Objectives
- Understand the role of the liver in whole-body homeostasis and the structural features that subserve its functions
- Understand the functions of bile secretion and the anatomy of the biliary system
- Describe the unusual circulatory features of the liver and the relationship of blood flow to bile flow
- Identify the parenchymal and nonparenchymal cell types of the liver, their anatomic relationships, and their respective functions
- Understand pathological conditions where structure and function of the liver and biliary system is compromised
- Describe the role of liver transplantation in treating end-stage liver disease
- Describe the physiological rationale for commonly used tests of liver function, liver injury, and disease prognosis
- Describe the role of liver transplantation in treating end-stage liver disease
Overview of the Liver and Biliary Systems and Their Functions
The liver is the largest gland in the body, and conducts a myriad of vital metabolic and excretory functions. In addition, by virtue of its circulatory relationship to the absorptive surface of the gastrointestinal tract, the liver is the initial site where ingested nutrients, and other substances entering via the gastrointestinal tract, such as drugs and bacterial metabolites, are processed by the body. Thus, the liver is a gate-keeper that can process useful substances while detoxifying orally absorbed substances that are potentially harmful, such as toxic xenobiotics.
The liver contributes in a pivotal way to the biochemical status of the body as a whole. Indeed, a major portion of the discipline of biochemistry is concerned with the chemical reactions that take place in the cell type that makes up the majority of the hepatic mass, the hepatocyte. For this reason, it is beyond the scope of this text to provide a comprehensive analysis of all of the metabolic functions of the liver. Instead, we will focus our discussion on broad categories of metabolic functions of the liver that are relevant to the function of the gastrointestinal system, or are of particular importance to whole-body homeostasis.
First, the liver is an important repository of carbohydrate metabolism. The liver performs four specific functions in this regard: glycogen storage, conversion of galactose and fructose to glucose, gluconeogenesis, and the formation of many important biochemical compounds from the intermediate products of carbohydrate metabolism. Many of the substrates for these reactions derive from the products of carbohydrate digestion and absorption that travel directly to the liver from the gut, as will be described in more detail in Chapter 15. As a consequence, the liver plays a major role in maintaining blood glucose concentrations within normal limits, particularly in the postprandial period. The liver removes excess glucose from the blood and returns it as needed, in a process referred to as the glucose buffer function of the liver. This function is markedly impaired in an individual whose hepatic function has been reduced by disease, resulting in abnormally high postprandial glucose concentrations. However, because the liver also regulates the other aspects of glucose homeostasis described, in liver failure, hypoglycemia is seen. Indeed, hepatectomized animals rapidly develop fatal hypoglycemia.
The liver also contributes in a major way to fat metabolism. While many aspects of lipid biochemistry are common to all cells of the body, others are concentrated in the liver. Specifically, the liver supports an especially high rate of oxidation of fatty acids to supply energy for other body functions. Likewise, the liver converts amino acids and two-carbon fragments derived from carbohydrates to fats that can then be transported to adipose tissue for storage. Finally, the liver synthesizes most of the lipoproteins required by the body, as well as large quantities of cholesterol and phospholipids. Lipoproteins are used to transport various lipids to other sites in the body, whereas phospholipids and cholesterol are important structural components of plasma and intracellular membranes, as well as serving as substrates for the synthesis of other significant biochemicals. Indeed, about 50% of the cholesterol synthesized by the liver is converted to bile acids, which play important roles in the digestion and absorption of lipids, as will be discussed in greater detail in subsequent chapters.
The body also cannot dispense with the liver’s capacity for protein processing for more than a few days. The liver contributes the following important aspects of protein metabolism: deamination of amino acids, formation of urea as a means to dispose of blood ammonia, formation of plasma proteins, and interconversion of various amino acids, as well as conversion of amino acids to other intermediates important in the body. Deamination of amino acids is an initial step in their conversion to carbohydrates or fats, and the liver conducts the vast majority of such reactions. Likewise, the liver can synthesize all of the so-called nonessential amino acids that need not be supplied in the diet in their native form (as will be discussed in more detail in Chapter 15).
