Follicular Adenoma
Yuri E. Nikiforov
N. Paul Ohori
DEFINITION
Follicular adenoma is a benign, encapsulated, noninvasive tumor originating from thyroid follicular cells. When used without additional qualifiers, this term typically refers to a conventional type of follicular adenoma. The variants of follicular adenoma are designated using additional adjectives or other qualifiers. Oncocytic follicular adenoma is the most common variant of follicular adenoma.
INCIDENCE AND EPIDEMIOLOGY
The true incidence of follicular adenomas is difficult to establish with high accuracy because they cannot be reliably discriminated from solitary hyperplasic nodules based on clinical parameters or fine needle aspiration (FNA) cytology. The incidence of adenomas in adults can be estimated at 3% to 5% based on autopsy series.1,2,3 This estimate correlates well with the prevalence of thyroid nodules detected by palpation. Palpable thyroid nodules are found in 3% to 7% of adults living in iodine-sufficient areas, and three-fourths of those are solitary nodules on palpation and may represent adenomas.4,5 Follicular adenomas more frequently affect females, with the female-to-male ratio ranging from 4:1 to 5:1. They are found on all age groups, although most patients present during the fifth and sixth decades of life.
ETIOLOGIC FACTORS
Most follicular adenomas are sporadic, although some may arise as manifestations of known inherited syndromes. The risk factors implicated in the development of sporadic adenomas include radiation exposure and iodine deficiency. Exposure to X-ray or γ-radiation during childhood and adolescence increases the risk of follicular adenomas up to 15-fold.6,7,8 The risk is radiation dose dependent and exists after a dose as small as 0.25 Gy. Most follicular adenomas develop 10 to 15 years after exposure, but elevated risk persists for at least 50 years. Follicular adenomas developing after radiation exposure are usually solitary and reveal conventional type histology, although oncocytic follicular adenomas may also be seen.8
Iodine deficiency is another risk factor that correlates with higher incidence of benign thyroid nodules including adenomas. Palpable thyroid nodules are two to three times more common in the areas of low iodine consumption as compared with iodine-sufficient areas, and a significant proportion of those are adenomas.9,10 In support of this association, follicular adenomas can be induced in experimental animals by administration of low iodine diet or drugs that interfere with iodine uptake and metabolism in thyroid cells.11,12 The exact mechanism of how iodine deficiency promotes adenoma formation is not clear, but it probably involves the increase in thyroid-stimulating hormone (TSH) levels that stimulate proliferation of thyroid cells.
A cholesterol-lowering agent, 3-hydroxymethylglutaryl coenzyme A (HMG CoA) 1 reductase inhibitor simvastatin, increases incidence of thyroid adenomas in female rats.13 This is likely because of enhanced liver clearance of thyroid hormone, which is expected to cause elevation in TSH levels. It is unclear whether similar side effects occur in humans. To date, one case of thyroid follicular adenoma with oncocytic features has been reported in a patient treated with simvastatin.14
Follicular adenomas develop in patients affected by inherited diseases such as Cowden disease and Carney complex. Cowden disease (multiple hamartoma syndrome) is caused by germ line mutation of the phosphatase and tensin homolog (PTEN) tumor suppressor gene located on chromosome 10q23.15,16,17 PTEN codes for a dual specificity phosphatase that functions as a negative regulator of the phosphatidylinositol 3-kinase (PI3K)/AKT signaling pathway responsible for cell survival and proliferation (illustrated in Fig. 10.5, Chapter 10). The inherited mutation, followed by inactivation of the gene on the second allele through a somatic event, results in the loss of PTEN function and chronic stimulation of AKT and its downstream targets. Follicular adenomas in the affected patients develop at young age and are almost always multiple and bilateral. They are typically follicular adenomas of conventional type, although oncocytic and clear cell adenomas and adenolipoma have also been reported in these patients.18 Carney complex is another rare autosomal dominant disease caused by germ line mutations in the PRKARIA gene on chromosome 17q22-24 and in other still unknown genes.19 PRKARIA encodes the regulatory 1-α subunit of the protein kinase A that mediates the cyclic AMP (cAMP) signaling. Patients affected by this disease frequently develop thyroid adenomas, which are often multiple and may show oncocytic features.20
PATHOGENESIS AND MOLECULAR GENETICS
Clonality
