23 FERTILIZATION, PLACENTATION, AND LACTATION
FERTILIZATION
The condensed nucleus consists of DNA surrounded by very basic protamines. Nucleosomes are not present because somatic histones have been replaced by protamines that protect and stabilize the DNA during fertilization.
The acrosomal sac is formed by three constituents (Figure 23-1): (1) the outer acrosomal membrane, (2) the inner acrosomal membrane, and (3) hydrolytic enzymes (mainly hyaluronidase and acrosin, the latter derived from the precursor proacrosin). The thin portion of the acrosomal sac, extending toward the tail, is the equatorial segment.
The three main events during fertilization are, sequentially the acrosome reaction, sperm binding to ZP3, a glycoprotein of the zona pellucida (ZP), and sperm-egg fusion (Figure 23-2).
In the proximity of the ovum, and in the presence of Ca2+, the sperm plasma membrane fuses with the outer acrosomal membrane. This event is known as the acrosome reaction. Small openings created by membrane fusion facilitate the release of hydrolytic enzymes (see Figures 23-1 and 23-2). The equatorial region of the acrosome does not participate in the plasma membrane-outer acrosome membrane fusion process. Male infertility may occur when the acrosome reaction fails to occur or takes place before the sperm reaches the egg.
Two membrane proteins have been shown to be essential for sperm-egg fusion, Izumo in the sperm and CD9 in the egg. Izumo is a sperm-specific membrane protein of the immunoglobulin superfamily that localizes to the sperm plasma membrane after the acrosomal reaction. CD9 is a member of the tetraspanin super family of transmembrane proteins (see Box 23-A). Izumo and CD9 may be involved in the organization or stabilization of plasma membrane protein complexes essential for the sperm-egg fusion reaction. Other proteins, such as ADAMs (a disintegrin and metalloprotease), may participate in this reaction. We discussed in Chapter 1, Epithelium, how the disintegrin domain of ADAMs participates in the shedding of the ectoplasmic portion of transmembrane proteins.
Box 23-A Tetraspanins
Zona pellucida during fertilization
The plasma membrane of mammalian eggs is surrounded by a 6- to 7-μm-thick zona pellucida (plural zonae pellucidae), a glycoprotein coat produced mainly by the primary oocyte during folliculogenesis, as early as during the primary follicle stage. The zona pellucida has important roles in fertilization and implantation of the embryo in the endometrium. In vitro fertilization overcomes most forms of infertility (see Box 23-B)
Box 23-B In vitro fertilization
The zona pellucida is composed of three glycoproteins (see Figure 23-2): ZP1, a dimer of 200 kd; ZP2, 120 kd; and ZP3, 83 kd. ZP2 and ZP3 interact to form a long filament complex interconnected by ZP1 dimers at regular intervals.
PLACENTATION
Cell diversity is achieved in the blastocyst, when the trophectoderm and inner cell mass are recognized. In the late blastocyst, the trophectoderm is referred to trophoblast and is distributed in two regions: in direct contact with the inner cell mass, the polar trophoblast, and surrounding the blastocyst cavity, the mural trophoblast. The blastocyst hatches from its zona pellucida at 6 to 7 days and the the differentiation of the inner cell mas proceeds.
Implantation of the blastocyst
The implantation of the blastocyst into a nurturing endometrium involves (1) the initial unstable adhesion of the blastocyst to the endometrial surface, called apposition, followed by a stable adhesion phase and (2) the decidualization of the endometrial stroma (Figure 23-3).
The timing of preimplantation and implantation is extremely precise (see Box 23-C). So is the preparation of the implantation site.
Box 23-C Timetable of implantation
On day 4 of pregnancy, the embryo—at the blastocyst stage—is within the uterine cavity. The coordinated effect of ovarian estrogens and progesterone has already conditioned the endometrium for implantation, including an increase in endometrial vascular permeability at the implantation site.
At implantation (see Figure 23-3), cytoplasmic processes of trophoblastic cells interact with small processes on the apical surface of the endometrial epithelial cells, called pinopodes, and penetrate the intercellular spaces of the endometrial cells. Penetration is facilitated by a decrease in the number of desmosomes linking the endometrial epithelial cells that undergo apoptosis.
As you recall, the endometrial lamina propria has undergone a decidual transformation during the secretory phase of the menstrual cycle. This primary decidual zone is remodeled by the action of metalloproteinases (see Figure 23-5), and a secondary decidual zone houses the implanting embryo.
Role of the decidual cells during implantation
The decidua provides an immune-protective environment for the development of the embryo. The decidual reaction involves (1) the production of immunosuppressive substances (mainly prostaglandins) by decidual cells to inhibit the activation of natural killer cells at the implantation site and (2) infiltrating leukocytes in the endometrial stroma that secrete interleukin-2 to prevent maternal tissue rejection of the implanting embryo. Syncytiotrophoblastic cells do not express major histocompatibility complex class II. Therefore, the syncytiotrophoblast cannot present antigens to maternal CD4+ T cells.
Formation of primary, secondary, and tertiary villi
At the end of the second week, cytotrophoblastic cells proliferate under the influence of the extraembryonic mesoderm, and extend into the syncytiotrophoblast mass, forming the primary villi (Figure 23-4).
Early in the third week, the extraembryonic mesoderm extends into the primary villi, forming the secondary villi (see Figure 23-4). Secondary villi cover the entire surface of the chorionic sac. In cross section, a secondary villus is formed by a core of extraembryonic mesoderm surrounded by a middle cytotrophoblast layer and an outer layer of syncytiotrophoblast.
