Introduction
In 1976, Dr. Robert Bartlett and his colleagues first reported successful results from the use of extracorporeal membrane oxygenation (ECMO) in neonates.1 As methodology improved to form the backbone of ECMO practices today, many more studies and publications were written. Advancements in pump technology from roller to centrifugal mechanisms as well as development of hollow-fiber oxygenators have propelled ECMO science forward. Improvements continue moving toward a more awake patient who is easier to assess. Numerous extrapolations of these advancements have been adopted in the pediatric and adult populations, and their use in neonatal circles has become a treatment mainstay of many diseases of the newborn.
The Extracorporeal Life Support Organization, an international group of subject matter experts in ECMO, estimates that 1 out of every 1,309 live U.S. births could benefit from ECMO annually.2 Neonates tend to develop respiratory failure as a result of immaturity, airway anomalies, or abnormalities of pulmonary circulation. Coupled with the limitations of conventional ventilator practices, this created the need for a different approach to treat diseases (e.g., congenital diaphragmatic hernia, meconium aspiration, respiratory distress syndrome).3 Additionally, congenital cardiac defects often require support for a neonatal cardiopulmonary system as a bridge to surgical repair, a recovery mechanism postoperatively if weaning from cardiopulmonary bypass fails or as a stopgap measure while awaiting cardiac transplantation. The latter is perhaps a population waning in size because data are emerging that indicate bridging with a ventricular assist device may result in improved outcomes post-transplant.4,5
Veno-venous ECMO
ECMO utilizes an external pump, oxygenator, and heat exchanger to provide a modified form of heart−lung bypass, and the method of ECMO selected for a patient depends on the support required for the diagnosis. For patients requiring pulmonary support, veno-venous (VV) ECMO is initiated where blood is removed from a large vein, circulated external to the patient (to provide oxygenation and removal of carbon dioxide), warmed, and returned via a vein. Oxygen is delivered via a blender, and sweep gas flow allows removal of carbon dioxide via rapid diffusion according to Fick’s law.6 Cannulation, performed by a surgeon, commonly involves the right internal jugular vein and spares the arteries. Venous drainage often limits flow in VV ECMO, and two-site venous drainage can be used to decrease recirculation. Cannulae can be placed percutaneously or by venous cutdown.
Veno-arterial ECMO
Conversely, in patients with impaired cardiac physiology requiring full cardiopulmonary support, veno-arterial (VA) ECMO is the selected methodology where blood return is conducted via an artery. The flow provided via the ECMO pump supports cardiac output, bypassing the entire cardiovascular system and decreasing cardiac work and oxygen demand. Cannulae for VA ECMO are typically placed in the right internal jugular vein (providing access to the right atrium) and right common carotid artery (providing access to the aortic arch), often resulting in sacrifice of the aforementioned artery. The side-graft technique can be employed for arterial return in cases when artery ligation presents complications; some institutions have reported success in reconstruction of the artery post-decannulation. Complication rates in VA ECMO exceed those in VV ECMO and are most frequently associated with systemic emboli.7 However, approximating normal left heart filling pressures assists in the correction of pulmonary edema.
Inclusion Criteria and Indications
Inclusion criteria for neonatal ECMO vary by center but largely include
gestational age > 34 weeks
weight approaching 2 kg
presence of a reversible process
absence of lethal anomaly, uncorrectable defect, uncorrectable coagulo-pathy, or major intracranial hemorrhage
Gestational age limits are often based on the required systemic heparinization required during ECMO and the reported rates of intracerebral hemorrhage and mortality related to premature infants.8 However, a retrospective review of these early ECMO patients concluded that with further improvements in technique, lowering the gestational age requirement may be possible.9 The weight requirement of 2 kg is determined by two factors: the lack of availability of ECMO cannulae smaller than 8 French and the resultant flow limitations of such a small catheter. In 2012, Lazar and colleagues published a report of successful use of a 13 French dual-lumen bicaval catheter in nine neonates, weight range of 2.2 to 5.5 kg, and a survival rate of 56%.10 However, some centers have reported delayed atrial perforation with this particular catheter, which may limit its use in younger patients.11 Hermon and colleagues compared two-site cannulation with double lumen ECMO and found similar outcomes in the two groups.12 Further work is needed to investigate optimal cannulation techniques to maximize ECMO flow while minimizing complications in premature neonates.
