Fluid and Electrolyte and Acid-Base Balance
The maintenance of water, electrolyte, and acid-base balance is a daily requirement of all living organisms. In humans, water and electrolyte intake and output are regulated through hormonal and neural interactions that overlie behavioral and dietary practices. As for acid-base balance, most metabolic processes occurring in the body result in the production of acid. It is essential that these acids be removed from the body. The removal of carbon dioxide is performed by the lungs. The removal of other, nonvolatile (nongaseous) acids is performed by the kidneys. The lungs and the kidneys, together with various buffer systems in the body, maintain plasma acid concentration within narrow, physiologic limits.
● Physiologic Concepts
WATER BALANCE
Water makes up approximately 60% of total body weight. Of this amount, two thirds (66%) is intracellular and one third (33%) is extracellular (i.e., in the plasma or interstitial space) (Fig. 19-1). Water is essential to life because of the role it plays in energy production, the maintenance of osmotic pressure, and the transport of substances in the body and across cell membranes. Maintaining the correct balance between water intake and output is critically important; if an individual becomes overhydrated, dilution of plasma electrolytes and solutes, cell swelling, and
possibly death can result. Likewise, if one becomes severely dehydrated, plasma solute and electrolyte overconcentration and cell shrinkage occur and can lead to nervous system dysfunction and death. Stimuli to take in fluid may be physiologic or social. Outputs vary, related to ambient temperature, exercise, and clothing. Ultimately, the thirst drive centered in the hypothalamus and the output of urine by the kidneys maintain the harmony between intake and output.
possibly death can result. Likewise, if one becomes severely dehydrated, plasma solute and electrolyte overconcentration and cell shrinkage occur and can lead to nervous system dysfunction and death. Stimuli to take in fluid may be physiologic or social. Outputs vary, related to ambient temperature, exercise, and clothing. Ultimately, the thirst drive centered in the hypothalamus and the output of urine by the kidneys maintain the harmony between intake and output.
Normal Water Intake and Output
Adults ingest between 1.5 and 2.5 L of fluid a day. Another 300 to 400 mL are produced daily via metabolic reactions. Daily outputs exactly balance these inputs in healthy individuals: 1.0 to 2.0 L excreted in urine, 100 mL excreted in the feces, 50 mL excreted in sweat, and approximately 1000 mL excreted through exhalation of air and surface evaporation. Insensible fluid loss refers to the loss of fluid from respiration, evaporation, sweat, and other drainage. As highlighted in Figure 19-2, fluid ingestion and urine excretion are the only variables under the precise control of neural and hormonal stimuli.
Control of Fluid Ingestion
Although the amount of fluid we drink each day is influenced by dietary and social influences, the ultimate control over whether we ingest an adequate
amount of fluid is exerted by the thirst center located in the hypothalamus at the level of the third ventricle. Thirst is sensed by hypothalamic osmoreceptors that increase their rate of firing with increased plasma osmolarity (i.e., decreased water concentration). When activated, these osmoreceptors, along with neighboring cells that sense blood pressure, signal the hypothalamus to increase the release of antidiuretic hormone (ADH, also called vasopressin) from the posterior pituitary gland (see Chapters 9, 13, and 18). ADH has two major effects. The first and most important is to increase the permeability of the renal collecting ducts of the kidney to water (see Chapter 18). Increased permeability of the collecting ducts allows water to be reabsorbed out of the urine back into the blood, thereby diluting the plasma back toward normal (and so reducing the stimulus for ADH release). The second effect of ADH is to act as a pressor, an agent causing a rise in blood pressure; its other name is vasopressin. In this role, ADH causes a constriction of vascular smooth muscle and an increase in blood pressure (see Chapter 13). The increase in blood pressure also reduces the stimulus for continued release of ADH.
