Fig. 7.1
Cytokine expression in the female reproductive tract is regulated by ovarian steroid hormones and a variety of intrinsic and extrinsic factors. The cytokine environment in the periconception period is a balance between embryotrophic (anti-apoptotic) and embryotoxic (apoptotic) factors. Together these factors influence gene expression, metabolism, cell stress and apoptosis pathways in the pre-implantation embryo to initiate downstream events impacting implantation, placental development and fetal growth, and ultimately the phenotype and health of progeny
7.3.1 Cytokine Production by Endometrial and Oviductal Epithelial Cells
A prominent maternal tract cytokine source is the epithelial cells forming the luminal surface of the oviduct and endometrium, and the endometrial glands. These epithelial cells secrete an extensive repertoire of cytokines into the luminal cavity where the embryo develops (Hannan et al. 2011). In the 1990s, colony stimulating factor-1 (CSF1 or MCSF), GM-CSF, leukaemia inhibitory factor (LIF), and transforming growth factor-β (TGFβ) were all identified as epithelial products. Later, IL11, IL13, IL15 and IL18 were added to the repertoire (Robertson et al. 1994, 1996; Tabibzadeh 1994; Pollard 1998; Salamonsen et al. 2000; Dimitriadis et al. 2005; Kelly et al. 2001; Hunt 1993; Daiter and Pollard 1992; Kimber 2005). Epithelial cells also produce an array of chemokines and vascular endothelial growth factors (VEGFs), which as well as regulating the local leukocytes and vasculature, may also influence developing embryos (Hannan and Salamonsen 2007).
Epithelial cytokine synthesis is not constitutive, but regulated at the transcriptional level over the reproductive cycle, by ovarian sex steroids. Individual cytokine genes show different temporal patterns, suggesting independent regulation or sequential activation, by mechanisms likely to involve interactions between cytokine transcription factors and steroid hormone receptors. Factors other than hormones—cytokines, growth factors and prostaglandins derived from leukocytes, seminal fluid and the conceptus—can further modulate epithelial cell cytokine expression. This is well described in mice, where a surge in GM-CSF, IL6 and an array of chemokines is induced in estrogen-primed uterine and oviductal epithelial cells after the introduction of seminal fluid at mating (Bromfield et al. 2014; Robertson 2005). The secretion pattern alters again at implantation, when increasing progesterone is associated with resolution of the inflammatory response of the peri-conception period (Robertson 1996), and a switch to anti-inflammatory CSF1 and LIF expression occurs (Daiter and Pollard 1992; Kimber 2005).
The considerable similarity between cytokine patterns suggests a high degree of conservation of pathways between mammalian species. In women, estrogen-regulated cytokines including CSF1, GM-CSF and TNF increase over the course of the proliferative phase and into the early luteal phase. A late secretory phase decline in GM-CSF and further increase in CSF1, TNF and LIF, match comparable patterns in the mouse (Hunt 1993; Daiter and Pollard 1992; Charnock-Jones et al. 1994; Giacomini et al. 1995). Seminal factors including TGFβ and PGE2 induce expression of IL8, GM-CSF, IL6 and various chemokines in human cervical epithelial cells (Sharkey et al. 2012) and may influence endometrial cytokine production as well (Gutsche et al. 2003). In the event of embryo implantation, CSF1, LIF and other progesterone-induced epithelial cytokines are upregulated and then predominate for the duration of the pregnancy (Daiter and Pollard 1992; Kimber 2005). In a non-fertile cycle, a switch back to pro-inflammatory cytokines occurs in response to pre-menstrual progesterone withdrawal (Zhang et al. 2000; Jones et al. 2004).
7.3.2 Cytokine Production by Endometrial and Oviductal Leukocytes
Large numbers of leukocytes reside in the mucosal surface of the endometrium and oviduct over the course of the cycle and in early pregnancy. These macrophages, dendritic cells, granulocytes, lymphocytes and mast cells are all potent sources of a variety of cytokines that may well access the luminal cavity and developing embryo. Their secretory profile is highly variable depending on activation state. Uterine macrophages secrete an array of cytokines in patterns that fluctuate in response to ovarian hormone changes, as well as signals from the conceptus and semen, and microbial populations (Hunt and Robertson 1996). In healthy pregnancies, decidual macrophages synthesise anti-inflammatory and tolerance-inducing cytokines TGFβ and IL10 (Chen et al. 1993; Heikkinen et al. 2003) and IL1 receptor antagonist (Tabibzadeh and Sun 1992), reflecting their anti-inflammatory “M2” phenotype. HLA-G expressed by the embryo may contribute to sustaining this behavioural state, resulting in increased TGFβ synthesis (Petroff et al. 2002).
Lymphocytes are important contributors to the uterine cytokine environment because their cytokine products, particularly IFNγ, are extremely potent at low picomolar concentrations. Many of the lymphocytes are uterine natural killer (uNK) cells, distributed throughout the decidual tissue every cycle and during pregnancy . uNK cells produce many cytokines that are relevant to embryo development including GM-CSF, CSF1, TNF, IL10, IFNγ and LIF (Croy et al. 2006). Although sparse in the endometrium, T-lymphocytes also exhibit complex patterns of cytokine production, secreting a broad array of cytokines (Piccinni and Romagnani 1996; Jokhi et al. 1994). A pivotal T-lymphocyte population required to confer immune tolerance at embryo implantation is the CD4 + CD25 + T regulatory (Treg) cells, which synthesise copious amounts of TGFβ and IL10 (Guerin et al. 2009). Eosinophils, neutrophils and mast cells also synthesize a variety of different cytokines and chemokines, including IFNγ, GM-CSF and TNF, depending on local stimuli (Yeaman et al. 1998; Cocchiara et al. 1995.
7.4 Cytokine Regulation of Embryo Development
A wide range of growth factors and cytokines exert effects in preimplantation embryos. The identity and biological actions of these factors have been reviewed extensively (Hardy and Spanos 2002; Richter 2008; Pampfer et al. 1991; Kaye and Harvey 1995; Stewart and Cullinan 1997; Kane et al. 1997; Diaz-Cueto and Gerton 2001). Experimental strategies to explore cytokine effects include supplementing embryo culture medium, neutralizing cytokine ligands or receptors with antibodies or RNA inhibitors, or null mutations in ligand or receptor genes. Together such studies show that many factors synthesized by local epithelial cells and leukocytes facilitate blastocyst development. While GM-CSF, CSF1, LIF, IL6, HB-EGF, IGFI and IGFII all have beneficial or trophic activity, others including TNF, IFNγ and TRAIL exert potent inhibitory effects, with TNF and TRAIL inducing cell death in embryos (Pampfer et al. 1994a; Riley et al. 2004) while IFNγ is embryotoxic and limits trophectoderm proliferation (Hill et al. 1987).
