Year
Reference
Type of amyloid
Controls
Amyloid patients
Sensitivity (%)
Specificity (%)
PPV (%)
NPV (%)
1986
[15]
A, L, TTR
12
12
83
100
100
86
1987
[16]
A
81
65
82
100
100
87
1988
[17]
L
72
82
72
99
98
76
1988
[18]
A
80
44
75
100
100
88
1989
[19]
A, L, TTR
10
7
57
100
100
77
1989
[6]
A
18
26
54
100
100
60
1995
[20]
A, L
96
4
75
87
20
99
1997
[21]
A, L, TTR
89
11
82
100
100
98
2001
[22]
A, L
28
12
58
100
100
85
2004
[23]
A, L
62
20
75
92
75
92
2006
[24]
A, L, TTR
45
120
93
100
100
83
2007
[25]
A, L
30
14
78
93
84
90
Total
623
417
79
97
94
87
In a group of 450 patients with peripheral polyneuropathy, Congo red-stained fat tissue helps to detect amyloid only in patients with a monoclonal protein, a family history, or other clinical findings associated with amyloidosis and it is useless in patients without such a characteristic [28].
In patients with long-standing (more than 5 years duration) inflammatory diseases, such as rheumatoid arthritis and ankylosing spondylitis, abdominal fat aspiration may help to detect AA amyloidosis in a presymptomatic state or associated with only minor symptoms in 5–10 % [29–35] and sometimes in even 25–30 % of the patients [36, 37]. However, the clinical relevance of presymptomatic AA amyloidosis is not clear. Well-documented long-term (10–20 years) follow-up studies of such patients are lacking.
A recent study showed that the yield of a fat aspirate in diagnosing wild-type ATTR amyloid cardiomyopathy (84 patients) appears to be disappointedly low, i.e., sensitivity of 14 % [38]. Sensitivity can be increased to 73 % (8 of 11 patients), however, by careful looking for amyloid in the deep layer of surgically obtained subcutaneous fat tissue, where amyloid appears to be deposited in a patchy distribution [39]. Amyloid in blood vessel walls of fat tissue is seen more frequently in amyloid cardiomyopathy in AL amyloidosis than in ATTR amyloidosis in a small study of 14 patients [40].
There are only rare and incomplete data published about detection in fat tissue of types of systemic amyloidosis other than AA, AL, and ATTR [41], such as AApoAI (apolipoprotein AI) [42, 43], Aβ2M (β2-microglobulin) that is frequently absent or infrequently present as small deposits [44–47], AFib (fibrinogen α-chain) [48], AGel (gelsolin) [49–51], and ALys (lysozyme) amyloidosis [51]. Amyloid was easily detected in fat tissue of all three patients with AGel amyloidosis, we recently saw at our outpatient clinics (unpublished observation). We are currently not aware of any published data about amyloid in fat tissue of patients with AApoAII (apolipoprotein AII), AH (immunoglobulin heavy chain), and ALect2 (leukocyte chemotactic factor 2) amyloidosis. In this respect, it is important to mention AIns (insulin) amyloidosis. This rare localized type of nodular amyloidosis is an iatrogenic type of amyloid in which insulin-derived amyloid is deposited in abdominal fat tissue after long periods of repeated insulin injections in that particular site [52, 53]. A constant feature of this type of amyloid seems to be a negative immunohistochemical staining of serum amyloid P component (SAP) [53]. This insulin-derived amyloid is a potential pitfall in the diagnosis of systemic amyloidosis using aspirated fat tissue. Because long-standing diabetics can have hypertrophic cardiomyopathy, proteinuria, peripheral polyneuropathy, and autonomic neuropathy, symptoms and signs can be mistaken for systemic amyloidosis. Presence of monoclonal gammopathy may even confound the situation [54]. Therefore, in a patient with insulin-dependent diabetes mellitus, a Congo red-positive fat biopsy should always be treated with caution: either by making insulin-derived amyloid unlikely (e.g., by proteomics or immunohistochemistry) or by seeking additional conformation of systemic amyloidosis.