amount of fluid is exerted by the thirst center located in the hypothalamus at the level of the third ventricle. Thirst is sensed by hypothalamic osmoreceptors that increase their rate of firing with increased plasma osmolarity (i.e., decreased water concentration). When activated, these osmoreceptors, along with neighboring cells that sense blood pressure, signal the hypothalamus to increase the release of antidiuretic hormone (ADH, also called vasopressin) from the posterior pituitary gland (see Chapters 9, 13, and 18). ADH has two major effects. The first and most important is to increase the permeability of the renal collecting ducts of the kidney to water (see Chapter 18). Increased permeability of the collecting ducts allows water to be reabsorbed out of the urine back into the blood, thereby diluting the plasma back toward normal (and so reducing the stimulus for ADH release). The second effect of ADH is to act as a pressor, an agent causing a rise in blood pressure; its other name is vasopressin. In this role, ADH causes a constriction of vascular smooth muscle and an increase in blood pressure (see Chapter 13). The increase in blood pressure also reduces the stimulus for continued release of ADH.
A second hormone, angiotensin II, also has a direct effect on the cells of the hypothalamus to increase the sensation of thirst. It, too, is an important dipsogenic (thirst-stimulating) hormone.
ELECTROLYTE BALANCE
Sodium
As the major extracellular ion in the body, sodium is responsible in large part for determining plasma osmolality and is also important in maintaining membrane potential and neural conductance. The regulation of plasma sodium is mainly achieved by the kidney, which freely filters and then reabsorbs at least 98% of filtered sodium. The remaining 2% is reabsorbed or excreted into the urine, depending on the presence or absence of the hormone aldosterone; increased aldosterone increases sodium reabsorption back into the blood by acting at the level of the distal tubule of the kidney (see Chapter 18). Low levels of aldosterone lead to the excretion of all or some of the final 2% of sodium in the urine.
Aldosterone is secreted from the adrenal cortex following stimulation by the hormone angiotensin II. A brief summary of this hormonal system, presented in detail in Chapter 18, is as follows: Angiotensin II is produced after a cascade of reactions initiated by the hormone renin released from the juxtaglomerular cells of the kidney. Renin is released in response to low plasma sodium and low blood pressure. As a result of an increase in angiotensin II levels, aldosterone release is stimulated, and sodium balance and blood pressure return toward normal; this process is an excellent example of a negative feed-back cycle.
Sodium output also occurs through small amounts lost in the sweat and in the feces. In times of excessive sweating or diarrhea, depletion of sodium from these routes can be life threatening.
Sodium ingestion is influenced by taste as well as by a homeostatic drive (salt appetite) to maintain sodium balance. Humans and other animals have a drive to ingest salt that is triggered by low plasma sodium. Humans and other animals also show a distinct preference for salt ingestion, a circumstance that for a minority of humans may contribute to salt-sensitive hypertension.
Potassium
Potassium, the major intracellular ion in the body, plays a vital role in determining cell membrane potential. Although extracellular potassium concentration is low, potassium level in the extracellular fluid is carefully regulated, because changes in extracellular concentration can result in life-threatening disturbances in neural and cardiovascular function. Potassium can shift between the intracellular and extracellular compartments, depending on various neural and hormonal influences and the pH of the extracellular fluid. For example, beta-adrenergic nervous stimulation and insulin secretion both increase the movement of potassium intracellularly by stimulation of the Na+/K+ pump. In contrast, decreasing the pH of the plasma may increase the movement of potassium out of the cell.
The source of potassium in the body is that ingested in the diet. Excretion of potassium is primarily through the urine, with a small amount lost in the
sweat and the feces. The major controlling factor over total body stores of potassium is the hormone aldosterone.
sweat and the feces. The major controlling factor over total body stores of potassium is the hormone aldosterone.
As described in Chapter 18, the maintenance of potassium balance is achieved by the kidney. Potassium is freely filtered across the glomerulus and then at least 80% is reabsorbed. If excess potassium has been ingested in the diet, the kidney can also secrete potassium into the urine to return to balance. If potassium is deficient in the diet, none is secreted into the urine and all is reabsorbed. Increased secretion (and so excretion) occurs in response to stimulation of the distal tubules of the kidney by the hormone aldosterone. Aldosterone is released from the adrenal cortex in response to angiotensin II, as described previously. Aldosterone release is also, to a lesser extent, stimulated directly by low plasma potassium and by an increase in the pituitary hormone adrenocorticotropin (ACTH).