A common criterion to ECMO progression is referred to as the failure of optimal medical management, although the definition of “optimal” varies by center and often by provider. Most agree that modalities including maximal pharmacologic support, high frequency oscillatory ventilation, and use of nitric oxide should be trialed, where appropriate, prior to the decision to cannulate. Wung et al. reported successful medical management of persistent pulmonary hypertension in 15 patients who met institutional criteria for ECMO.13 Additionally, Hintz and colleagues demonstrated a decrease in ECMO utilization as newer treatment modalities were invoked for hypoxemic respiratory failure.14 As more data are published, the inclusion criteria for ECMO may be modified to maximize less invasive, more targeted therapies. Data are emerging that document the use of ECMO in many disease states with positive mortality results. For example, a German center’s 20-year experience with neonatal ECMO showed increasing numbers of patients, a preponderance of patients with congenital diaphragmatic hernia, and a survival-to-discharge rate of 67% (with the best rates seen in meconium aspiration syndrome).15
Changes on ECMO
Organ System Changes
Most organ systems are affected by the initiation of ECMO, and changes should be anticipated and managed proactively to maximize positive outcomes. Although the cardiovascular system is supported on ECMO, it must still be attended to during therapy. Ideally, intravascular volume should be maintained to facilitate flow through the circuit. Native cardiac output can also be supplemented by the use of pharmacologic inotropes, with milrinone being the most commonly used in this population.
Pulmonary System
This system is supported relative to the modality of ECMO utilized. In VA ECMO, minimal flow enters the pulmonary circuit and, therefore, rest settings sufficient to maintain expansion and functional residual capacity are optimal through appropriate levels of positive end-expiratory pressure (PEEP). Conversely, VV ECMO ventilator settings may require adjustment throughout the course of treatment because gas exchange from the native pulmonary system contributes to a greater degree than on VA ECMO.16
Central Nervous System
This system is at significant risk in the neonate, so frequent assessment is helpful in early identification of changes in mental status or seizures. Most centers are moving away from chemical paralysis to facilitate frequent evaluation of neurologic status. Serial cranial ultrasounds can offer assistance too. Neurologic complications result in increased mortality, with risks correlating to patient factors, pre-ECMO illness severity, and use of VA ECMO.17 Neonates have the highest rate of adverse long-term neurological sequelae from ECMO, including intraventricular hemorrhage and neurologic infarction.18,19
Hematologic System
This system is affected by the presence of a large, nonbiological circuit that triggers an inflammatory reaction; acute changes in the complete blood count and other hematologic markers must be recognized quickly. Mitigating reductions in hemoglobin levels and platelet counts with frequent transfusions are critical to maintenance of oxygen-carrying capacity and hemostasis, respectively. Thrombosis is prevented through the use of a continuous infusion of unfractionated heparin titrated to an activated clotting time congruent with the institution’s ECMO protocol. This is typically 180 to 200 seconds with some centers allowing patients to trend upward toward 240 seconds. Antithrombin III levels often drop during ECMO and require supplementation, either with infusion of fresh frozen plasma or recombinant antithrombin III alone.