Typing of Amyloid
Antibody-Based Detection
Immunohistochemical staining of tissue biopsies is a standard technique used for typing amyloid. Orfila et al. used the immunofluorescence technique on abdominal fat tissue aspirates and detected five of nine samples with AA amyloid deposits [55] and Halloush et al. detected with immunoperoxidase technique three of five samples with AL and ATTR amyloid deposits [56]. Immunohistochemical staining of subcutaneous fat tissue, however, is fairly difficult due to nonspecific reactions [7]. Therefore, Westermark developed different immunochemical methods for typing amyloid in fat tissue. The initial method was a double immunodiffusion method that satisfactorily identified some patients with AA amyloidosis [4, 7]. Later his group developed an enzyme-linked immunosorbent assay (ELISA) that was useful for typing AA, AL, and ATTR amyloidosis in 14 of 15 cases [8]. Recently he described a method based on Western blot analysis combined with specific amyloid fibril antibodies that was successful in typing 32 of 35 patients with AA, AL, and ATTR amyloidosis [49] and all of 33 patients with ATTR amyloidosis [57]. Kaplan et al. also used immunochemical methods to type amyloid successfully in three of four patients with AL-kappa, in five of six patients with AL-lambda, and in one patient with AA amyloid [58].
Because of unsatisfactory typing of amyloid in fat aspirates using immunohistochemistry, Arbustini et al. developed immunoelectron microscopy for typing amyloid of patients with cardiac amyloidosis. This appeared to be a promising technique, because in all 15 patients the amyloid types involved were identified: eight samples with AL-lambda, two with AL-kappa, three with ATTR, two with AApoAI, and none with AA amyloid [42]. In a recent study of this group, immunoelectron microscopy was used for typing amyloid in abdominal fat tissue in 423 patients. The specific type of amyloidosis was correctly identified in 99 % of the cases. An abstract of this study has been presented at the XIVth International Symposium on Amyloidosis in Indianapolis in April 2014 [59, 60].
Antibody-Based Quantification of Amyloid Proteins
Our group in Groningen also developed and used immunochemical methods for measuring the concentrations in fat tissue of the four major amyloid proteins, i.e., amyloid A protein, TTR, light chain kappa, and light chain lambda. See Table 16.2 for the panel of cutoff values we use for fat aspiration samples in our center. An ELISA was used to measure the concentration of amyloid A protein in fat tissue in a group of 24 patients with AA amyloidosis and a group of 72 controls, including 25 patients with AL or ATTR amyloidosis and 25 patients with chronic inflammation. The upper limit of the 99 % CI of controls (11.6 ng/mg tissue) was chosen as cutoff level [10]. In a large collaborative international study, this method was validated and identified 129 of 154 patients with AA amyloidosis, whereas only 3 of 354 controls (87 AL, 30 ATTR, and 27 localized amyloidosis and 210 non-amyloidosis patients) were identified incorrectly [61]. Thus, in this study the sensitivity of detecting AA amyloidosis was 84 % (95 % CI 77–89 %), specificity was 99 % (95 % CI 98–100 %), PPV was 98 % (95 % CI 94–100 %), and NPV was 93 % (95 % CI 90–96 %). Men had lower amyloid A protein concentrations in fat than women (Fig. 16.1a). Patients with familial Mediterranean fever (FMF) had lower values than patients with arthritis or other inflammatory diseases (Fig. 16.1b). The low yield of fat tissue aspirates for the detection of AA amyloidosis in patients with FMF was already observed and discussed by Tishler et al. in 1988: they even did not detect amyloid in any of the 15 FMF patients studied [62]. However, Congo red-positive amyloid deposits were detected in 20 fat tissue samples (80 %) and the amyloid A protein concentration was elevated in 13 samples (about 50 %) of the 25 FMF patients we studied [61]. In a study of Egyptian patients with long-standing rheumatoid arthritis, quantification of the amyloid A protein concentration in fat tissue appeared to be a useful screening tool for the detection of AA amyloidosis [32]. Subcutaneous fat tissue is a major source of the acute-phase reactant serum amyloid A protein (SAA), the protein precursor of AA protein [63]. Extensive washing of fat tissue, however, was effective to prevent unwanted contamination and retention of SAA from blood in fat tissue extracts of controls. Although indeed a positive correlation between the SAA concentration in blood and amyloid A protein concentration in fat tissue was present in controls, the magnitude of the effect was very small, resulting in maximum amyloid A protein concentrations in fat tissue largely within the reference range of controls [61].