Calcium
Calcium is primarily an intracellular ion, with nearly 99% of its total body stores in bone and most of the remaining 1% stored intracellularly in other tissues. A very small amount of extracellular calcium either circulates bound to albumin; is complexed to nonorganic substances such as citrate, phosphate, or sulfate; or exists in an ionized form. Calcium in the ionized form is important for muscle contraction and a variety of enzymatic reactions. It is also required for most steps of the coagulation pathway. Calcium is ingested in the diet, filtered, reabsorbed, and excreted by the kidney. It is not secreted.
The control of serum calcium results primarily from changes in the secretion of parathyroid hormone from the parathyroid gland (see Chapter 9); decreased serum calcium stimulates increased parathyroid hormone secretion. Increased parathyroid hormone then acts in one of three ways to return serum calcium back toward normal: it (1) increases renal reabsorption of calcium; (2) stimulates bone breakdown to release bone calcium; or (3) stimulates the activation of vitamin D, thereby increasing calcium reabsorption across the gut. A second hormone, calcitonin, secreted from specialized cells of the thyroid gland, also exerts control over serum calcium levels. Calcitonin is released in response to increased serum calcium, and it acts to reduce serum calcium by causing a decrease in bone breakdown. Calcitonin also decreases calcium reabsorption by the kidney, further lowering serum levels. Serum calcium is inversely related to serum phosphate.
Phosphate
Phosphate is an intracellular ion that is vital for most metabolic reactions and is an essential component of ATP, DNA, and RNA. Phosphate also serves as a hydrogen ion buffer in plasma and urine. Approximately 85% of phosphate is stored in bone, with 14% in all other cells; less than 1% is extracellular. Phosphate is ingested in the diet and, in proportion to its ingestion, is filtered and excreted
in the urine. In the serum, it varies inversely with calcium. With renal failure, phosphate levels rise precipitously and result in low serum calcium levels.
in the urine. In the serum, it varies inversely with calcium. With renal failure, phosphate levels rise precipitously and result in low serum calcium levels.
Magnesium
Magnesium is primarily an intracellular ion that is stored in bone (50%), in body cells (49%), and in blood (1%). Magnesium is required for a variety of enzymatic reactions and is an important ion for DNA synthesis and RNA transcription, for translation, and for protein synthesis. Magnesium can bind to calcium receptors, either turning on the calcium response (mimicking) or blocking the effects of calcium. Magnesium is ingested in the diet and is filtered and excreted in the urine.
ACID-BASE BALANCE
pH
pH is a reflection of the ratio of acid to base in extracellular fluid. The pH in serum can be measured with a pH meter, or one can calculate it by measuring serum bicarbonate and carbon dioxide concentrations and placing these values into the Henderson-Hasselbalch equation, as shown in the following equation:

In this equation, HCO3− is the concentration of bicarbonate in the serum, and CO2 is the concentration of dissolved carbon dioxide in the serum. The pK refers to the negative logarithm of the dissociation constant, K. The dissociation constant is a fixed value for the bicarbonate/carbon dioxide system at normal body temperature. It reflects the degree to which bicarbonate and carbon dioxide dissociate to accept or donate a hydrogen ion. For the bicarbonate/carbon dioxide system, pK is 6.1.
The pH reflects the hydrogen ion concentration of the solution. The greater the hydrogen ion concentration, the greater the acidity of the solution and the lower the pH. In contrast, the higher the pH, the lower the hydrogen ion concentration, and the more basic the solution.