Follicular adenomas are true neoplasms and therefore expected to have a monoclonal origin, that is, arise from a single cell. This is opposed to nonneoplastic lesions such as hyperplastic nodules, which have a polyclonal origin. Clonality of a lesion can be assessed in female tissue based on the randomness of inactivation of two × chromosomes. This can be achieved by several assays, including the PCR-based human androgen receptor gene (HUMARA) assay. The principles and limitations of clonality studies are discussed in more detail in Chapter 20. Most studies have confirmed that follicular adenomas diagnosed based on common morphologic criteria are monoclonal, which is consistent with their neoplastic nature.21,22 Another way to establish clonal origin of a given tumor is through the detection of a mutation (such as RAS mutation) or a distinct cytogenetic abnormality (such as trisomy for chromosome 7). The presence of any of these molecular alterations detected in most cells within the lesion indicates its origin from a single cell, that is, its monoclonal origin.
Cytogenetic Abnormalities
Cytogenetically detectable chromosomal changes are found in 30% to 45% of follicular adenomas.23,24 Most of those are numerical chromosome changes, typically gains, which involve one or several chromosomes. Chromosome 7 is most frequently affected with gains of one or more copies, followed by gains of chromosomes 12 and 5. These numerical chromosome changes can be visualized by G-band karyotyping, comparative genomic hybridization, or fluorescence in situ hybridization (FISH). By FISH, chromosome 7 gains are seen in ˜15% of conventional follicular adenomas (typically trisomy 7) and in ˜45% of oncocytic follicular adenomas (typically tetrasomy 7) (Fig. 8.1).25
The second most common cytogenetic alternation, found in approximately 10% of follicular adenomas, is a group of translocations involving chromosomal loci on 19q13 and 2p21, which are fused with various partners. These adenomas reveal no trisomy 7 or other numerical chromosomal changes and represent a cytogenetically distinct tumor group.23,26 The gene participating in the fusion on 2p21 has been identified as THADA.27 It is likely to be involved in the death receptor pathway, and its disruption by translocation may affect apoptosis.28 A putative gene on 19q13 targeted by translocation has been identified as ZNF331.29 The translocation apparently results in aberrant transcription of this gene, although how it promotes tumorigenesis remains clear.
DNA Ploidy
Change in DNA ploidy typically correlates with losses and gains of whole chromosomes. Overall, 20% to 30% of follicular adenomas have aneuploid cell populations.30,31,32 Aneuploidy is more common in adenomas with high cellularity, particularly in those with a solid/trabecular growth pattern, where it can be found in >50% of tumors.33,34 The frequency of aneuploidy tends to increase from hyperplastic nodules to follicular adenomas and then to follicular carcinomas, although a significant overlap between these groups exists.30,31
Loss of Heterozygosity
Loss of heterozygosity (LOH) results from deletions of small or large chromosomal regions and is an important genetic feature of neoplastic growth. Regions that are consistently deleted in tumors typically correspond to the location of tumor suppressor genes whose loss of function is required for tumor progression. Follicular adenomas have on average a 6% rate of LOH per chromosome arm, as compared with a 20% rate in follicular carcinomas.35 The frequency of LOH tends to be higher in follicular adenomas of oncocytic type.36 The regions most commonly deleted in follicular adenomas are on chromosomes 1q, 3q, 9p, 10q, 13q, and 18q.35,36,37 LOH in those regions is found in 10% to 30% of adenomas. On 10q, the loss frequently affects the 10q22-24 region where the PTEN tumor suppression gene resides. Another potential target for deletion in this region is the MINPP1 gene, which is located in proximity to PTEN and encodes a protein with similar functions.38,39
Somatic Mutations
RAS Mutations
Activating point mutations of the RAS genes are common in follicular adenomas. They may involve codons 12, 13, or 61 of the three RAS genes (NRAS, KRAS, and HRAS), although NRAS codon 61 and HRAS codon 61 mutations are by far the most prevalent. RAS mutations are found in approximately 30% of conventional type follicular adenomas.40,41,42 The frequency may be higher in follicular adenomas with a microfollicular growth pattern.40 One study has shown a significantly higher incidence of RAS mutations in adenomas from an area of iodine deficiency as compared with an iodine-rich region.43 RAS mutations appear to be less frequent in oncocytic adenomas, although only few reported studies have examined this tumor type.44,45 This is also supported by our experience.46 In a series of 45 oncocytic and conventional type follicular adenomas, we observed RAS mutations in 7% of oncocytic adenomas as compared with 37% of conventional type adenomas.