Table 16.2
Immunochemical quantification of the four major amyloid proteins in subcutaneous abdominal fat tissue: cutoff values (Groningen), sensitivity, and specificity
AA | ATTR | AL-κ | AL-λ | |
---|---|---|---|---|
Cutoff value | ||||
Amyloid A protein (ng/mg fat) | >11.6 | |||
TTR (ng/mg fat) | >4.36 | |||
κ/λ ratio | >5.86 | <0.49 | ||
Patients (number) | 154 | 49 | 23 | 84 |
Controls (number) | 354 | 204 | 95 | 95 |
Specificity (%) | 99 | 99 | 97 | 97 |
Sensitivity (%) | 84 | 71 | 78 | 70 |

Fig. 16.1
Amyloid A protein concentration in fat tissue of patients with clinical AA amyloidosis. The dotted line marks the upper reference limit of controls (11.6 ng/mg fat). Asterisks (*), (**), and (***) represent p < 0.05, p < 0.001, and p < 0.0001, respectively. The horizontal lines denote the means of the log-transformed values. (a) Men (N = 63) and women (N = 91). (b) Patients with chronic arthritis (N = 93), patients with FMF (N = 25), and patients with other diseases or more than one disease (N = 36). (c) Congo red (CR) stain scores of fat tissue: CR negative (N = 14), CR 1+ (N = 25), CR 2+ (N = 34), CR 3+ (N = 55), and CR 4+ (N = 45) (Reproduced with permission of Informa UK Ltd. [61])
An ELISA is also used in our center to measure the concentration of TTR in fat tissue. The upper limit of the 99 % CI of controls is 4.36 ng/mg fat tissue and this value has been chosen as cutoff value. Quantification of free light chains by nephelometry is used to measure light chain kappa (95 % CI upper limit 24.4 ng/mg fat tissue), light chain lambda (95 % CI upper limit 10.6 ng/mg fat tissue), and the resulting κ/λ ratio (95 % CI reference range 0.49–5.86). See also Table 16.2 [64, 65].
Advantages and Disadvantages of Antibody-Based Typing
Antibody-based detection of amyloid has the advantage of fast, inexpensive, and specific detection and amplification of minute amounts of a particular protein in an enormous background ocean of other proteins and tissue components. However, disadvantages are sometimes considerable: limited reactivity with commercial antibodies (false-negative), “contamination” by serum proteins (false-positive), the increasing number of other types of amyloidosis that are recognized, the issue of combined deposits (more than one type of amyloid protein present), and finally, experience and principles in the interpretation of results [66]. The consequences of mistyping amyloid may lead to a wrong choice of treatment and may be harmful for the patient. This is the main reason we wanted to develop in our center typing methods with high specificity (≥99 %) resulting in a high PPV. If a particular type is then successfully identified in a sample, the result should be virtually beyond doubt. The practical consequence of very high specificity on the other hand is relatively low sensitivity with corresponding low NPV. However, it is our opinion that it is easier to manage cases in which typing is not successful: these inconclusive or negative cases should be diagnosed as such and considered for evaluation by reference laboratories that have experience in amyloid typing using a wider antibody panel as well as the capacity to apply methods that are more sophisticated [66].
Proteomics-Based Detection
Typing of amyloid should be performed with confidence [67]: amyloid typing should result in certainty of both presence of a particular type of amyloid and absence of all other (relevant) types of amyloid. Chemical typing of the amino acid sequence of the amyloid protein involved has been the gold standard for a long time. Until recently, macropurification techniques were needed for isolation of these proteins from large amounts of tissue obtained at necropsy or after surgery, at least 10–30 g of amyloid-rich tissue [68, 69]. With some modifications, Westermark et al. were able to determine the amino acid sequence of the amyloid protein isolated from only 1 g of amyloid-rich fat tissue [70, 71] and 10 years later from an even lower amount of fat tissue [72].