7.4.1 Endogenous Embryo-derived Growth and Survival Factors
For many years it was thought that unlike other cells, the preimplantation embryo develops by an entirely autopoietic process, independently of growth factor or cytokine actions. However observations that embryo culture in vitro leads to reduced developmental potential, which may partly be alleviated by culture in groups, or when culture medium volume is minimised, led to the discovery of a wide range of endogenously-produced trophic factors (Paria and Dey 1990). Comprehensive analysis of preimplantation embryos from several species show synthesis of several growth factors and cytokines , including IL1, LIF, growth hormone (GH), insulin-like growth factor-I (IGFI), IGFII, EGF, HB-EGF, TGFα, TGFβ, vascular endothelial growth factor (VEGF), platelet-derived growth factor (PDGF) and IL6 (Fig. 7.2). The co-expression of cognate receptors from fertilization onwards, indicates the capacity for autocrine and juxtacrine interactions between adjacent blastomeres.
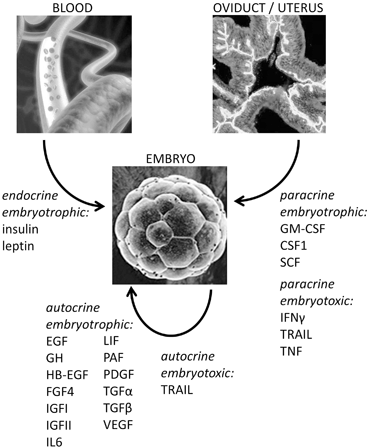
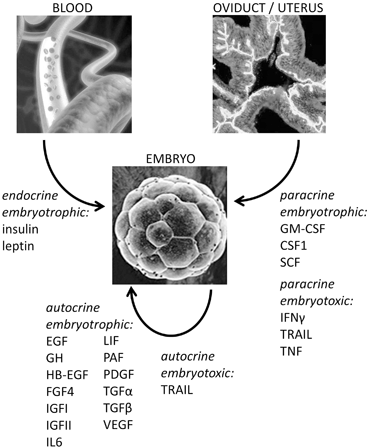
Fig. 7.2
A wide range of cytokines impact development of the preimplantation embryo as it traverses the female reproductive tract prior to implantation. These cytokines can be classified according to their embryotrophic (anti-apoptotic) or embryotoxic (apoptotic) effects, and also by their autocrine, paracrine or endocrine source. Almost all of the cytokines synthesised endogenously within the embryo are also secreted by female tract tissues and so there is the opportunity for both autocrine and paracrine activity. However some cytokines, notably embryotrophic factors GM-CSF and CSF1 are not synthesised by the embryo and are solely of maternal origin. Embryotoxic factors IFNγ, TRAIL and TNF, are regulated by environmental events and can also exert potent death-inducing effects on embryos
Defining the actions of any single endogenous factor is challenging, given that there are so many factors with shared receptors and overlapping actions. Experimental strategies to define the requirement and actions of endogenous factors require neutralising antibodies or small inhibitory RNAs to block factor production, receptor function or downstream signalling cascades. However, exogenous addition of various factors has been shown to affect embryo viability, gene expression and development. Trophic ligands are important for sustaining viability and developmental competence from the early cleavage stage of development (O’Neill 1997).
To date there is no evidence that autocrine factors induce blastomeres to enter the mitotic cell cycle; rather they act as survival agents to prevent cells from constitutive death pathways mediated by p53-induced apoptosis (O’Neill et al. 2012). Observations that growth factors increase the cell number in blastocysts has sometimes been interpreted as indirect evidence of a mitogenic effect (Byrne et al. 2002a, b). However given that growth factors exert anti-apoptotic effects more often and at lower concentrations than required to stimulate cell proliferation, this effect is more likely to be explained by effects of growth factors on protecting cells from apoptosis.
Exposure to cytokines acts to reduce the proportion of blastomeres that would otherwise undergo apoptosis due to cell stress associated with survival factor deprivation, but does not completely prevent apoptosis in developing embryos (Sjoblom et al. 2002). A certain level of apoptosis in blastocysts is considered normal in both in vivo and in vitro developed embryos, since apoptosis is a necessary biological mechanism for elimination of abnormal or unwanted cells in development (Hardy 1999). To date, there is no evidence that provision of physiologically appropriate levels of cytokines to embryos in vitro causes rescue of abnormal cells, and such an action would seem unlikely given that no such function of cytokines is seen in other cell lineages.
In addition to effects within the embryo, several embryo-derived factors communicate with maternal tissues in vivo. Embryo-derived IL1, hCG and HLA-G are each implicated in signalling to elicit endometrial receptivity prior to implantation. Sheep and cattle preimplantation embryos release IFNτ to mediate maternal recognition of pregnancy through preventing regression of the corpus luteum (Bazer et al. 1997).
7.4.2 Maternal Tract Cytokine—Embryo Communication
It is generally accepted that even with best practice and conditions to promote autocrine growth factor actions, embryos develop at a slower rate and with fewer cells in vitro than in vivo (Richter 2008). This demonstrates the crucial contribution made by the female reproductive tract in supplying an optimal environment, underscoring a role for paracrine factors secreted by maternal tissues. In vivo, cytokines released from oviductal and uterine cells bathe the developing embryo as it traverses the reproductive tract prior to implantation. Their concentrations are sufficient to exert biological functions and the high permeability of the zona pellucida allow their free access to the cell surface of the blastomeres within the embryo.
Through promoting or inhibiting a wide range of cellular events that determine blastomere survival and the timing of division and differentiation, maternal factors are capable of acting in concert with autocrine mediators produced within the embryo to modulate the endogenous developmental program (Table 7.1). Almost all of the same cytokines and growth factors known to be produced by the embryo are also released into the luminal fluid by oviductal epithelial cells. However some factors are not made by embryos, and are known to have beneficial effects on embryo development. The most notable of these are GM-CSF and CSF1 (Fig. 7.2). Given this substantial overlap, a crucial question is whether the maternal factors have unique roles or simply reinforce the actions of endogenous autocrine factors. Since production of growth factors incurs a metabolic cost it seems self-evident that there must be biological and evolutionary benefits.
Table 7.1
Cytokines exert a wide range of physiological effects in mouse and human preimplantation embryos
Physiological effecta | Mouse | Human |
---|---|---|
% zygote development to blastocyst | Yes | Yes |
Kinetics of development to blastocyst | Yes | Yes |
Cell number & allocation to ICM and TE | Yes | Yes |
Cell viability and apoptosis | Yes | Yes |
Gene expression profile | Yes | Yes |
Metabolism | Yes | Yes |
Stress response | Yes | Yes |
Implantation & developmental competence | Yes | Yes |
Developmental programming in fetus | Yes | ? |
From a physiological perspective, it is reasonable to suppose that the flexibility provided by maternal factor signalling could contribute to (1) altering cell survival and function to influence embryo viability and implantation success; (2) synchronising embryo growth with maternal receptivity; (3) mediating embryo sensing and adaptation to varying environmental circumstances, and/or (4) maternal quality control of reproductive investment. Carefully designed experiments to distinguish the actions of autocrine from maternal cytokine actions, as well as effects in the maternal tract from those in the embryo, provide evidence to support each of these pathways, as discussed below.