RAS mutation results in chronic stimulation of various downstream signaling pathways, most notably the mitogen-activated protein kinase (MAPK) pathway and PI3K/AKT pathway, by the mutant RAS protein. This mutation is likely to be an initiating event in the development of follicular adenomas. This is supported by experiments on cultured human thyroid cells and by animal studies. RAS activation in cultured cells induces self-limited proliferation with formation of well-demarcated, differentiated cell colonies, recapitulating the growth of follicular adenomas.47 Expression of mutant RAS in transgenic mice leads to the development of follicular adenomas and follicular carcinomas.48,49
TSHR and Gsα Mutations
Somatic mutations in the TSH receptor (TSHR) and α subunit of the stimulatory G protein (Gsα) genes play a causal role in the development of hyperfunctioning (toxic) thyroid adenomas. These mutations lead to chronic stimulation of the cAMP signaling pathway, which is involved in the regulation of all major aspects of thyroid growth and function by TSH (Fig. 8.2). In hyperfunctioning adenomas, point mutations of the TSHR gene are found in 50% to 80% and of the Gsα genes in ˜5% of cases.50,51 Mutations cluster in the functionally important regions of both genes. In TSHR, they are primarily located in the transmembrane domain involved in interaction with the Gsα protein and in the region of the extracellular domain responsible for the receptor binding to TSH.52 In the Gsα gene, mutations affect codons 201 and 227 and result in the inhibition of the intrinsic GTPase activity, so that the protein is permanently locked in the active form. These mutations invariably lead to the development of nodules with elevated thyroid function and increased iodine uptake. The causal role of these mutations in the development of hyperfunctioning thyroid nodules has been confirmed in transgenic mice carrying the mutant Gsα.53 The animals develop thyroid nodules composed of cells with an elevated cAMP level and high uptake of radioiodine.
Other Mutations
Somatic mutations affecting the PI3K/PTEN/AKT pathway are rare in follicular adenomas. Mutations in the PIK3CA gene, coding for the subunit of PI3K, occur in ˜5% of tumors.54 No somatic mutations in the PTEN gene have been identified so far, despite the known role of PTEN germ line mutations in the development of thyroid adenomas in a setting of Cowden disease.
PAX8/PPARγ rearrangement, a feature of thyroid follicular carcinomas, is found in about 8% of tumors histologically diagnosed as follicular adenoma.55,56,57,58 Many of these tumors are cellular and have thick capsule but fail to demonstrate invasion.57 It remains to be determined whether the PAX8/PPARγ positive adenomas are actually follicular carcinomas in situ.