New methodological approaches, including the search for appropriate extraction solvents and separation strategies, have been developed and tested in order to design efficient procedures for small scale isolation and purification of these amyloid proteins [73–75]. Kaplan et al. were among the first to investigate these micropurification techniques and they found that the strategy for small scale purification of amyloid proteins depends largely on the type of tissue sample (fresh or formalin fixed), sample size, amyloid content of the tissue, and its chemical nature [75]. Abdominal fat tissue aspirates were among the tissues they studied [58]. These techniques were further improved using different mass spectroscopic techniques in the following years [51, 76–79]. Vrana et al. introduced laser microdissection to concentrate the amyloid content in extracts from tissue samples of patients with AA, ATTR, AL-kappa, and AL-lambda amyloidosis [79]. Her group recently applied mass spectrometry-based proteomics to type amyloidosis in fat aspirates [51]. In the validation study, the presence of amyloid was detected with high sensitivity (88 %) and specificity (96 %). In 366 Congo red-positive cases, mass spectrometry analysis successfully identified an amyloid subtype in 330 cases, yielding a sensitivity of 90 %. The specific diagnoses were AL-lambda (58 %), AL-kappa (16 %), ATTR (12 %), AA (1 %), AGel (1 %), AIns (1 %), and ALys (1 %). A universal amyloid proteome signature (i.e., the universal presence of apoAIV, ApoE, and SAP) was identified with high sensitivity and specificity, similar to that of Congo red staining [51].
Lavatelli et al. developed a proteomics methodology utilizing two-dimensional (2D) polyacrylamide gel electrophoresis followed by matrix-assisted laser desorption/ionization mass spectrometry and peptide mass fingerprinting to directly characterize amyloid deposits in abdominal subcutaneous fat obtained by fine needle aspiration from seven patients with AL amyloidosis, two with ATTR amyloidosis, and seven controls. Striking differences in the 2D gel proteomes of adipose tissue were observed between controls and patients and between the two types of patients with distinct, additional spots present in the patient specimens that could be assigned as the amyloidogenic proteins in full-length and truncated forms [80]. The 2D-tandem MS technique was further developed (called Multidimensional Protein Identification Technology or shortly MudPIT) and was used to identify reliably 26 patients with the most common (AL, AA, and ATTR) types of amyloidosis who had grade 3+ or 4+ amyloid in fat tissue [81]. In another study of 30 patients with systemic amyloidosis, comprehensive protein profiles of human amyloid-affected adipose tissue from patients and its control (nonamyloid affected) counterpart were acquired. In particular, extracellular matrix (ECM), protein folding, lipid metabolism, and mitochondrial functions were among the most affected structural/functional pathways [82]. These proteomic techniques have great potential to diagnose and type amyloid, but also to clarify all kind of molecular mechanisms involved in amyloidosis [82–84].
Semiquantitative Assessment of Amyloid in Fat Tissue
Amyloid deposition in tissue is the result of the balance between accumulation and breakdown of amyloid. Progressive deposition of amyloid in a vital organ or tissue generally leads to loss of function of that organ or tissue. The mechanism behind this loss of function is not satisfactorily explained and both mere physical hindrance and toxic effects may play a role. Regression of amyloid deposition sometimes results in (partial) recovery of function of the organ or tissue involved. It is generally believed that continuous and massive supply of available amyloid protein precursors leads to accumulation of amyloid, whereas complete lack of supply of these precursors may facilitate endogenous breakdown of amyloid leading to regression of amyloid. Monitoring the intensity of amyloid deposition in tissue might help to understand more about the balance between accumulation and breakdown of the total amyloid load of the body. Screening of subcutaneous abdominal fat tissue seems to be a suitable tool for this purpose because this tissue is easily available by aspiration, amyloid can almost always be found if present, and aspiration can be repeated at intervals during the course of the disease. This was the background of our ambition to develop tools for measuring the intensity of amyloid deposition in fat tissue. A scoring system was developed that semiquantitatively graded the amount of amyloid in fat on a scale of 0 to 3+ [10, 32]. Severity was assessed by visual estimation of the percentage of the smear surface area affected by deposition of amyloid recognized in polarized light [32]. Later we widened the scale from 0 to 4+ and validated this scale (Fig. 16.1c) using AA protein quantification in a large group of patients with AA amyloidosis [61]. Figure 16.2 shows typical examples of this semiquantitative grading system: 0 (negative, no apple-green birefringence detectable), 1+ (minute, <1 % of surface area), 2+ (little, between 1 % and 10 %), 3+ (moderate, between 10 % and 60 %), 4+ (abundant, >60 %). Kimmich et al. demonstrate how this technique reliably can be introduced and implemented with confidence in another center [85].