7.5 Cytokine Receptor Signal Transduction in Pre-Implantation Embryos
A crucial factor in defining the roles and relative importance of different maternal cytokines is their potential redundancy in signalling activity. Such information would help clarify whether a specific blend of factors is required for optimal embryo health, or whether one or two crucial cytokines might be sufficient. To answer this issue it is necessary to consider the range of receptors and signalling cascades active in embryos. Such evidence reveals extensive signalling redundancy with many receptors converging on the same intracellular signalling pathways. Different cytokines act through one or more downstream pathways, of which the most prominent in embryos are the phosphatidylinositol-3 kinase-protein kinase B (PI3K-AKT), Janus kinase/signal transducer and activator of transcription (JAK/STAT), and mitogen-activated protein kinase (MAPK) pathways. For some cytokine receptors, ligation activates only PI3K-AKT, while others activate all three signalling pathways (Table 7.2).
Table 7.2
The cytokine signalling pathways used by key ligands and receptors implicated as regulators of cell survival or cell death in pre-implantation embryos
Ligand | Receptor | Embryo | Maternal | Signalling pathway |
---|---|---|---|---|
Embryotrophic factors | ||||
GM-CSF | GMRα | – | ✓ | JAK/STAT5, PI3K-AKT, MAPK |
SCF | c-kit | – | ✓ | ERK, JAK/STAT1, PI3K-AKT |
CSF1 (M-CSF) | c-fms | – | ✓ | ERK, JAK/STAT1, PI3K-AKT |
Insulin | IRS | – | ✓a | PI3K-AKT |
Leptin | – | ✓a | ||
EGF | EGFR | ✓ | ✓ | JAK/STAT1/STAT3, PI3K-AKT, MAPK |
HB-EGF | EGFR | ✓ | ✓ | JAK/STAT1/STAT3, PI3K-AKT, MAPK |
GH | GHR | ✓ | ✓ | JAK/STAT5, PI3K-Akt, MAPK |
IGF-I | IGF-IR | ✓ | ✓ | PI3K-AKT |
IGF-II | IGF-IIR | ✓ | ✓ | PI3K-AKT |
IL6 | IL6R, gp130 | ✓ | ✓ | JAK/STAT3, PI3K-AKT, MAPK |
LIF | LIFR, gp130 | ✓ | ✓ | JAK/STAT3, PI3K-AKT, MAPK |
PAF | PAFR | ✓ | ✓ | PI3K-Akt |
TGFα | EGFR | ✓ | ✓ | JAK/STAT1/STAT3, PI3K-AKT |
TGFβ | TGFBR1, TGFBR2 | ✓ | ✓ | SMAD2/3, MAPK |
Embryotoxic factors | ||||
IFNγ | IFNGR1, IFNGR2 | – | ✓ | JAK/STAT, SMAD6/7, apoptosis |
TNF | TNFR1 | – | ✓ | MAPK, NFκB, apoptosis |
TRAIL | TRAILR (DR4, DR5) | ✓ | ✓ | Apoptosis |
7.5.1 Phosphatidylinositol-3 kinase (PI3K)/Protein Kinase B (AKT) Pathway
A canonical pathway activated by many embryotrophic cytokines is the PI3K-Akt signalling pathway. PI3K-mediated phosphorylation of the inositol ring 3’-OH group in inositol phospholipids is the rate-limiting step in forming phosphatidylinositol-3,4,5-triphosphate (PIP3) (Fruman et al. 1998), which in turn provides a docking site for a wide range of important signalling molecules that promote cell survival, prevent apoptosis and induce calcium mobilisation. Class I PI3K are heterodimers composed of a catalytic subunit (p110) and an adaptor/regulatory subunit (p85). The PI3K pathway is involved in cell growth, differentiation, proliferation, motility, survival and intracellular trafficking in many cell lineages. It is recognised as a major anti-apoptotic pathway operating in all cells, activated by a wide range of trophic signals including soluble growth factors as well as by attachment to extracellular matrix (Cantley 2002). A major component in the survival signalling induced by the activation of PI3K is the protein kinase B (AKT) family of protein serine/threonine kinases, AKT1, AKT2 and AKT3 also known as PKBα, PKBβ and PKBγ (Osaki et al. 2004).
The PI3K pathway is present in the early embryo (Li et al. 2007) and is activated by many embryotrophic ligands such as CSF1, SCF, PAF, insulin, IGF1, IGFII and TGFα (O’Neill 2008; Brison and Schultz 1997; Kawamura et al. 2005) (Table 7.2), which is consistent with observations these ligands act as autocrine survival factors in embryos in an overlapping, redundant manner. Pharmacologic inhibition of PI3K or AKT reduces embryo development in vitro, demonstrating the essential role of autocrine signalling via PI3K for embryo survival (Li et al. 2007). Transcription of the cell survival gene Bcl2 in the 2-cell embryo depends on PI3K (Jin and O’Neill 2011), while activation of PI3K/AKT is required to suppress pro-apoptotic Bax and to sustain the latent, inactive form of transformation related protein 53 (TRP53) (Jin et al. 2009), a major stress-induced transcription factor which when activated, leads to blastomere death and embryo demise.
7.5.2 Janus Kinase/Signal Transducer and Activator of Transcription (JAK/STAT) Pathway
The majority of cytokines (the interleukins, colony stimulating factors and many others) all signal through type 1 or type 2 cytokine receptors which activate the JAK signal transduction pathway. Depending on the specific cytokine-receptor interaction, one or more of four tyrosine kinases (JAK1, JAK2, JAK3 and TYK2) mediate signalling by phosphorylating tyrosine residues on various downstream signalling components, including the STAT factors (Leonard 2001). Subsequent dimerisation of phosphorylated STATs leads to translocation to the nucleus, where they bind to DNA and either initiate or inhibit transcription. There are seven members of the STAT family, each activated by a range of different cytokines (Mitsuyama et al. 2001) to regulate cell survival and proliferation. The different STATs have non-redundant biological roles—for instance, STAT1 and STAT2 mediate interferon signalling while STAT3 and STAT4 mediate IL-12 signalling (Mitsuyama et al. 2001). STAT3 and STAT5A/B transduce signals from a broad range of cytokines, as exemplified by the lethal phenotypes in mice deficient in STAT3, STAT5A or STAT5B (Leonard and O’Shea 1998).