The liver also synthesizes proteins that are critical to the circulatory system. With the exception of the immunoglobulins produced by cells of the immune system, the liver provides most of the plasma proteins. Following loss of a large portion of plasma proteins, such as following blood loss, the liver rapidly resynthesizes these such that they can be replaced in a matter of days to weeks. Likewise, the liver is also the major site of synthesis of proteins that contribute to blood clotting, including prothrombin and several clotting factors. These metabolic events depend on the availability of vitamin K.
The liver also serves to detoxify the blood of substances that originate from the gut or elsewhere in the body. In part, this detoxification function is a physical one, in that the liver is highly active in removing particulates from the portal blood, such as small numbers of colonic bacteria that may cross the wall of the intestine under normal circumstances and evade the lymph nodes, but would potentially be harmful if allowed access to the remainder of the circulation. The majority of this “blood cleansing” is provided for by specialized cells related to blood macrophages, known as Kupffer cells. These are highly effective phagocytes that are strategically located to be exposed to the majority of the blood flow originating from the gut.
Other detoxification functions of the liver are biochemical in nature. Hepatocytes express large numbers of cytochrome P450 and other enzymes that can convert xenobiotics, including drugs and toxins, to inactive, less lipophilic metabolites that can subsequently be excreted into the bile and thereby eliminated from the body. Indeed, the oral availability of many drugs is compromised by highly effective removal of such agents arriving in the portal blood, in a process known as first-pass metabolism, of which you will learn more in pharmacology lectures. In general, hepatic metabolism of xenobiotics is divided into phase I metabolism (oxidation, hydroxylation, and other reactions mediated by cytochrome P450s) followed by phase II esterification reactions that link the products to another molecule such as sulfate, glucuronic acid, amino acids, or glutathione. Occasionally, liver metabolism of specific drugs can lead to the production of toxic intermediates, particularly if an overdose is ingested, overwhelming normal pathways of safe metabolism. This scenario accounts for the liver injury that can ensue if patients ingest an overdose of acetaminophen (Tylenol). In addition to the metabolism of xenobiotics, the liver is responsible for the metabolism and excretion of a wide variety of hormones and other endogenous regulators that circulate in the bloodstream, thereby terminating their activity. In particular, the liver is responsible for essentially all of the metabolism of the various steroid hormones such as estrogen, cortisol, and aldosterone. As we saw for glucose homeostasis, liver disease can result in abnormal steroid metabolism and resulting overactivity of the associated hormonal systems.
The renal system is the major contributor to the excretion of water-soluble end products of metabolism in the body. However, the kidneys cannot dispose of lipophilic molecules and heavy metals that circulate in protein-bound form in the plasma, and as such cannot enter the glomerular filtrate. Instead, these are handled by the liver, and excreted in the bile. Hepatocytes express a family of specific transport proteins on their apical and basolateral domains. These recognize high-molecular-weight organic anions and cations, and mediate their vectorial transport into the bile, as will be discussed in more detail in Chapters 11 and 13. In turn, the biliary system is designed to convey these substances out of the liver and into the intestinal lumen, where they undergo little if any reabsorption and thus can be eliminated from the body in the feces.
Engineering Considerations
Based on the foregoing discussion of the major functions fulfilled by the liver, we can begin to consider the design of the organ that is necessary to support these functions. Principally, these include the need for the hepatocyte mass, as well as the Kupffer cells, to be exposed to large volumes of blood, a design that provides for rapid exchange of solutes, including macromolecules, between the various cell types in the liver, and a ductular system to convey excreted substances out of the liver. The liver is also divided into various macroscopic divisions known as lobes, but these are distinguished only by their anatomy and blood supply. With respect to physiologic functions, all lobes of the liver are equivalent.
The liver is unique among all of the organs of the body in that it receives the vast majority of its blood supply in the form of venous blood, especially in the postprandial period. Even at rest, blood flow to the liver via the portal vein is at a rate of 1300 mL/min, compared to only 500 mL/min supplied by the hepatic artery. Moreover, the proportion of blood flow supplied to the liver by the portal vein may increase to almost 90% in the period immediately after a meal. A schematic diagram of the splanchnic circulation is provided in Figure 10–1. Note that the portal vein is the confluence of the splenic, superior mesenteric and inferior mesenteric veins, and thus drains the spleen, stomach, pancreas, small intestine, and colon. It is also notable that while the splanchnic organs represent only 5% of adult body mass, they receive 25% of cardiac output, third in mass/perfusion mismatch behind only the brain and kidneys. Thus, the liver receives a blood supply that contains disproportionate amounts of both oxygen and nutrients compared with its weight.
