Eric J. Nestler, MD, PhD
4
Diverse types of chemicals cause addiction. Such drugs share no similarities in chemical structure, and yet they produce similar behavioral syndromes: addiction. The initial protein targets for almost all drugs of abuse are known (Table 4-1). Also, several circuits in the brain, containing these drug targets, have been shown to mediate the addicting actions of drugs of abuse. Most attention has been given to the nucleus accumbens (a part of the ventral striatum) and its dopaminergic input from the ventral tegmental area of the midbrain as key substrates for these drug effects. Other brain regions interact with this circuit, including several regions of the prefrontal cortex, amygdala, hippocampus, and hypothalamus, to name a few.
TABLE 4-1. ACUTE ACTIONS OF SOME DRUGS OF ABUSE
aActivity at μ (and possibly) δ receptors mediates the reinforcing actions of opioids; κ receptors mediate aversive actions.
bCocaine and amphetamine exert analogous actions on serotonergic and noradrenergic systems, which may also contribute to the reinforcing effects of these drugs.
cGi couples D2-like dopamine receptors, and Gs couples D1-like dopamine receptors, both of which are important for dopamine’s reinforcing effects.
dEthanol affects several other ligand-gated channels and, at higher concentrations, voltage-gated channels, as well. In addition, ethanol is reported to influence many other neurotransmitter systems, including serotonergic, opioidergic, and dopaminergic systems. It is not known whether these effects are direct or achieved indirectly via actions on various ligand-gated channels.
eActivity at CB1 receptors mediates the reinforcing actions of cannabinoids; CB2 receptors are expressed predominantly in the periphery but may also be involved in central actions. Endogenous ligands for the CB1 receptor include the arachidonic acid metabolites, anandamide, and 2-arachidonylglycerol.
Data from Nestler EJ. Molecular basis of neural plasticity underlying addiction. Nat Rev Neurosci 2001;2:119–128.
These brain structures form the reward circuitry, which is very old from an evolutionary point of view and which is presumably evolved to mediate an individual’s responses to natural rewards, such as food, sex, and social interaction. Drugs of abuse activate this circuitry, in the absence of natural rewards, with a force and persistence not seen under normal conditions. Over time, repeated drug exposure causes adaptations in the neurons that define this circuitry, which have three major consequences. First, during periods of active drug use or shortly after ceasing drug intake, the ability of natural rewards to activate the reward pathways is diminished, and the individual experiences depressed motivation and mood. Taking more drug is the quickest, easiest way for a person with addiction to feel “normal” again. Second, drug use causes long-lasting memories related to the drug experience, such that even after prolonged periods of withdrawal (months, years), stressful events or exposure to the drug or to drug-associated cues can trigger intense craving, and in many cases relapse, by activating the brain’s reward circuitry. Third, drug use impairs cortical control over subcortical reward-related brain regions, which results in the impulsivity, compulsivity, and corrupted judgment that characterize a state of addiction. Roughly half of the risk for addiction is genetic, although few specific genes that comprise this risk have to date been identified. A great deal of effort is aimed at identifying these specific genes and the mechanisms by which diverse nongenetic factors interact with the genes to influence the development of an addictive disorder.
Addiction should be viewed as distinct from physical dependence, wherein individuals become physically sick when drug administration ceases. Physical dependence per se is neither necessary nor sufficient to cause addiction: some drugs of abuse do not cause appreciable physical dependence, and some medications used in general medicine cause physical dependence but are not addicting (e.g., β-adrenergic antagonists). Moreover, physical dependence and withdrawal syndromes for drugs of abuse and nonabused medications are largely mediated by different central nervous system regions than the brain reward regions, which we know are important for addiction.
We now have very good treatments for the physical dependence and withdrawal syndromes associated with several drugs of abuse, in particular, opiates, alcohol, and nicotine. In contrast, treatment of the core symptoms of addiction—namely, drug craving and relapse to drug use even after prolonged abstinence—has proved much more difficult. Approaches pursued to overcome this challenge can be divided into three major categories: blockade of drug targets, mimicry of drug action, and blockade of the addiction process.
BLOCKADE OF DRUG TARGETS
The most straightforward strategy is to block the drug from getting to its target. Such a treatment agent should have the additional requirement of not affecting that target on its own. The best example of this approach is naltrexone, a long-acting opioid receptor antagonist. In theory, naltrexone is inactive in the absence of an opioid but blocks the ability of opioids to produce their many effects, including addiction. Indeed, naltrexone can be used to treat opioid addiction but has its limitations. Naltrexone is in fact not inactive in the absence of exogenous opioids. This is because the drug blocks the actions of the body’s endogenous opioid peptides (enkephalin, endorphin, and dynorphin); this can cause negative emotional effects (such as depressed mood), which reduce patient compliance. As a result, naltrexone is mostly effective for highly “motivated” addicted individuals whose employment can be used to coerce compliance. Based on animal studies showing that alcohol’s and nicotine’s addicting actions are mediated in part via activation of endogenous opioidergic neurons (Fig. 4-1), naltrexone has been used to treat addiction to these drugs as well. Some efficacy is observed clinically, but the effects of naltrexone are relatively small in magnitude and effective for a subset of patients only.