The JAK/STAT pathway is constitutively activated in preimplantation mouse embryos with evidence of phosphorylated STAT1, STAT2 and STAT3 in the nucleus within oocytes and through the preimplantation period (Truchet et al. 2004). Several cytokines using type 1 and type 2 cytokine receptors are active in embryos, including GM-CSF, growth hormone (GH), leptin, LIF and IL6 (Kimber 2005; Markham and Kaye 2003; Robertson 2007; Shen et al. 2009; Kawamura et al. 2002) which largely operate through STAT3 (LIF, IL6, leptin) and STAT5 (GM-CSF, GH). Interestingly, ligation of type 1 and type 2 cytokine receptors generally activates PI3K and MAPK signalling as well as JAK/STAT, influencing a wider range of downstream target genes and cellular events (Table 7.2). STAT3 is reportedly essential for maintenance of pluripotent inner cell mass (ICM) lineages, but not for blastocyst formation (Do et al. 2013). STAT5A/B protein expression is present in the nucleus from the 1-cell to blastocyst stage in mouse pre-implantation embryos, and reduced embryo development is observed following treatment with JAK inhibitor, consistent with a key role for STAT5 in mediating cytokine signalling in embryos (Nakasato et al. 2006). These experiments imply an active role for the JAK/STAT pathway within embryos in autocrine and paracrine cytokine signalling.
7.5.3 Mitogen-Activated Protein Kinase (MAPK) Pathway
The MAPK pathway is utilised by several cytokines and growth factors (including TGFα, EGF, HB-EGF, TGFβ) to elicit mitogenic signal transduction and stimulation of cell proliferation and differentiation (Pearson et al. 2001). The MAPK superfamily of proteins comprises four separate signalling cascades: the c-Jun N-terminal kinase or stress-activated protein kinase (JNK/SAPK) (Woodgett et al. 1996), the extra-cellular-signal-regulated kinases (ERKs) (Boulton and Cobb 1991), the ERK5 or big MAPK1 (BMK1) (Zhou 1995) and the p38 MAPK group of protein kinases (Han et al. 1994). The entire MAPK pathway is detectable in mouse pre-implantation embryos at the mRNA, protein and phosphoprotein level (Wang et al. 2004). The p38 MAPK cascade, which is activated in response to many types of environmental stress and pro-inflammatory cytokines (Ono and Han 2000), is demonstrated to be required for development from the 8-cell to blastocyst stage and inhibition of this pathway in vitro causes complete loss of filamentous actin in 8-cell to 16-cell embryos (Natale et al. 2004; Paliga et al. 2005). SAPK/JNK mRNA transcripts are expressed in mouse pre-implantation embryos, suggesting a role in mediating stress-induced responses that can cause embryo demise (Zhong et al. 2004).
7.6 Cytokines Promoting Pre-Implantation Embryo Development
Out of the full range of cytokines produced by the maternal tract, some are strong candidates for key effectors in embryo development and will be discussed in detail. The factors of greatest interest have characteristics including: (1) their production by the female reproductive tract which thus have paracrine actions on the embryo, as opposed to autocrine factors produced by the embryo itself; (2) action through more than one signal transduction pathway in embryos leading to profound effects on stress response , apoptosis and developmental competence; (3) a capacity for regulation in response to changing environmental conditions and stressors, as well as male seminal fluid signals, and (4) experimental indications that their perturbation leads to altered embryo development and has long-term impact on the offspring. Cytokines with trophic effects on embryo development will be discussed first.
7.6.1 Granulocyte-Macrophage Colony-stimulating Factor (GM-CSF)
The cytokine GM-CSF meets all of the criteria listed above as a non-redundant, master regulator of embryo health. GM-CSF is a 23 kDa glycoprotein originally identified as a regulator of myeloid haematopoietic cells (Ruef and Coleman 1990). Its receptor in hemopoietic cells consists of a GM-CSF specific subunit (GM-Rα) which confers low-binding affinity and a high affinity beta common (βc, gp160 or CD131) subunit which does not bind GM-CSF directly, but forms a high affinity complex when associated with the ligand coupled GM-Rα (Gearing et al. 1989). During early pregnancy, GM-CSF is secreted into the luminal compartment by epithelial cells in the oviduct and the uterus . In the mouse, its synthesis is predominantly induced by oestrogen and is further up-regulated in the oviduct and the uterus following exposure to seminal fluid which reaches these distal parts of the tract after coitus (Bromfield et al. 2014; Robertson and Seamark 1992). In women, GM-CSF is secreted by Fallopian tube and uterine epithelial cells, where its expression is maximal in the mid-luteal phase (Giacomini et al. 1995; Zhao et al. 1994). Although seminal fluid elevates GM-CSF in the cervix of women (Sharkey et al. 2012), whether there is any influence on expression in the oviduct and uterus is not known, and may not occur given the limited access of seminal fluid to regions of the tract beyond the cervix in women.
GM-Rα, but not βc, is expressed at all stages of pre-implantation embryo development. In blastocysts, expression occurs in both the outer trophectoderm layer and the ICM cells (Sjoblom et al. 2002; Robertson et al. 2001). Notably, GM-CSF ligand is not detectable in mouse or human embryos at any preimplantation developmental stage (Robertson et al. 2001; Murray et al. 1990), such that all GM-CSF affecting embryos in vivo is of maternal tract origin. In vitro experiments in mouse embryos show that GM-CSF acts as an embryotrophic factor with survival-promoting effects on the ICM (Sjoblom et al. 2002; Robertson et al. 2001). Culture with GM-CSF generates blastocysts with a 20 % increase in the total number of cells, particularly in the ICM where 30 % more viable cells were seen (Sjoblom et al. 2002; Behr et al. 2005).
GM-CSF, like several growth factors produced by the embryo itself, operates as a survival factor in embryos, providing protection from the default pathway of stress response and apoptosis that occur in the absence of growth factors. The rates of cell division, differentiation and/or cell death that accompany progression from zygote to blastocyst are highly sensitive to disruption by various environmental stressors, some of which alter growth factor balance and bioavailability (Xie et al. 2008). Most notably, culture ex vivo exerts cell stress resulting in fewer blastomeres, due both to retarded progression through the cell cycle and elevated incidence of apoptosis (Xie et al. 2006). A response to stress that precedes apoptosis is activation of the heat shock protein (HSP) or stress response pathway, which acts to limit cell damage and facilitate recovery. HSPs interact with and modulate the apoptosis pathway, and influence commitment to either cell death or survival, depending on the severity of cell damage. Microarray and qPCR studies show that exposure to GM-CSF mitigates culture-induced activation of gene pathways associated with cell stress and apoptosis, suppressing several HSPs and pro-apoptosis factors including Bax (Chin et al. 2009). Pathways associated with integrity and developmental competence—including cell adhesion and tight junctions—are also affected (Chin et al. 2009). This is consistent with observations that the GM-CSF response may interact with environmental stressors, since GM-CSF appears most effective in sustaining blastocysts when stress is induced by omitting human serum albumin from the culture medium (Karagenc et al. 2005). One role of albumin is to act as a carrier for autocrine growth factors that sustain embryo development.