At a microscopic level, blood perfuses the liver via a series of sinusoids, which are low-resistance cavities that receive blood supply both from branches of the portal vein and from the hepatic artery. At rest, many of these sinusoids are collapsed, whereas as portal blood flow to the liver increases coincident with ingestion and absorption of a meal, sinusoids are gradually recruited to allow the perfusion of the liver with a much greater volume per unit time but only a minimal increase in pressure. The liver also has a distinctive morphologic organization that underpins its functions. This organization is based on the so-called hepatic triad of branches of the portal vein, the hepatic artery, and the bile ducts. Blood flows into a branch of the portal vein in the center of portal areas, which are linked by anastomosing cords of cuboidal hepatocytes to a central venule that in turn drains into the hepatic vein. Branches of the hepatic artery likewise run close to the bile ducts, and likely play an important role in supplying energy and nutrients to the bile duct epithelial cells to support their transport functions. A diagram showing the interrelationships of the various cell types that make up the liver is shown in Figure 10–2.
Figure 10–2.
Arrangement of blood vessels, bile ducts, and hepatocytes to form the liver lobule. Branches of the portal vein and hepatic artery run parallel to bile ducts in the so-called portal triads. Blood percolates through sinusoids arranged between the hepatocytes, to be collected eventually in the central vein. (From Ross MH, Reith EJ. Histology. A Text and Atlas. New York: Harper and Row; 1985.)
The circulatory features of the liver are also notable for the fact that some substances circulate continuously between the liver and intestine, in a circuit known as the enterohepatic circulation. The enterohepatic circulation in fact involves passage of solutes through three different environments—the portal vein and the sinusoids into which it empties, the biliary system, and the intestinal lumen (Figure 10–3). Thus, the requirement for a solute that enters into the enterohepatic circulation is that it be transported into hepatocytes and secreted into bile, then reabsorbed at an appreciable rate (either actively or passively) from the intestinal lumen. Most notably, this occurs for the bile acids that are utilized during intestinal lipid digestion and absorption, of which we will learn more later, but certain drugs and their metabolites may also circulate via this route, altering their pharmacokinetics. The physiological significance of this circuit is that it permits the secretion rate to greatly exceed the synthesis or input rate.
Figure 10–3.
Schematic of the enterohepatic circulation of conjugated bile acids. Bile acids secreted by hepatocytes enter the bile and flow through the biliary system to the duodenum. Conjugated bile acids are selectively reabsorbed in the terminal ileum, and flow through the portal vein back to the liver to be reabsorbed by hepatocytes and resecreted.
The transport and metabolic functions of the liver center predominantly on the cells making up its parenchyma, the hepatocytes (80% of the total cells, approximately 100 billion in an adult human liver). Nevertheless, the nonparenchymal cell types, including stellate cells, sinusoidal endothelial cells, and previously mentioned Kupffer cells, also play vital roles. In this section, we will review the distinctive structures of these cells and the extracellular environments in which they reside, as well as how their properties contribute to the physiological functions of the liver.