Fig. 16.2
Congo red-based grading system of intensity of amyloid deposition in fat tissue (Reproduced with permission of Informa UK Ltd. [61])
Gómez-Casanovas et al. introduced in 2001 a comparable semiquantitative scoring system of Congo red-stained specimens and graded them as 3+ (marked), 2+ (moderate), and 1+ (mild) according to the amount of amyloid deposits. Marked refers to massive amyloid deposition in >25 % of the tissue fragments sampled and/or linear deposits in >75 % of fragments, moderate refers to massive amyloid deposits in <25 % of fragments or linear deposition in >25 % of fragments, and mild refers to linear deposits in <25 % of fragments. Very small and isolated Congo red-positive deposits were considered inconclusive and were classified as negative. Marked amyloid deposits were found more frequently in patients with clinical amyloidosis than in those whose amyloidosis remained subclinical [86].
In this respect, it is interesting to mention the study of Bardarov et al. who describe the development of a generally available computer-assisted image analysis of Congo red-stained amyloid deposits in abdominal fat tissue biopsies [87]. Further improvement of such techniques will make it easier and less subjective not only to detect amyloid but also to (semi)quantify the amount of amyloid present.
Quantification of Amyloid in Fat Tissue for Diagnosing and Typing Amyloid
Semiquantitative grading of amyloid in fat tissue may be helpful in diagnosing amyloidosis with confidence. Dhingra et al. propose that patients with grade 1+ of amyloid in fat tissue should not undergo a toxic therapeutic regimen on the basis of only this result. In this situation, they advise histologic confirmation of visceral amyloid deposition in deeper tissue [25].
Grading the amount of amyloid in fat tissue may be helpful in typing amyloidosis with confidence. All patients with grade 3+ or 4+ and 90 % of patients with grade 2+ AA amyloid (as shown in Fig. 16.1c) have an elevated amyloid A protein concentration in fat tissue [61]. This is true for only half of the patients with grade 1+ amyloid. Comparable results have been observed in patients with ATTR and AL amyloidosis [64, 65]. All patients with grade 3+ and 4+ ATTR amyloid had an elevated TTR concentration in fat tissue, whereas this was true for only half of the patients with grade 2+ and almost none of the patients with grade 1+. All patients with grade ≥2+ AL-kappa amyloid had an elevated κ/λ ratio in fat tissue, whereas only 58, 78, and 90 % of the patients with grade 2+, 3+, and 4+ AL-lambda amyloid, respectively, had a decreased κ/λ ratio in fat tissue. We conclude that in nearly all patients with grade 3+ and 4+ amyloid in fat tissue immunochemical quantification enabled us to type AA, ATTR, AL-kappa, and AL-lambda with confidence. The chance of reliable typing of patients with grade 2+ amyloid is much lower (about 50 %) and in only a minority of patients with grade 1+ (about 10 %) immunochemical typing will be successful.
Amyloid Quantity in Fat Tissue and Clinical Characteristics
In patients with AA amyloidosis, amyloid was detected in fat tissue in 95 % of women and in 90 % of men [61]. Although this difference was not statistically significant, the grade of amyloid was higher in women (median 3+) than in men (median 2+). As stated earlier (Fig. 16.1a), the concentration of amyloid A protein in fat tissue was higher in women (geometric mean 315 ng/mg) than in men (geometric mean 63 ng/mg). This difference of amyloid quantity in fat tissue between men and women was confirmed in another study of 220 patients concerning all major types of systemic amyloidosis [88]. A satisfactory explanation of this increased deposition in women or decreased deposition in men of all types of amyloid in fat tissue is lacking. Amyloid grade in fat tissue was associated with the number of major organs involved and was a predictor of decreased survival (Fig. 16.3), independent of other predictors such as heart involvement, the number of organs involved, type of amyloid, and age [88]. These data support the notion that, at least at group level, the amount of amyloid in subcutaneous fat tissue in systemic amyloidosis reflects disease severity.