Confirming a role for maternal GM-CSF in vivo, blastocyst development is impaired in mice with a null mutation in the Csf2 gene encoding GM-CSF. Thus, blastocysts flushed from Csf2 null mothers have 18 % fewer cells in both the trophectoderm and ICM (Robertson et al. 2001); a phenotype associated with increased expression of heat shock protein Hsph1 (Chin et al. 2009), and a subfertility phenotype with 25 % smaller litter size at weaning (Robertson et al. 1999). Since embryos do not produce this cytokine, interpretation of these data is not confounded by effects of endogenous GM-CSF synthesis within pre-implantation embryos. The developmental alterations in blastocysts in Csf2 null mice can thus be attributed to deficiency in maternal tract-derived cytokine, despite the presumably normal production of other embryotrophic factors in embryos and by maternal tissues. This result provides clear evidence for an important role for maternal tract derived GM-CSF in mouse preimplantation embryos, and supports the conclusion that endogenous trophic factors alone are insufficient for optimal development.
In hematopoietic cells, GM-CSF signalling largely occurs via the βc subunit to result in activation of JAK2, STAT5, ERK1/2 and PI3K pathways (Guthridge et al. 1998). In embryos, signalling in response to GM-CSF has not been well investigated, but microarray experiments indicate that genes associated with the MAPK, STAT and PIP3K signalling pathways are influenced by GM-CSF (Chin et al. 2009), consistent with use of multiple signalling pathways by this ligand. Interestingly, there is emerging evidence that when the βc subunit is absent, GM-CSF signals predominantly through the PI3K and p85 pathways (Dhar-Mascareno et al. 2005; Perugini et al. 2010), to cause translocation of glucose transporters to the surface membrane via a hydrogen peroxide-dependent mechanism (Dhar-Mascareno et al. 2003). This is consistent with a key effect of exposure to GM-CSF in mouse blastocysts on glucose uptake (Robertson et al. 2001). Since a switch to anaerobic glycolysis is programmed at the blastocyst stage (Leese 2012) and the rate of blastocyst glycolysis correlates inversely with developmental potential (Gardner et al. 2011), facilitating glycolytic activity in blastocysts may be a key effect of this cytokine.
In human IVF embryos, culture is generally characterised by arrested, delayed and abnormal cell division with fewer than 50 % of embryos reaching the blastocyst stage (Hardy and Spanos 2002). Profound embryotrophic effects of GM-CSF are seen in human embryos (Sjoblom et al. 1999), with addition of GM-CSF to culture medium from the 2-cell stage increasing the proportion of embryos developing to blastocysts from 30 to 76 % (Sjoblom et al. 2002; Sjoblom et al. 1999). The developmental competence of these blastocysts, assessed by hatching and attachment to extracellular matrix-coated culture dishes, was markedly improved by GM-CSF exposure. The period in culture required to yield 50 % blastocyst development was reduced by 14 h, and blastocysts grown in GM-CSF contained approximately 35 % more cells, due primarily to a 50 % reduction in the incidence of apoptosis and an increase in the size of the inner cell mass (Sjoblom et al. 1999). Even in the presence of other cytokines, GM-CSF has a paramount role, since when human IVF embryos were co-cultured with autologous endometrial cells, production of GM-CSF correlated with likelihood of successful pregnancy after embryo transfer (Spandorfer et al. 1998).
Effects of GM-CSF on promoting blastocyst development in vitro are reported for bovine (de Moraes and Hansen 1997) and porcine (Cui et al. 2004) embryos. In sheep embryos (Imakawa et al. 1993) and cattle embryonic trophectoderm cells (Michael et al. 2006), GM-CSF promotes blastocyst secretion of the pregnancy recognition molecule IFNτ.
7.6.2 Colony Stimulating Factor-1 and Stem Cell Factor
CSF1, like GM-CSF, is not synthesised within the embryo itself is secreted by the maternal tract has a paracrine influence on the embryo. In mice and in women, uterine epithelial cells express high levels of CSF1 under the influence of ovarian steroid hormones (Kauma et al. 1991; Pollard et al. 1987). The receptor for CSF1, the c-fms protooncogene, is present in mouse oocytes but then decreases to undetectable levels after fertilisation, before reappearing with embryonic genome activation at the late two-cell stage with persistence through to the blastocyst stage (Arceci et al. 1992). Similar expression patterns are seen in human embryos (Sharkey et al. 1995). Inclusion of CSF1 in embryo culture media promotes development of blastocysts in mice, and appears to rescue development of slow-growing embryos (Pampfer et al. 1991). In CSF1 deficient op/op mice, reduced fertility may in part be linked with adverse effects in blastocysts, although this is difficult to discern against the broader reproductive defects evident in these mice (Cohen et al. 1997). Detailed analysis has shown that formation of the blastocoel cavity is accelerated in mouse embryos in the presence of CSF1, and that the resulting blastocysts have higher cell numbers due primarily to greater numbers of trophectoderm cells (Bhatnagar et al. 1995).
The receptor for stem cell factor (SCF, also known as steel factor, SF) is the c-kit protooncogene which follows a similar pattern of expression, being abundant in oocytes, diminishing in one-cell embryos and then reappearing from the late two-cell stage onwards (Arceci et al. 1992). SCF is highly expressed in oviducts and uterus through early pregnancy, demonstrating the prospect of paracrine signalling to the developing embryo (Arceci et al. 1992). Addition of SCF improves blastocyst formation, and silencing c-kit reduces the kinetics of blastocyst formation indicating a proliferation-inducing effect (Lim et al. 2010). Since both c-fms and c-kit are tyrosine kinases that activate similar signalling pathways, there is a high likelihood of cross-compensation between the two ligand-receptor systems. Paracrine signalling to human embryos via the SCF/c-kit system is likely since human embryos express c-kit (Sharkey et al. 1995), and the decidualised endometrium is a rich source of this factor (Kauma et al. 1996).