A single case of follicular adenoma carrying BRAF K601E mutation has been reported.59
Pathogenesis of Oncocytic Tumors
Oncocytic follicular adenomas are composed of cells with distinct cytoplasmic granularity because of accumulation of a large number of frequently abnormal mitochondria. The cause of the mitochondrial changes and their relationship to the neoplastic process are not fully understood. It remains unclear whether changes in the number and morphology of mitochondria are driven by specific mutations or whether they simply represent a secondary, compensatory change in the cell organelles.60,61,62 Existing evidence supports each of the possibilities, and it is likely that the oncocytic cell appearance may develop through both pathways. In one pathway, tumors would be initiated and driven by the same oncogenes as nononcocytic follicular tumors, such as by mutant RAS, and would develop oncocytic features secondarily during the process of tumor progression. The increased replication of mitochondria in this setting may be a result of growth stimulation by cytokines and other mediators released from the inflammatory cells frequently seen in some tumors or a compensatory reaction to the functional deficiency of the existing abnormal mitochondria. These oncocytic tumors are expected to demonstrate either fully or partially developed oncocytic features and not to differ substantially in their biologic properties from nononcocytic tumors. In the other pathway, tumors would be driven by a specific somatic mutation that initiates tumorigenesis and also directly leads to the increased production of mitochondria. These tumors would have a uniform oncocytic appearance throughout and exhibit distinct biologic characteristics. It is likely that at least some oncocytic tumors develop after this pathway, although no specific mutations have been identified till date. Somatic mutations in mitochondrial DNA (mtDNA), including deletions and frameshift and missense point mutations, are frequently found in many oncocytic tumors.63,64 These mutations predominantly affect the mitochondrial complex I genes and are predicted to disrupt gene function, although their role in tumor initiation remains unclear. This is because a high mutation rate is a general feature of mtDNA, as these mutations are also seen in nonneoplastic oncocytic cells and in nononcocytic tumors.60,65
CLINICAL PRESENTATION AND IMAGING
Thyroid adenomas typically present as a painless thyroid nodule incidentally discovered by palpation or during thyroid ultrasound performed for other reasons. Most adenomas are asymptomatic, although tumors of large size may cause difficulty in swallowing and other local symptoms. They grow very slowly, and some patients seek medical attention many years after discovering the nodule. Occasionally, bleeding into the tumor may occur and cause sudden pain, tenderness, and increase in nodule size. It may occur spontaneously or after vigorous neck palpation or FNA.
On palpation, adenomas are usually felt as a discrete mass that is not fixed to the neck and moves with the thyroid. Most patients are euthyroid. By radionuclide scan, adenomas typically appear as “cold” nodules because they concentrate radioiodine or other tracers less avidly than adjacent thyroid parenchyma (Fig. 8.3A). A small
subset of adenomas are hyperfunctional and present with variably prominent symptoms of hyperthyroidism. Overt thyrotoxicosis, however, is rare, and many patients reveal subclinical hyperthyroidism manifested by the decreased serum TSH level and normal thyroid hormone levels. TSH suppression in these patients is a result of the negative feedback regulation of TSH secretion because of excess thyroid hormone production by the nodule. Hyperfunctioning adenomas appear “hot” on imaging in comparison with the adjacent thyroid tissue that is hypofunctional because of deprivation of TSH stimulation (Fig. 8.3B). On ultrasound examination, follicular adenoma is typically seen as a solid, homogeneous mass that may be hyperechoic, isoechoic, or hypoechoic as compared with the surrounding thyroid tissue (Fig. 8.4, top). The margins of the nodule are well defined and smooth. A peripheral hypoechoic halo that represents a fibrous capsule may be seen. Blood flow can be visualized by color Doppler ultrasound (Fig. 8.4 bottom). Low blood flow is more suggestive of a benign nodule, although no ultrasound features allow for a reliable diagnosis of adenoma.