Fig. 16.3
Kaplan-Meier survival curves of equally sized groups for grades of amyloid in fat tissue (Reproduced with permission of John Wiley and Sons [88])
Amyloid Quantity in Fat Tissue and Follow-Up
If the amount of amyloid in fat tissue indeed reflects disease severity, it may be interesting to study the effect of treatment on amyloid deposition in fat tissue. Only a few follow-up studies of amyloid in fat tissue have been published. In one study of patients with ATTR amyloidosis, fat tissue was monitored in nine patients before liver transplantation and in 11 patients thereafter [89]. When a change of at least two grades of amyloid in fat tissue was used as marker of significant progression or regression, no regression was seen whereas progression was seen in two of three patients followed for at least 7 years without transplantation. After transplantation, none of the patients showed progression and only one showed regression of amyloid in fat tissue. The numbers in the study were too small to draw any firm conclusion, but one may conclude that without liver transplantation some progression can be detected after 7 years and that significant improvement following transplantation does not occur in most patients within 5 years. However, significant improvement may occur more than 10 years after transplantation as shown in one study of six ATTR patients in whom amyloid (almost) completely regressed in fat tissue [90].
Not only the quantity, but also the composition of ATTR-V30M amyloid in fat tissue of patients with a TTR-Val30Met mutation, is clinically relevant as has been shown in some studies before and after liver transplantation [57, 90–92]. Fibrils composed of only full-length TTR were associated with early age at onset, no clinical cardiac involvement and a strong affinity for Congo red. In contrast, the presence of TTR fragments in the amyloid was associated with late age at onset, signs of cardiac involvement and weak Congo red staining [57]. In a study of 63 patients carrying 29 different TTR non-V30M mutations a fibril composition with fragmented ATTR appears to be very common in ATTR amyloidosis, and suggests that fibrils composed of only full-length ATTR is an exception found only in a subset of patients [93]. In another study of 44 patients with ATTR amyloidosis without liver transplantation, proteomic analysis showed that the percentage of wild-type TTR, i.e., nonmutated TTR, was higher in patients older than 50 years than in patients younger than 50 years, i.e., 51 % vs. 31 %, respectively [91]. Wild-type TTR may be more strongly amyloidogenic than previously considered because it is detectable even in amyloid of young ATTR patients. The percentage of wild-type TTR in amyloid in fat tissue samples increased significantly to 90–100 % after liver transplantation compared to pretransplantation values of 35–60 % [90, 92]. Fat tissue was analyzed in 32 other patients with ATTR amyloidosis of whom 20 had received a liver transplant [91]. In pretransplant patients, it was found that cardiac amyloid more easily incorporated wild-type TTR than fat tissue amyloid, offering a potential explanation for the vulnerability of cardiac tissue for continued amyloidosis after liver transplantation. In fat tissue, a rapid increase in wild-type TTR proportion after liver transplantation indicates that a rather fast turnover of the deposits occurs. Some difference in wild-type TTR proportion between full-length TTR and fragmented TTR fibril types was seen after transplantation but not pretransplantation, possibly caused by differences in turnover rate [92].