Renal System
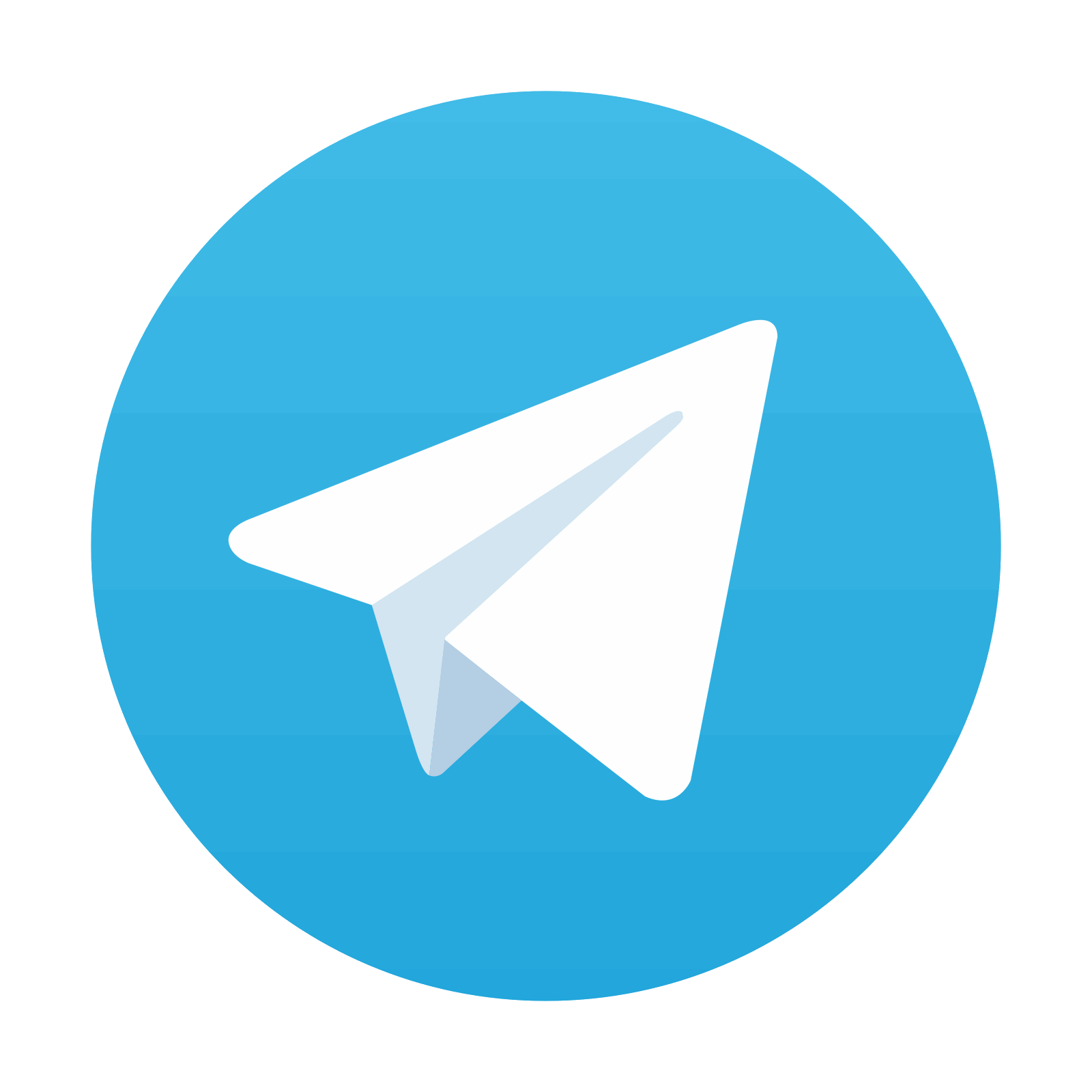
Stay updated, free articles. Join our Telegram channel

Full access? Get Clinical Tree
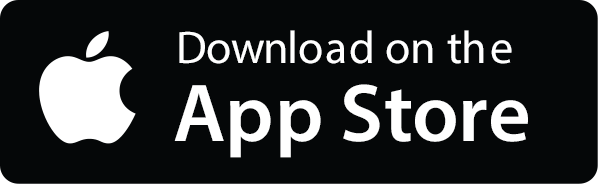
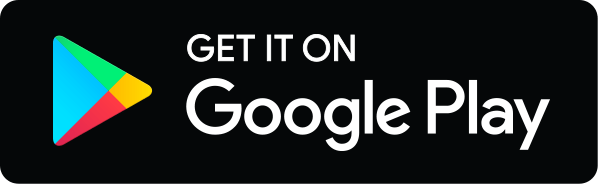