Hepatocytes are the metabolic “factories” of the liver, and are responsible for most of its characteristic functions. Hepatocytes are highly specialized polarized epithelial cells. Their apical membranes are in the form of grooves between adjacent cells, known as canaliculi (Figure 10–4). The canaliculi form a continuous network that drains eventually into the bile ductules (Figure 10–5
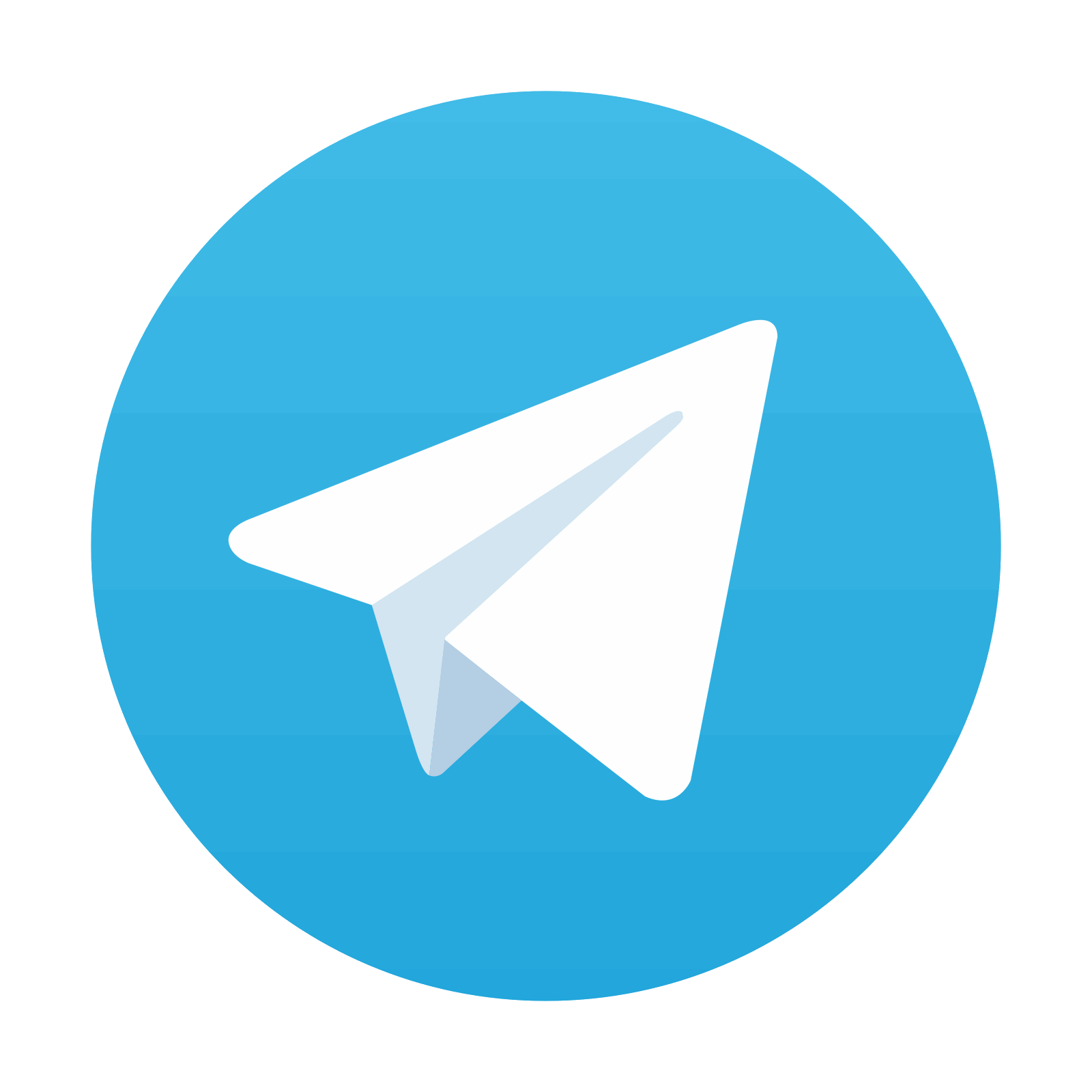
Stay updated, free articles. Join our Telegram channel

Full access? Get Clinical Tree
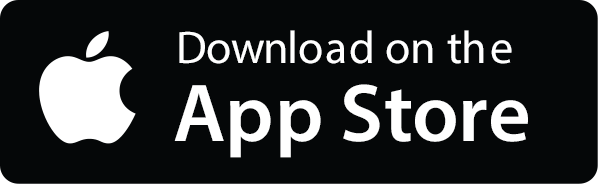
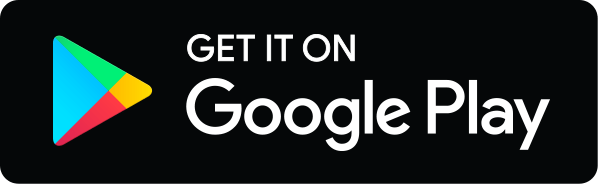