Acids
An acid is any substance capable of liberating a hydrogen ion. Examples of acids include the substances in bold in the following formulas, all of which are shown giving up a hydrogen ion:
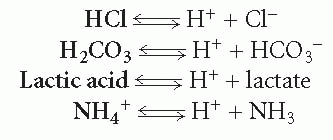
An acid can be strong or weak, depending on the degree to which it breaks down to liberate hydrogen ions. For example, hydrogen chloride (HCl) rapidly and totally breaks down into hydrogen and chloride ion; therefore, it is considered a strong acid. In contrast, few lactic acid molecules break down to hydrogen ion and lactate; therefore, lactic acid is considered a weak acid. The double arrow in each equation indicates that the reactions are reversible.
Bases
In each reaction above showing the dissociation (breakdown) of an acid, the substance produced in addition to the hydrogen ion is considered a base. A base is any substance that can accept a hydrogen ion, thereby taking it out of solution. Because each of these reactions is reversible, each substance produced with the hydrogen ion can rejoin with it and move the reaction in the opposite direction. Thus, these substances can be considered bases. These reactions are rewritten in the following formulas with the base in bold:
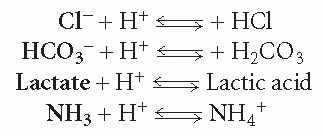
A base can be strong or weak, depending on the degree to which it accepts a hydrogen ion. Most acids and bases found in the body are weak.
Buffers
Weak acids and weak bases make good buffers. A buffer is a substance that can either take free hydrogen ions from a solution or release hydrogen ions to a solution, thereby preventing large fluctuations in pH. There are three important buffer systems in the body: the bicarbonate-carbonic acid buffer system, the phosphate buffer system, and the hemoglobin buffer system.
Bicarbonate-Carbonic Acid Buffer System
The main buffer system in the body is the bicarbonate-carbonic acid buffer system. This system acts in the blood to buffer plasma pH. When free hydrogen ions are added to blood containing bicarbonate, the bicarbonate ions bind with the hydrogen ions, becoming carbonic acid (H2CO3). This binding ensures that there will be few free hydrogen ions remaining in solution, thereby preventing a significant decrease in blood pH. Carbonic acid is considered a weak acid; bicarbonate ion is considered its weak, conjugate (complementary) base. As shown in Equation 19-2, carbonic acid can also dissociate to form carbon dioxide and water; therefore, the bicarbonate buffer system is primarily used to eliminate hydrogen ion from the body through the elimination of the volatile gas carbon dioxide. The breakdown of carbonic acid to carbon dioxide and water requires the enzyme carbonic anhydrase, which is present in red
blood cells. The reaction of carbonic acid to carbon dioxide and water is reversible, and carbon dioxide and water can rejoin to form carbonic acid. This process also requires the action of carbonic anhydrase. This reversible reaction is shown in the following equation.
blood cells. The reaction of carbonic acid to carbon dioxide and water is reversible, and carbon dioxide and water can rejoin to form carbonic acid. This process also requires the action of carbonic anhydrase. This reversible reaction is shown in the following equation.

Phosphate Buffer System
The second buffer system used by the body is the phosphate buffer system. Phosphoric acid (H2PO4−) is a weak acid; it dissociates in plasma to phosphate (HPO42−) and hydrogen ion (H+). Phosphate is a weak base. The kidney uses this system to buffer the urine as it excretes hydrogen ion. A sulfuric acid-sulfate buffer system is used to a lesser degree.
Hemoglobin Buffer System
The third main buffer system in the body, the hemoglobin buffer system, is provided by proteins in the blood, especially hemoglobin present in red blood cells. Hemoglobin binds to free hydrogen ions as the red blood cells circulate past metabolically active cells. As a result of the binding of free hydrogen ions by hemoglobin, increases in free hydrogen ion concentration in the blood are minimized, and venous blood pH decreases only slightly compared with arterial blood. As the blood flows through the lungs, hydrogen ions dissociate from the hemoglobin and join with bicarbonate to become carbonic acid (Equation 19-2), which breaks down to carbon dioxide and water. Carbon dioxide is exhaled, resulting in the elimination of the metabolically produced hydrogen ions. Most of the water is reabsorbed, and some is exhaled in the breath.