subset of adenomas are hyperfunctional and present with variably prominent symptoms of hyperthyroidism. Overt thyrotoxicosis, however, is rare, and many patients reveal subclinical hyperthyroidism manifested by the decreased serum TSH level and normal thyroid hormone levels. TSH suppression in these patients is a result of the negative feedback regulation of TSH secretion because of excess thyroid hormone production by the nodule. Hyperfunctioning adenomas appear “hot” on imaging in comparison with the adjacent thyroid tissue that is hypofunctional because of deprivation of TSH stimulation (Fig. 8.3B). On ultrasound examination, follicular adenoma is typically seen as a solid, homogeneous mass that may be hyperechoic, isoechoic, or hypoechoic as compared with the surrounding thyroid tissue (Fig. 8.4, top). The margins of the nodule are well defined and smooth. A peripheral hypoechoic halo that represents a fibrous capsule may be seen. Blood flow can be visualized by color Doppler ultrasound (Fig. 8.4 bottom). Low blood flow is more suggestive of a benign nodule, although no ultrasound features allow for a reliable diagnosis of adenoma.
Most adenomas that present as a solitary, “cold” nodule undergo FNA. Because FNA cytology cannot discriminate between follicular adenoma and follicular carcinoma, the patients are typically referred for surgery.
GROSS FEATURES
Macroscopically, most follicular adenomas are solitary nodules that are clearly demarcated from the surrounding thyroid tissue by a well-formed fibrous capsule (Fig. 8.5A). Multiple adenomas are rare and should raise the possibility of an inherited disease. Adenomas usually have a round to ovoid shape. The size is highly variable and is typically between 1 and 3 cm. The color closely reflects the cellularity of tumors. Densely cellular, solid/trabecular adenomas have light, whitish to gray appearance, whereas colloid-rich adenomas are tan to brown in color. Oncocytic adenomas have characteristic mahogany brown color. Typical adenoma has rubbery consistency and a fleshy, homogeneous solid cut surface. Secondary changes may occur spontaneously or be precipitated by an FNA procedure and manifest as hemorrhage, infarction, cystic degeneration, fibrosis, or calcification (Fig. 8.5B). Oncocytic follicular adenomas are more prone to extensive hemorrhage and infarction.
Gross examination of surgically removed thyroid samples that contain an encapsulated solitary nodule requires careful inspection of the capsule in search for the areas of irregularity or overt
invasion. Unless the nodule is very large, the entire peripheral zone of the nodule surrounding the capsule should be submitted for microscopic examination to rule out tumor invasion. The principles and details of gross examination are described in Chapter 19.
invasion. Unless the nodule is very large, the entire peripheral zone of the nodule surrounding the capsule should be submitted for microscopic examination to rule out tumor invasion. The principles and details of gross examination are described in Chapter 19.
MICROSCOPIC FEATURES
On microscopic examination, follicular adenoma appears as an encapsulated follicular lesion with architectural and cytologic features that are distinct from the surrounding thyroid parenchyma (Fig. 8.6). The capsule is complete and typically thin to moderately thick. The capsule is composed of parallel layers of collagen occasionally penetrated by smooth muscle-walled blood vessels (Fig. 8.7). Myxoid degeneration of collagen fibers and blood vessel walls within the capsule may be seen. Capsular calcification is sometimes found. Small aggregates of entrapped follicular cells may be seen within the capsule and should not be misinterpreted as evidence for invasion. A very thick capsule should raise the suspicion of carcinoma and prompt particularly
thorough evaluation for invasion. However, in the absence of capsular or vascular invasion, the diagnosis of adenoma is rendered.
thorough evaluation for invasion. However, in the absence of capsular or vascular invasion, the diagnosis of adenoma is rendered.
