinated by the vomiting centre in the medulla oblongata. The area postrema in the medulla oblongata is particularly implicated as a chemoreceptor zone which can trigger vomiting. The inputs stimulating this centre, and the resulting motor effects, are summarized in Fig. 97. Vomiting is usually associated with the sensation of nausea and is often preceded by sweating, pallor and an elevated heart rate. These are typical signs of sympathetic nervous activity. Although vomiting is preceded by reverse or antiperistalsis which can drive intestinal contents back into the stomach, the vomiting act is generated by an increase in abdominal pressure, which compresses the relatively relaxed stomach. There is little or no contribution from gastric contraction. In vomiting, NaCl, water and H+ are all lost, so that dehydration and alkalosis may result.
Diarrhoea
Diarrhoea is an increased frequency of defecation. It is commonly caused by:
Gastric secretion
Relevant structure
The inner surface of the stomach is thrown into a series of visible folds, known as rugae, which increase the surface area of the gastric mucosa. The mucosal surface consists of a flat layer of mucus-secreting, columnar epithelial cells, but this is interrupted by multiple gastric pits, each of which leads down into a number of tubular exocrine glands (Fig. 99). The mucosal cells lining the gastric glands are the source of the gastric secretions, with a number of specialist cells producing separate components. The upper one-third to half is lined by mucus-secreting cells. The other two types of exocrine cell, which line the deeper regions of the gastric pits, are the acid-secreting parietal cells (or oxyntic cells) and the smaller, more numerous, cuboidal chief cells, which secrete the enzyme precursor pepsinogen. The gastric mucosa also contains cells which produce chemicals capable of stimulating acid secretion. The G cells produce the peptide hormone gastrin, while the enterochromaffin-like cells secrete histamine, which acts as a paracrine signal stimulating acid secretion in adjacent parietal cells.
Components of gastric secretion
Gastric acid
The parietal cells secrete HCl. This produces a pH of 2–3 within the stomach itself. Active transport of H+ in exchange for K+ by an ATP-dependent proton pump (H+/K+ ATPase) in the cell membrane facing the gastric lumen (the luminal membrane) is the central mechanism involved (Fig. 100). Proton production in the cell depends on dissociation of carbonic acid formed by the reaction of CO2 with H2O in a reaction catalysed by the enzyme carbonic anhydrase (Section 4). This also produces HCO3 −, which is transported out of the parietal cell and into the blood. Because of this, gastric venous blood is more alkaline than systemic arterial blood.
Control of acid secretion
Acid secretion is stimulated in three phases during a meal.
Parietal cell stimulation
Box 22 Clinical note: Peptic ulceration
An ulcer is a break in the mucosal epithelium and when this occurs in the stomach or duodenum it is referred to as a peptic ulcer. One of the main causes is believed to be mucosal damage by gastric acid but infection with the bacterium Helicobacter pylori may also contribute in many cases. Current drug treatments aim to neutralize or decrease acid secretion or increase mucosal protection. This may be combined with antibiotics to eradicate H. pylori, if present.
Pancreatic secretion
The pancreatic secretions pass from the secretory cells, which are arranged in grape-like clumps termed acini, down branch ducts to the main pancreatic duct, which fuses with the common bile duct before opening into the duodenum (Fig. 101). The secretion is alkaline; therefore, it neutralizes the gastric acid entering the duodenum. The balance between H+ secretion in the stomach and HCO3 − secretion by the pancreas ensures that there is normally no overall change in arterial plasma pH following a meal. Pancreatic secretions sampled close to the acini contain the highest concentrations of HCO3 −, but as these secretions pass along the pancreatic ducts there is absorption of HCO3 − in exchange for Cl−, and pH falls.
The pancreatic acini elaborate and secrete enzymes relevant to the digestion of each main food type.
Pancreatic amylase digests carbohydrate under alkaline conditions, releasing oligosaccharides.
Bile
The aqueous component of bile contains NaHCO3. The main organic components are:
This mixture is secreted into the bile canaliculi by the hepatocytes of the liver (see Fig. 113) and then stored and concentrated in the gallbladder (Fig. 101). Following a meal, the gallbladder is stimulated to contract by cholecystokinin, and bile is expelled through the common bile duct into the duodenum.
Bile salts
Bile salts are important in both the digestion and absorption of lipid.
Lipid absorption requires bile salts to stabilize the fatty acids and monoglycerides released by lipases in the form of even smaller molecular aggregates known as micelles. The products of lipid digestion diffuse out of these to be absorbed by the cells of the mucosal epithelium (Section 6.4).
Enterohepatic recirculation of bile salts
Bile salts are reabsorbed in the small intestine, both by passive diffusion and by an active transport system located in the lower end of the ileum (the terminal ileum). Over 80% of the secreted bile salts are absorbed back into the blood in this way and as they pass through the liver they can be resecreted back into bile (Fig. 102). This reabsorption–secretion cycle is referred to as the enterohepatic recirculation of bile salts; it minimizes the amount of bile salt synthesis required.