Respiratory Control of Acid-Base Balance
The lungs rid the body of carbon dioxide. Although carbon dioxide itself is not an acid, it becomes one when it joins with water to form carbonic acid (Equation 19-2). The minute-by-minute regulation of plasma pH is controlled by an increase or decrease in the rate of respiration, thereby increasing or decreasing the exhalation of carbon dioxide. This system is possible because of the sensitivity of the respiratory center in the brain to free hydrogen ions (see Chapter 14), which usually vary in accordance with carbon dioxide.
Carbon Dioxide Production and Carriage in the Blood
Carbon dioxide is produced in all cells as a result of oxidative metabolism. From the cells, it diffuses into the bloodstream. Carbon dioxide is carried in the blood in three different ways: dissolved, bound in the red cell, and as bicarbonate. Approximately 7% is carried dissolved in the blood. The amount of carbon dioxide dissolved in the blood depends on the product of its partial pressure in the blood and its solubility constant (how well it dissolves). The
solubility constant of carbon dioxide in blood is 0.57. Although the partial pressure of carbon dioxide in the atmosphere at sea level is almost zero and thus is nearly zero in inspired air, venous blood carries carbon dioxide away from metabolizing cells and has a carbon dioxide partial pressure of 45 mm Hg. Partial pressure of carbon dioxide in arterial blood is nearly 40 mm Hg. Therefore, the amount of carbon dioxide dissolved in the blood differs between the arterial and venous circulations.
solubility constant of carbon dioxide in blood is 0.57. Although the partial pressure of carbon dioxide in the atmosphere at sea level is almost zero and thus is nearly zero in inspired air, venous blood carries carbon dioxide away from metabolizing cells and has a carbon dioxide partial pressure of 45 mm Hg. Partial pressure of carbon dioxide in arterial blood is nearly 40 mm Hg. Therefore, the amount of carbon dioxide dissolved in the blood differs between the arterial and venous circulations.
As shown in Equation 19-3, 23% of carbon dioxide diffuses into red blood cells and is carried bound to hemoglobin.

After reacting with water, as shown in Equation 19-2, 70% of carbon dioxide is carried in the blood. This reaction primarily occurs in red blood cells, where carbonic anhydrase is plentiful. As a result of this reaction, a very large amount of carbon dioxide first forms carbonic acid and then bicarbonate. The reaction is driven to bicarbonate, because as each hydrogen ion is produced, it is rapidly buffered in the cell by hemoglobin. Therefore, free hydrogen concentration remains low, and the reaction continues to move to the right, until nearly all of the carbon dioxide has reacted to bicarbonate. Hemoglobin buffering of hydrogen ion ensures that there is no significant fall in pH. Carbonic anhydrase is present in the gut and kidney as well as in the red cells.
Elimination of Carbon Dioxide by the Lungs
As dissolved carbon dioxide diffuses into the lungs and is exhaled, the reactions shown in Equation 19-2 reverse and flow to the left. When dissolved carbon dioxide diffuses out of the blood and into the lungs to be exhaled, carbon dioxide bound to hemoglobin becomes dissociated, dissolves in the blood, and is exhaled. Likewise, as carbon dioxide is blown off, bicarbonate ions react with hydrogen ions to form carbonic acid, which dissolves to carbon dioxide and water, and again, the carbon dioxide is exhaled.