7.6.3 Heparin-Binding EGF-like Growth Factor (HB-EGF)
A key example of a factor produced both by the embryo and the maternal tract is heparin-binding EGF-like growth factor (HB-EGF), a member of the EGF family. In humans and mice, HB-EGF is expressed in the oviduct (Sun et al. 2006; Dalton et al. 1994) and the uterine luminal epithelium, where it is produced in both transmembrane and soluble forms (Raab et al. 1996), suggesting a role as a paracrine mediator. In the mouse endometrium, leukaemia inhibitory factor (LIF) is a regulator of HB-EGF release (Song et al. 2000). The HB-EGF receptor EGFR is expressed in blastocysts (Wang et al. 2000) and signals via ErbB1 and ErbB4 from the EGF tyrosine kinase receptor family (Lim and Dey 2009). HB-EGF is highlighted as an important early molecular marker for embryo-maternal uterine epithelium communication during implantation, since expression of HB-EGF in the luminal epithelium is not hormone-regulated, but instead is induced in epithelial cells by blastocyst signals (Das et al. 1994). HB-EGF is also expressed in the blastocyst during implantation, suggesting an auto-induction loop during implantation (Hamatani et al. 2004).
In vitro studies showed that soluble HB-EGF stimulates proliferation, zona-hatching and trophoblast outgrowth in mouse blastocysts (Das et al. 1994). In Hb-egf null mutant mice, delayed implantation results in compromised pregnancy outcome (Xie et al. 2007). A similar signalling pathway is implicated in human implantation (Paria et al. 2001). Addition of HB-EGF substantially improved the proportion of human embryos developing to the blastocyst stage, as well as their developmental competence as assessed by hatching, adherence to extracellular matrix proteins and trophectoderm outgrowth (Martin et al. 1998). Blastulation rates increased from 41 % in the control group to 71 % in the presence of HB-EGF, and this effect of HB-EGF is accompanied by changes in embryo gene expression (Kimber et al. 2008). In contrast to GM-CSF however, HB-EGF did not accelerate the speed of development or increase cell number and its effect was limited to good quality embryos.
7.6.4 Leukaemia Inhibitory Factor (LIF)
LIF is a 45–56 kDa secreted glycoprotein member of the IL6 family of cytokines (Auernhammer and Melmed 2000). Initially identified as a differentiation factor in myeloid cells (Hilton et al. 1988), LIF has multiple effects on a variety of cell types and tissues. It is produced by the luminal epithelium and epithelial glands in the mouse uterus and its expression is up-regulated from ovulation until implantation, then down-regulated following decidual formation (Bhatt et al. 1991). LIF is also expressed in the oviduct where it is induced by factors in male seminal fluid (Bromfield et al. 2014; Robertson et al. 1993). Unlike GM-CSF and CSF1, LIF is expressed in pre-implantation mouse embryos (Murray et al. 1990), indicating that maternal LIF acts to supplement autocrine embryo-derived LIF activity.
LIF was the first cytokine discovered to have a non-redundant function in reproduction. It is crucial for successful embryo implantation as demonstrated by implantation failure and infertility in female Lif null mutant mice (Stewart et al. 1992). The key role of maternal, as opposed to embryo-derived, LIF is indicated by observations in Lif null mice, where injecting LIF on the day of implantation rescues fertility, while LIF deficient embryos are not developmentally compromised, but implant and develop successfully in wild-type mice (Stewart et al. 1992). LIF receptor (LIFR) is expressed in preimplantation embryos, predominantly from the blastocyst stage of development (Charnock-Jones et al. 1994; Nichols et al. 1996; Sharkey et al. 1995), and LIF ligation of the LIFR/gp130 complex activates the MAPK and PI3K pathways as well as JAK/STAT3 pathways in embryonic cells (Graf et al. 2011).
Like embryos developed without GM-CSF, LIF non-responsiveness does not arrest preimplantation development . However while Lifr null embryos develop to term, they show developmental anomalies incompatible with survival (Ware et al. 1995). Embryo culture and transfer experiments (see later) suggest that at least in part, this is due to adverse programming influences of LIF deficiency at the preimplantation stage. In mouse embryos, addition of LIF to embryos in vitro acts to increase the hatching rate and outgrowth of blastocysts cultured from the 8-cell stage onwards (Robertson et al. 1991). Studies in Lif null mutant mice show that LIF acts from the 4 cell stage to phosphorylate STAT3, an important regulator of the OCT4-NANOG circuitry required to maintain embryonic stem cell self-renewal, but that this action is redundant and can be replaced by the related cytokine IL6 (Do et al. 2013). Indeed, IL6 may partially protect embryos from LIF deficiency by activating STAT3-regulated anti-apoptotic pathways during cleavage stages (Shen et al. 2009). The actions of LIF in benefiting embryo development are more profound in human than in mouse embryos. Addition of LIF to a complex, serum-free culture media increased the blastulation rate from 18 to 44 % (Dunglison et al. 1996), and caused changes in embryo gene expression (Kimber et al. 2008), although this was not reflected in any advance in attachment and trophectoderm outgrowth.
7.6.5 Insulin-Like Growth Factor I and II (IGFI and IGFII)
The insulin-like growth factor family comprises the ligands, IGFI and IGFII as well as their respective receptors, IGFI receptor (IGFIR) and IGFII receptor (IGFIIR). IGFI binds solely to the IGFIR, while IGFII binds strongly to the IGFIIR and weakly to the IGFIR. IGFI and IFG-II are expressed in the oviduct (Dalton et al. 1994; Lighten et al. 1998; Pfeifer and Chegini 1994; Carlsson et al. 1993; Shao et al. 2007; Zhang et al. 1994). IGFI added to mouse embryos in vitro can promote pre-implantation embryo development, cell number and metabolism (Brison and Schultz 1997). Both IGFIR and IGFIIR are expressed in the mouse and human pre-implantation embryo (Rappolee et al. 1992; Smith et al. 1993; Lighten et al. 1997).
IGFII is also expressed by the mouse pre-implantation embryo (Rappolee et al. 1992). Expression of IGFII and IGFIIR is parentally imprinted, with the male inherited allele expressing specifically IGFII and the female allele expressing IGFIIR, in a mechanism that involves long-range regulation through shared regulatory elements (Caspary et al. 1998). Although IGFI and IGFII are synthesised endogenously, their bioavailablity may be diminished by dilution in standard culture environments where there is a larger volume of fluid surrounding the embryo compared with in vivo, such that embryos can be shown to benefit from the provision of additional exogenous factor. IGFI added to culture medium acts to promote blastocyst development rate and increase the inner cell mass cells in mouse embryos (Harvey and Kaye 1992). Exogenous IGFII increases protein synthesis within trophectoderm cells in mice (Rappolee et al. 1992).