Soon after, cells of the extraembryonic mesoderm differentiate into capillary and blood cells, forming the tertiary villi (Figure 23-5). The difference between the secondary and tertiary villi is the presence of capillaries in the latter. The capillaries in the tertiary villi interconnect to form arteriocapillary networks leading to the embryonic heart.
The following events occur as the chorionic tertiary villi continue to develop:
Histologic features of the placenta
Each lobe contains 10 or more stem villi and its branches. The 50- to 60-cmlong and 12-mm-thick and twisted umbilical cord is attached to the chorionic plate and contains two umbilical arteries (transporting deoxygenated blood) and one umbilical vein (transporting oxygen-rich blood). The umbilical vessels (Figure 23-6) are embedded in embryonic connective tissue, called Wharton’s jelly (see Chapter 4, Connective Tissue). The cord is lined by amniotic epithelium.
Maternal and fetal components
The placenta consists of a maternal and a fetal component (Figure 23-7). The maternal component is represented by the decidua. The decidua (Latin deciduus, falling off; a tissue shed at birth) is the endometrium of the gravid uterus.
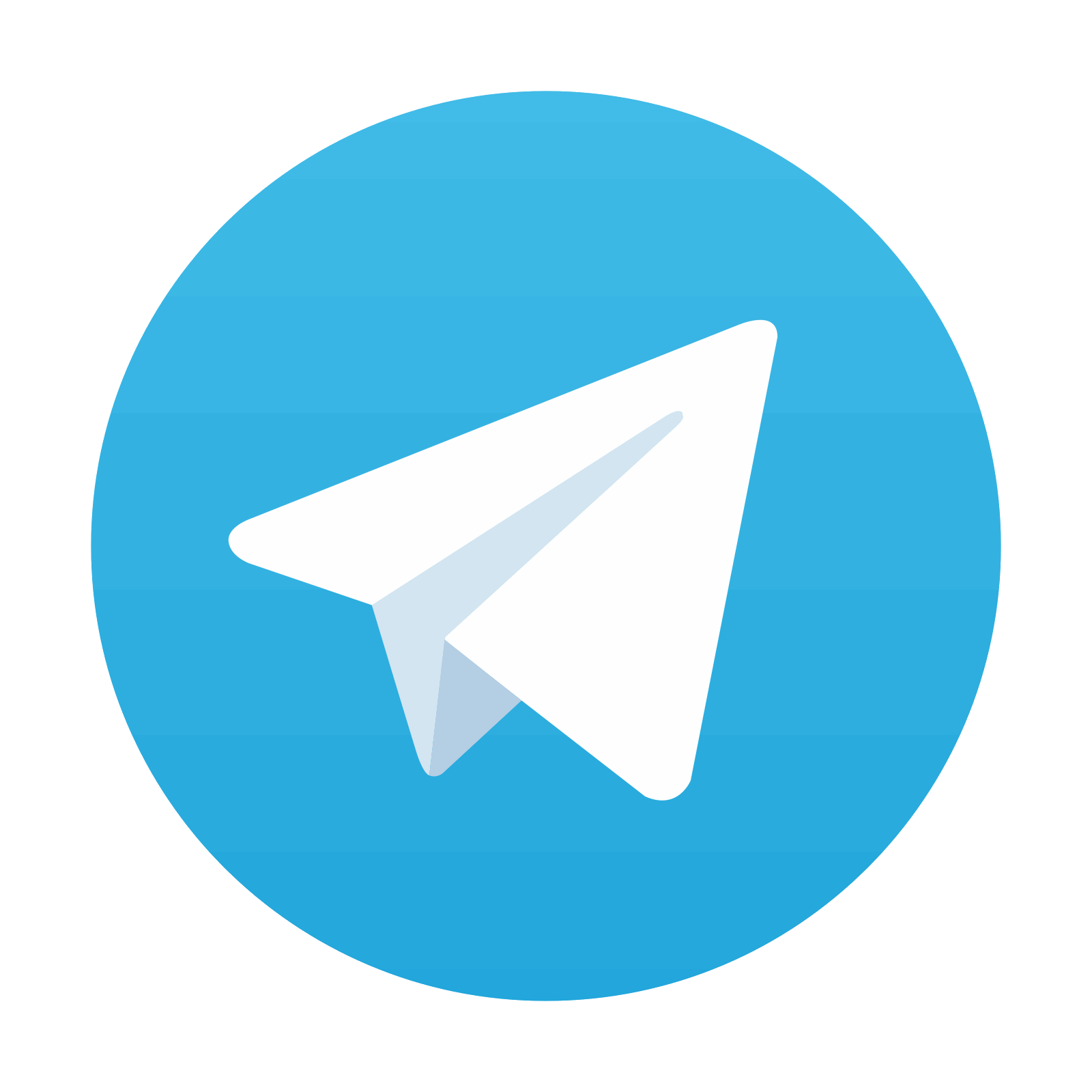
Stay updated, free articles. Join our Telegram channel

Full access? Get Clinical Tree
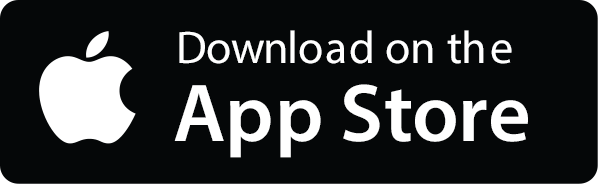
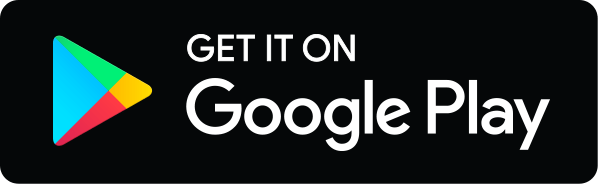