![]() FIGURE 8.6. Low-power view of follicular adenoma. Note a distinct lesion with a homogeneous microfollicular appearance separated from normal thyroid tissue by a thin capsule. |
![]() FIGURE 8.7. This follicular adenoma has a thick capsule that shows a blood vessel with myxoid changes in the wall. |
Follicular adenomas display various microscopic architectural patterns. Most commonly seen patterns are solid, trabecular, microfollicular, normofollicular, and macrofollicular (Fig. 8.8). The solid/trabecular pattern adenomas are sometimes designated as “embryonal” because they have an appearance of an early developmental stage of the thyroid. Those tumors are very cellular and depleted of colloid. Microfollicular adenomas are composed of small, round follicles and are referred to as “fetal” adenomas because of their resemblance to the thyroid tissue during the late fetal period of development. Small amounts of colloid are seen within the lumen of follicles. Normofollicular adenomas are composed of neoplastic follicles of size comparable with normal thyroid gland follicles. Macrofollicular adenomas have large follicles filled with colloid. Other growth pattern, such as nested/insular and papillary, may occasionally be found. One pattern usually predominates within an individual tumor nodule, although it is rarely pure and is typically seen in combination with other growth patterns. The patterns bare no diagnostic significance and do not have to be mentioned in the diagnostic line.
Inspissated colloid found in the lumen of some follicles may occasionally undergo calcification and show concentric laminations resembling psammoma bodies (Fig. 8.9). These pseudopsammoma bodies are more frequently seen in oncocytic adenomas and distinguished from true psammoma bodies based on their location within the lumen of follicles. In contrast, psammoma bodies in papillary carcinomas reside in the tumor stroma.
Adenomas are typically composed of uniform cells of cuboid or polygonal shape (Fig. 8.10A). The cells are tall in hyperfunctioning adenomas. The cytoplasm is moderately abundant, pale eosinophilic to amphophilic. Cell borders are well defined. The nuclei are basally located and relatively evenly spaced along the basement membrane. They are typically small, round, uniform, hyperchromatic or normochromatic. The coarse or vesicular chromatin is rarely seen. The cells of conventional type adenomas have one or several inconspicuous, eccentrically located nucleoli. Some variation in the nuclear size, shape, and chromatin texture may be seen, with single cells showing very large, irregular, hyperchromatic nuclei (Fig. 8.10B). The most extreme manifestations of nuclear atypia are observed in follicular adenomas with bizarre nuclei, as discussed later in the chapter. Severe nuclear atypia affecting random cells is occasionally seen in follicular adenomas and other benign thyroid nodules and is not a feature of malignancy. Mitotic figures are rarely found except in the areas of recent aspiration and other regenerative changes. Finding several mitoses is rare in adenomas and requires careful evaluation for malignancy.
The stroma is typically scant. Some adenomas have abundant edematous or hyalinized stroma that is more pronounced in the central portion of the nodule (Fig. 8.11A). Thick fibrotic bands are rarely seen, and their presence raises suspicion for the follicular variant of papillary carcinoma. Adenomas are rich in delicate capillary vessels, which are not readily identifiable on routine hematoxylin and eosin (H&E) staining. Secondary changes include hemorrhage, edema, ischemic necrosis, cystic degeneration, fibrosis and hyalinization, calcification, and osseous or cartilaginous metaplasia (Fig. 8.11). Squamous metaplasia is rarely seen. The FNA-induced injury may mimic invasion or lead to other change that may be mistaken for malignancy. The term worrisome histologic alterations following fine needle aspiration of the thyroid or WHAFFT has been proposed for these changes,66 which are described in more detail in Chapter 6.