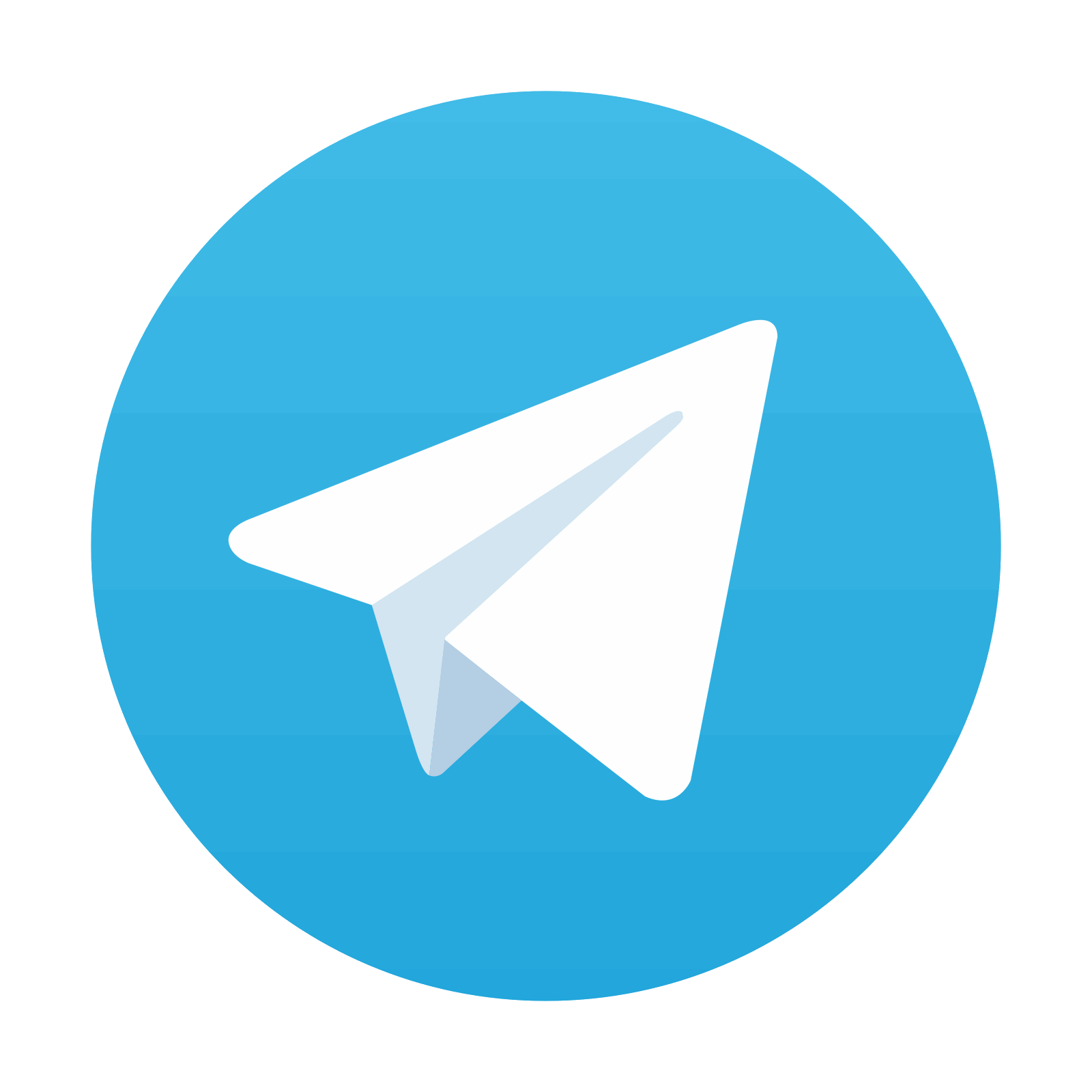
Stay updated, free articles. Join our Telegram channel

Full access? Get Clinical Tree
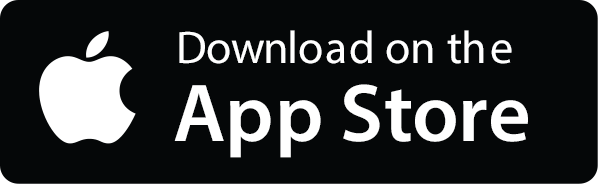
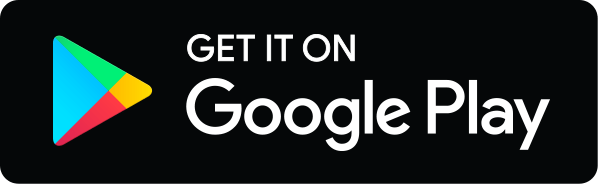