Mass Action of Carbon Dioxide and Hydrogen
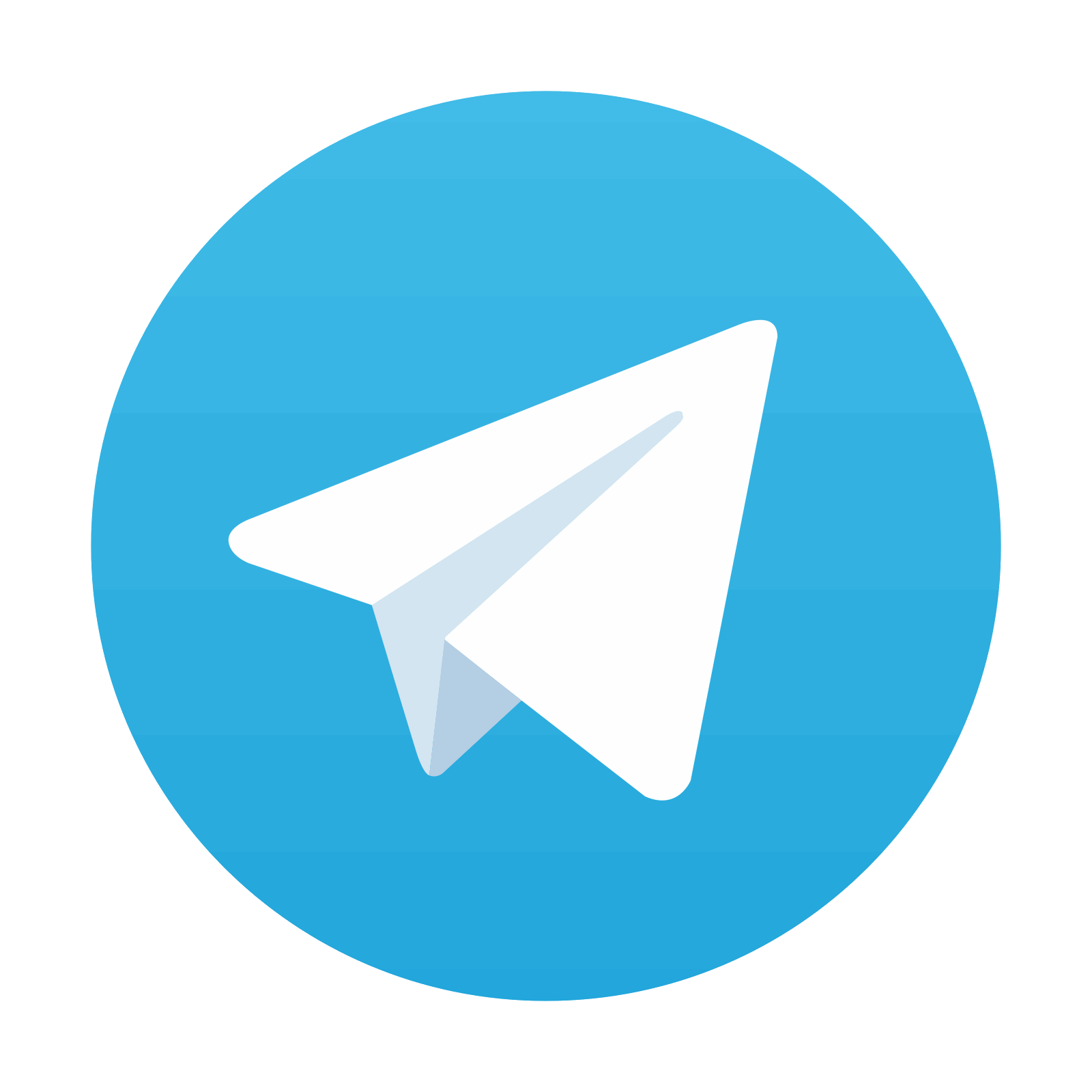
Stay updated, free articles. Join our Telegram channel

Full access? Get Clinical Tree
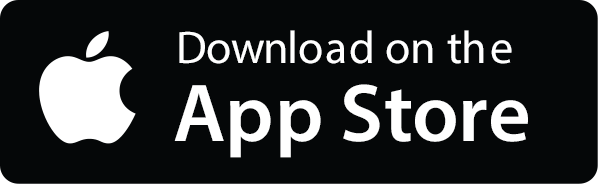
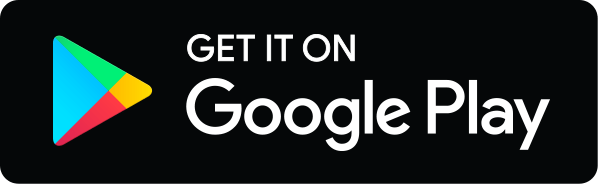