Genetic studies show that the absence of Igf1, Igf2 or Igf1r result in significantly reduced birth weight associated with neonatal lethality (Baker et al. 1993; Liu et al. 1993). However these studies do not specifically attribute poor fetal growth to effects of IGF signalling in pre-implantation embryos, and instead suggest that deficiency in growth factor signalling impairs later post-implantation stages of development. IGFI null mutant mice exhibit postnatal lethality, growth retardation and infertility (Liu et al. 2000). The use of a bi-transgenic mouse model shows that IGFI plays a significant role in programming growth as well as sustaining postnatal development and reproductive function (Stratikopoulos et al. 2008). In contrast, IGFII modulates fetal and placental growth (Constancia et al. 2002) and appears to influence the placental programming of anxiety in the adult offspring (Mikaelsson et al. 2013).
Culture of human pre-implantation embryos with IGFI substantially enhances development (Lighten et al. 1998), with addition of IGF1 to culture media increasing the blastulation rate from 35 to 60 %, associated with an increase in blastocyst cell number due to more cells in the inner cell mass (Lighten et al. 1998).
7.7 Cytokines Inhibiting Pre-Implantation Embryo Development
As well as cytokines which are embryotrophic, several others can have profound adverse effects on embryo development. Like the trophic factors discussed above, these cytokines are responsive to induction and regulation in the female tract, and in particular can be elevated in times of local or systemic infection or a range of other nutritional or environmental stressors. In the event of embryo exposure to high levels of these factors, the result may be embryo demise and failure to progress to pregnancy .
7.7.1 Tumour Necrosis Factor Alpha (TNF)
TNF is a pleiotropic cytokine exhibiting pro-inflammatory functions, eliciting a number of different cellular responses (Baker and Reddy 1998). Uterine macrophages and natural killer (NK) cells are a primary source of TNF in the reproductive tract, as well as oviductal epithelial cells of mice and women (Hunt et al. 1993; Bedaiwy et al. 2005; Strandell et al. 2004). TNF stimulates translocation of the nuclear factor kappa B (NFκB) transcription factors to the nucleus, subsequently triggering transcription of various inflammatory mediators such as IL6 and IL1α (Iwamoto and Konicek 1997; Malinin et al. 1997).
Mouse pre-implantation embryos were found to express the TNFR1 (p60) form of the TNF receptor (Pampfer et al. 1994b) and may secrete TNF under some circumstances (Lachapelle et al. 1993). TNF exerts detrimental effects on pre-implantation embryo development. For example, addition of TNF increases the percentage of apoptotic blastomeres in mouse (Byrne et al. 2002a; Fabian et al. 2007) and rat blastocysts (Pampfer et al. 1997). Soluble TNF production is elevated in diabetic rats and exposure to high levels of TNF appears to be a major contributor to poor fertility in this model (Pampfer et al. 1997). TNF administration to mice in early pregnancy impairs implantation and reduces litter size in mice and rats (Chaouat et al. 2004). Interestingly, the balance between pro- and anti-apoptotic factors is likely to be crucial in determining the outcome of TNF exposure, since SCF, IGFI and IGFII are protective against the inhibitory effects of TNF (Glabowski et al. 2005).
7.7.2 Interferon Gamma (IFNγ)
Interferon gamma (IFNγ) is a pro-inflammatory cytokine secreted into the uterine lumen during early pregnancy. It plays important roles in diverse cellular processes, including activation of innate and adaptive immune responses, inhibition of cell proliferation and induction of apoptosis (Boehm et al. 1997). Although IFNγ plays critical roles that include initiation of endometrial vasculature remodelling, angiogenesis at implantation sites, and maintenance of the decidual component of the placenta in normal rodent pregnancies (Murphy et al. 2009), elevated production of this cytokine is detrimental. IFNγ inhibits the secretion of GM-CSF and other cytokines that promote blastocyst growth and differentiation (Robertson et al. 1992), consistent with data showing that in the pre-implantation period IFNγ prevents implantation and is detrimental to the maintenance of pregnancy (Chaouat et al. 1990; Martal et al. 1997). The adverse effects of IFNγ are partly exerted directly on embryos, as the IFNγ receptor is expressed on mouse oocytes and pre-implantation embryos (Truchet et al. 2001) and IFNγ acts as an inhibitory factor in mouse embryo culture (Haimovici et al. 1988; Hill et al. 1992).
7.7.3 Tumor Necrosis Factor-Related Apoptosis-Inducing Ligand (TRAIL)
TRAIL and its receptor TRAILR (also known as death receptor, DR) are a death-inducing ligand and receptor pair that belong to the TNF and TNF-receptor superfamilies, respectively. Both TRAIL and TRAILR are expressed from the 1-cell through to the blastocyst stage of murine preimplantation embryo development, and embryos induced to express TRAIL or exposed to TRAIL undergo apoptosis (Riley et al. 2004). We have recently reported that TRAIL is expressed constitutively in mouse oviducts and attenuated during the periconceptual period when its expression is reduced in response to signals emanating either from the embryo or as a consequence of exposure to seminal fluid (Bromfield et al. 2014). Our unpublished data show that the bacterial product lipopolysaccharide (LPS) can induce TRAIL expression in oviducts and uterus , suggesting that TRAIL production may occur in the event of infection (PY Chin, SA Robertson unpublished data).
7.8 Maternal Tract Cytokines and Developmental Competence
The examples provided above show clearly that several cytokines produced by the maternal tract can influence embryo developmental competence and impart information beyond that supplied by factors produced endogenously by the embryo. This raises the question of the biological value of cytokine-mediated maternal tract—embryo communication.
7.8.1 Cytokines and Embryo Programming
The cytokine environment provided by the female reproductive tract at conception is implicated as a major factor in programming the later course of fetal and placental development. This early phase is a highly susceptible period for influencing later fetal and infant health (Thompson et al. 2005; Kwong et al. 2000; Sjoblom et al. 2005; Williams et al. 2011). Several stressors can act in the peri-conception period including immunological, infectious, nutritional, metabolic, physiochemical or even psychosocial perturbations—all of which exert similar subtle but permanent alterations in fetal development and the life-course trajectory of offspring. The capacity of an embryo to withstand and adapt appropriately to these stressors without substantial cost to later health is a key difference between a ‘viable’ embryo that survives, and an ‘optimally healthy’ embryo that thrives, and maternal tract cytokines contribute to the difference.
Cytokines affect at least two parameters impacting embryo programming—(i) apoptosis , which reduces cell number in the blastocyst, and (ii) epigenetic modification of the embryonic genome. Even small perturbations in blastomere number and inner cell mass/trophectoderm allocation in the blastocyst influence the growth trajectory of the fetus and offspring (Thompson et al. 2002). Changes in the epigenetic modification of imprinted genes Igf2, H19, Grb10 and Grb7 occur when mouse embryos are cultured in the presence of serum (Khosla et al. 2001) or in sub-optimal culture media deficient in cytokines (Doherty et al. 2000) each of which are linked with later growth changes. Fetal over-growth in cattle and sheep after embryo culture (the ‘large-offspring syndrome’) has been attributed to imprinting errors in Igf2r as a direct consequence of metabolic stress in culture (Young et al. 2001). Since expression of methyltransferases and other methylation machinery is cell cycle-regulated, disruption in their function is thought to occur following a slowing in the kinetics of embryo development in culture (De Rycke et al. 2002), whereas embryotrophic growth factors that protect blastomere survival and possibly advance cell division oppose this effect. In addition, cytokines can exert direct effects on the transcription of genes regulating methylation (Zhao et al. 2005).