< div class='tao-gold-member'>
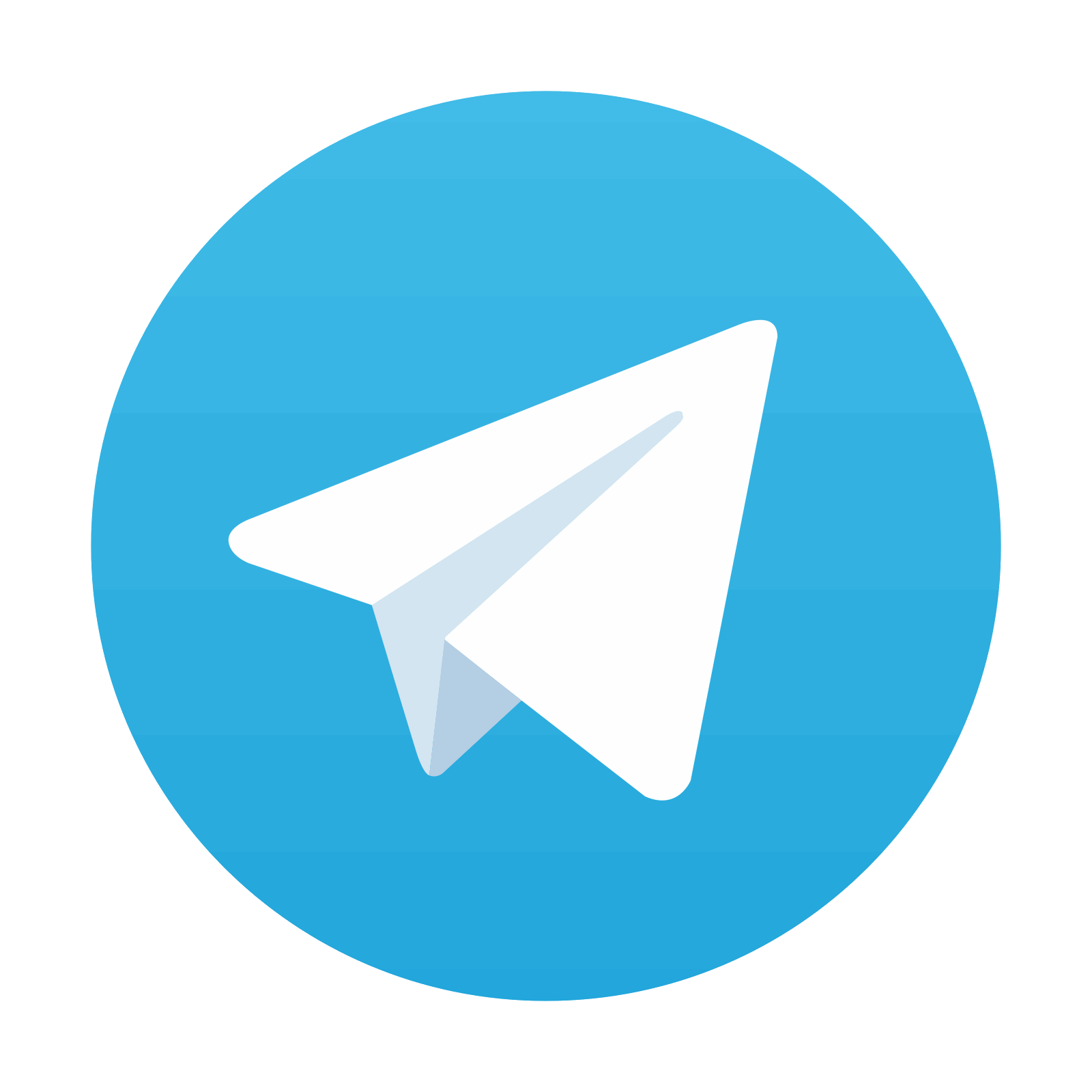
Stay updated, free articles. Join our Telegram channel

Full access? Get Clinical Tree
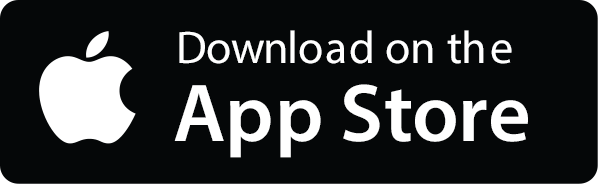
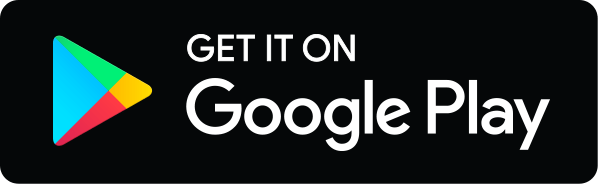