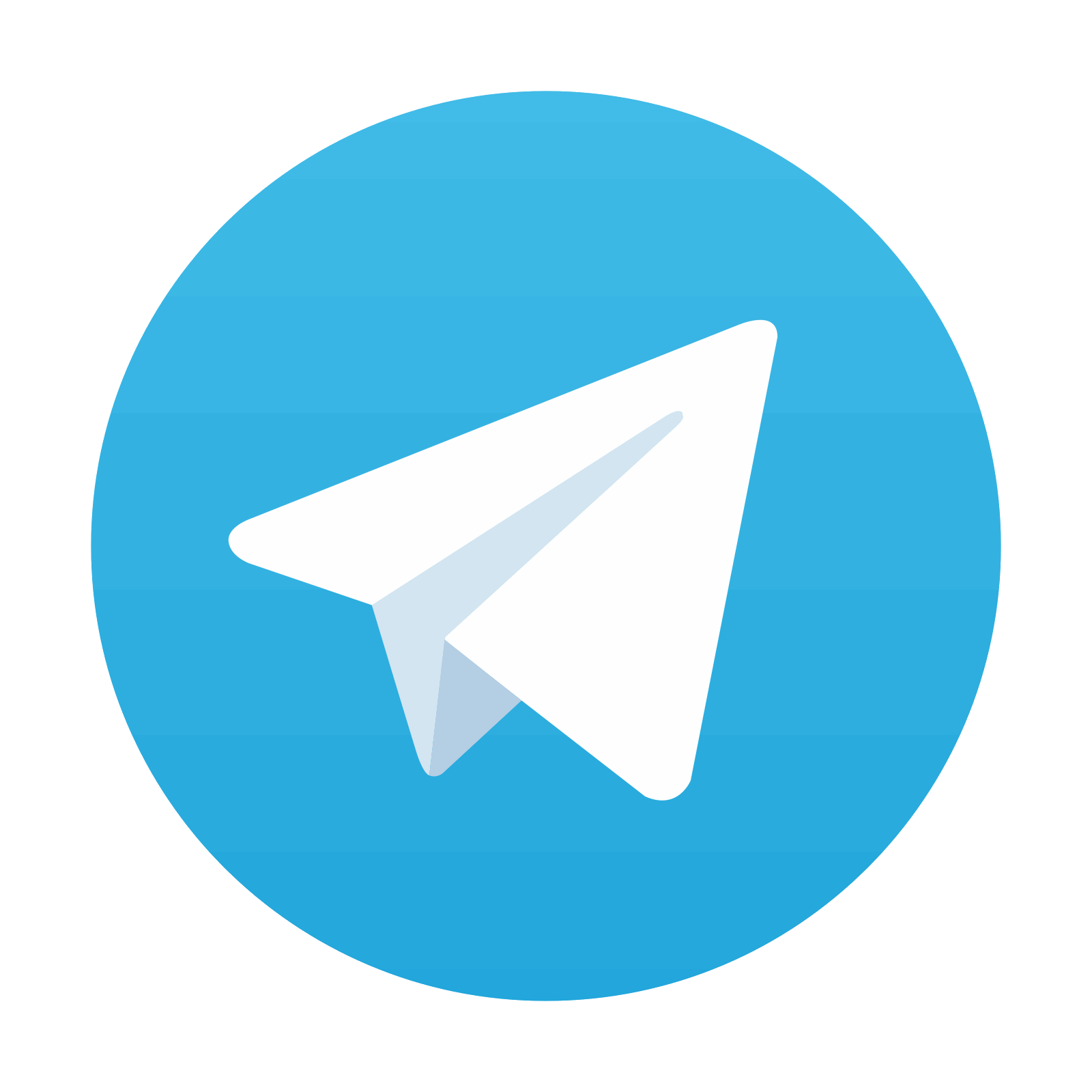
Stay updated, free articles. Join our Telegram channel

Full access? Get Clinical Tree
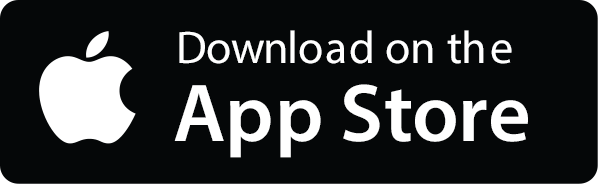
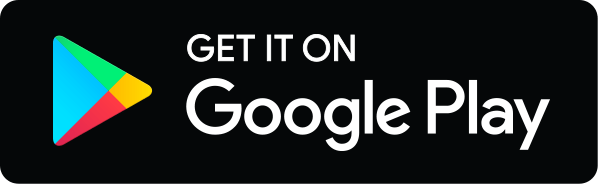