The full range of effects of early cytokine exposure on later fetal and postnatal growth is not yet understood, since the majority of studies on cytokine-mediated effects during embryo culture are limited to the developmental end-point of the blastocyst stage embryo. In many cases, the consequences of cytokine treatments following embryo transfer have not been adequately explored.
One exception is the effect on fetal development and subsequent intergenerational effects of GM-CSF which, when added to mouse embryos in culture, influenced fetal and post-natal growth, and the likelihood of obesity in the adult progeny (Sjoblom et al. 2005). In mice, embryo culture itself is known to have adverse effects on the fetus that manifest as growth restriction in utero, rapid compensatory growth after birth, and larger body mass with increased fat deposits in adults. Addition of GM-CSF to mouse embryos prior to transfer was found to improve embryo implantation rate, correct deficiencies in placental structure and fetal growth trajectory, and partly alleviated the long-term adverse effects of embryo culture on postnatal growth (Sjoblom et al. 2005). This result showed that in vitro, GM-CSF exposure in the pre-implantation period is essential for programming optimal fetal development after implantation. A similar effect of GM-CSF is likely in vivo, since despite the normal abundance of other growth factors, mice that are genetically deficient in GM-CSF have compromised blastocyst development and reduced implantation rates (Robertson et al. 2001, 1999). Embryos that implant in GM-CSF knockout mice show fetal growth restriction and high rates of fetal loss and abnormality in late gestation and death in the perinatal period (Robertson et al. 1999), which may in part be the consequence of GM-CSF deprivation in the pre-implantation phase, but could also be attributable to direct effects of GM-CSF on placental morphogenesis.
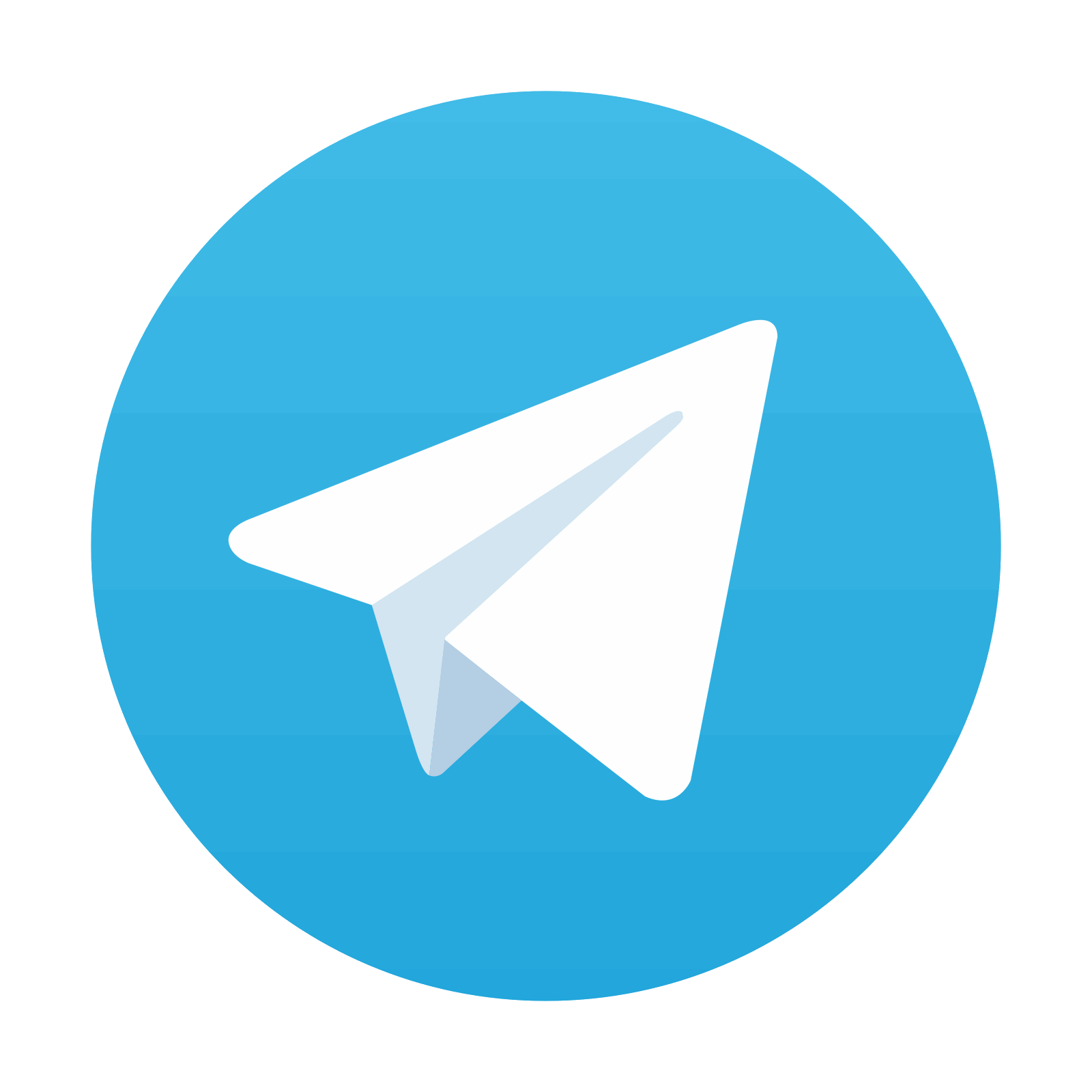
Stay updated, free articles. Join our Telegram channel

Full access? Get Clinical Tree
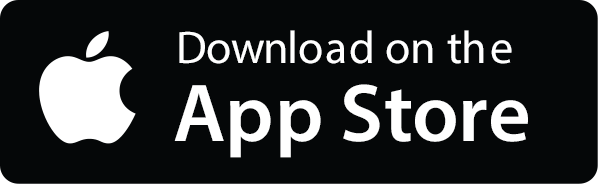
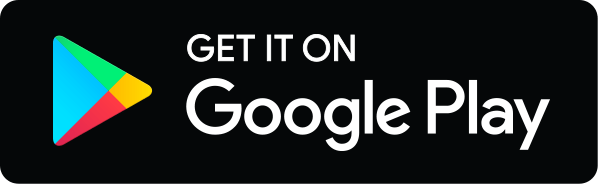