FIGURE 4-1. General strategies used to treat drug addiction or associated physical withdrawal syndromes. A dendritic spine of a nucleus accumbens (NAc) neuron and its innervation by terminals of glutamatergic (Glu), dopaminergic (DA), and opioidergic (Op) neurons are shown. 1: One approach is to block the ability of a drug to reach its initial protein target: for example, naltrexone’s antagonism of opioid receptors (OR) or a hypothetical drug that interferes with cocaine’s actions on the dopamine transporter. Not depicted is the use of immunologic methods (such as a cocaine or nicotine vaccine) to prevent a drug from entering the brain. 2: A second approach is to mimic drug action: for example, sustained activation of OR by methadone, or activation of DA receptors (DAR) by various agonists or partial agonists. 3: A third approach is to influence the process of addiction: for example, via perturbation of Glu receptors (AMPA, NMDA, metabotropic receptors) or a host of postreceptor signaling proteins (such as those involved in the cAMP, calcium, and MAP kinase pathways and in the regulation of gene expression—ΔFosB, CREB, or NFκB) that have been implicated in addiction. (From Nestler EJ. Molecular basis of neural plasticity underlying addiction. Nat Rev Neurosci 2001;2:119–128; Hyman SE, Malenka RC, Nestler EJ. Neural mechanisms of addiction: the role of reward-related learning and memory. Annu Rev Neurosci 2006;29:565–598; Robison AJ, Nestler EJ. Transcriptional and epigenetic mechanisms of addiction. Nat Rev Neurosci 2011;12:623–637.)
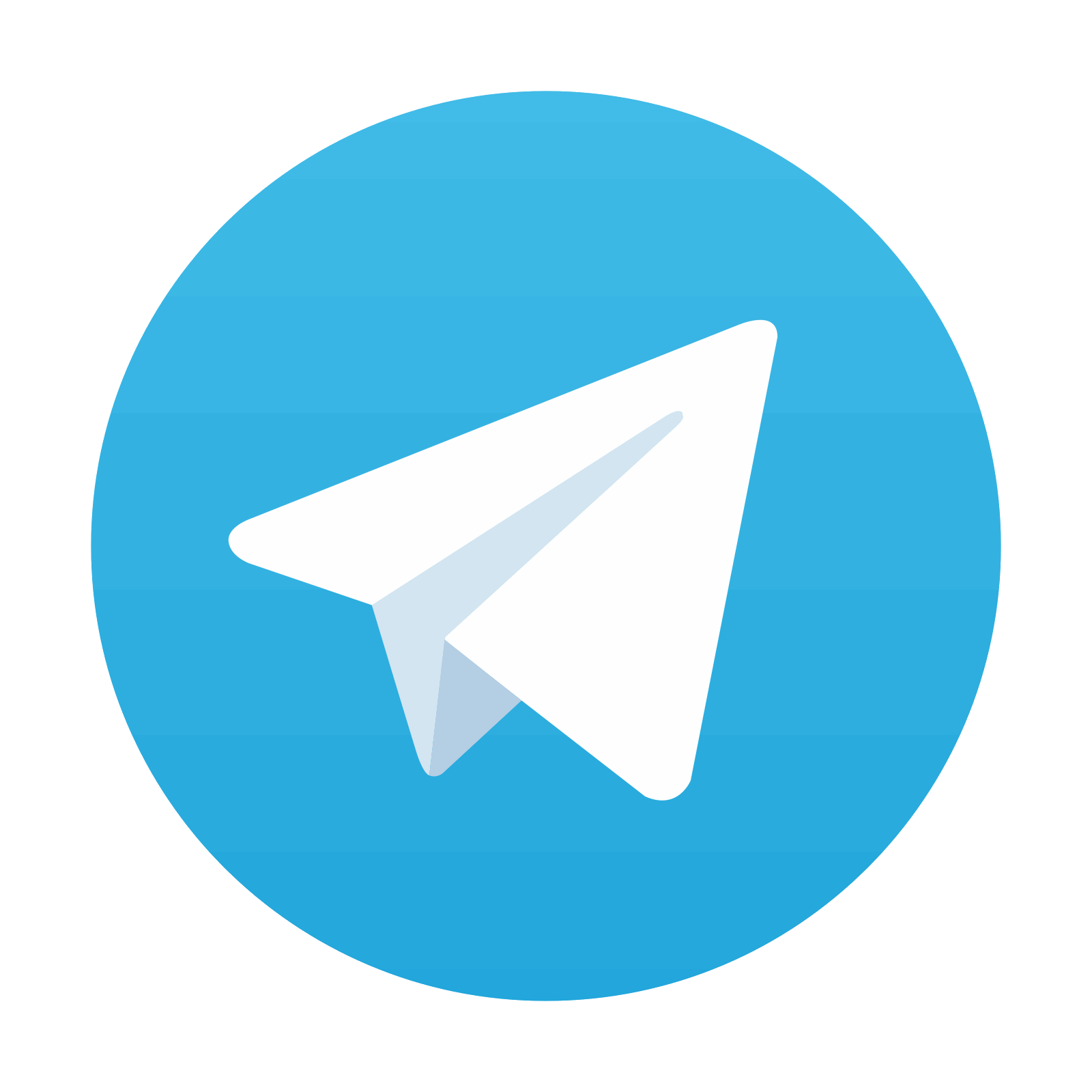
Stay updated, free articles. Join our Telegram channel

Full access? Get Clinical Tree
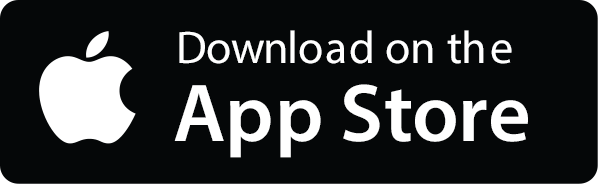
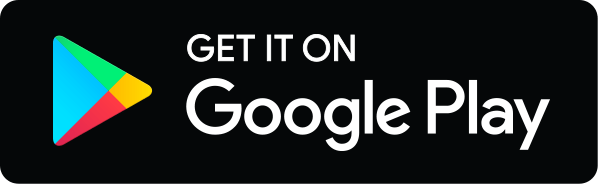