CHAPTER 93 Exercise Electrocardiography (Stress) Testing
For the diagnosis of CAD, although much money, time, and effort have been spent designing and applying other tests with greater sensitivity, the sensitivity of EET exceeds 90% (perhaps 95%) for significant CAD—meaning left main CAD or multivessel disease with resultant left main equivalent CAD (i.e., left ventricular [LV] dysfunction resulting from ischemia). Supporting this, several studies have examined the incremental value of exercise myocardial perfusion imaging compared with EET for diagnosis and risk stratification of CAD. In an analysis of these studies, the modest incremental benefit of imaging did not appear to justify its cost (which has been estimated at $20,550 per additional patient correctly classified). Consequently, the American College of Cardiology (ACC) and the American Heart Association (AHA) recommend a stepwise strategy for diagnosing CAD in patients with an intermediate pretest likelihood, using an EET as the initial test, and not an imaging procedure, if the patient is able to exercise, has a normal resting ECG, and is not taking digoxin* (ACC/AHA/ASNC Guidelines). (See chapter 94, Stress Echocardiography, for testing patients with an abnormal resting ECG or who are taking digoxin, and for a dobutamine echo protocol for patients unable to exercise.)
With medical management of CAD more successful than ever, primary care clinicians’ skills in managing CAD have become more important. Performing EET is one method of maximizing these skills. In fact, using the Duke Treadmill Score (DTS) or nomogram for positive tests, primary care clinicians can now risk-stratify and prognose CAD as well as their cardiologist colleagues. It should be noted that in the latest guidelines (2002, 2007) from AHA/ACC for management of stable CAD, an EET is a reasonable test for risk stratification in patients with a normal resting ECG and who are able to exercise. In part, these guidelines are based on “the simplicity, lower cost, and widespread familiarity with the performance and interpretation of the standard EET.” Also, when patients divided into risk groups using EET have been studied with imaging (Gibbons and colleagues, 1999), few patients (<5%) who have a low-risk DTS (≤1% annual cardiac mortality rate) will be identified as high risk after imaging, and thus the cost of identifying these patients again argues against routine imaging. Those patients identified as high risk (≥3% per year annual cardiac mortality) should probably be referred directly for cardiac catheterization and a possible intervention (again, see Chapter 94, Stress Echocardiography). Only those patients with an intermediate DTS (>1% and <3%) seem to benefit from an imaging study to further differentiate low-risk patients from those who might benefit from an intervention.
Performing a maximal EET provides additional information valuable for predicting prognosis, such as exercise capacity and heart rate in recovery (HRR, explained later), even if the ECG cannot be used for interpretation or predicting prognosis. From a study of 7163 patients (Diaz and colleagues, 2001) with known or suspected CAD undergoing myocardial perfusion imaging at the Cleveland Clinic and for whom a DTS could not be calculated (e.g., patient taking digoxin, resting ECG abnormalities), the independent prognostic values of exercise capacity and HRR were determined. Patients were followed for an average of 6.7 years, and, when compared with results of myocardial perfusion imaging, not only did exercise capacity and HRR provide additional prognostic information, but if exercise capacity and HRR were both abnormal, it portended a higher risk of mortality than the result from the myocardial perfusion test in nonrevascularized patients.
Physiology of Exercise ECG Testing
Basal oxygen consumption, or 1 metabolic equivalent (1 MET), defines the amount of oxygen an average individual consumes sitting at rest, which is approximately 3.5 mL/kg/min (i.e., 1 MET = 3.5 mL/kg/min O2). is often quantified as a multiple of the basal oxygen consumption in METs. For instance, walking 2 miles per hour (mph) on level ground requires approximately 2 METs. Walking 4 mph on level ground requires approximately 4 METs. Moderately active young men usually have a
of at least 42 mL/kg/min, or 12 METs. This means they are able to consume 12 times the amount of oxygen that they consume at rest. Obviously METs can also be used as a conversion factor between types of exercise. Charts are available (Fig. 93-1) to estimate a patient’s exercise capacity or maximal METs by cross-referencing MET levels with different daily activities. This estimate may then be used to predict performance before placing a patient on a treadmill. Performing a maximal EET remains one of the more accurate methods of estimating maximal METs. An individual’s maximal METs, whether determined on a bicycle or a treadmill, has significant management and prognosis implications if the person has CAD. Even if the test is positive for CAD, achieving certain MET thresholds can be very reassuring for prognosis.
During a maximal EET, a perceived exertion scale (PES) may be helpful for monitoring the patient. The PES is similar to a pain scale; however, the patient quantifies “effort” or “exertion” instead of “pain” during testing. Originally studied and published with a scale from 5 to 20 (with 20 being the subjective maximum that an individual could work and 5 being minimal or no work at all), it has now been modified. Most clinicians in the United States use a range from 1 to 10 (Box 93-1) for the PES. Although it is a subjective measurement, in most patients the PES level is very reproducible; in other words, if they were asked to repeat the EET in 2 weeks, they would report the same PES level at the same workload and duration for both EETs. Interestingly, their heart rate is also usually very similar at a given PES. As a result, a PES target can be given to most patients to use as part of their exercise prescription. This replaces the patient’s need to check the heart rate during exercise, and anything that simplifies an exercise prescription will, it is hoped, increase the chance of adherence/compliance.
Box 93-1 Perceived Exertion Scale
0 | Nothing at all |
0.5 | Very, very weak |
1 | Very weak |
2 | Weak |
3 | Moderate |
4 | Somewhat strong |
5 | Strong |
6 | |
7 | Very strong |
8 | |
9 | |
10 | Very, very strong |
With exercise-induced ischemia, ST segment depression becomes a global phenomenon, meaning it involves the entire subendocardium. When one area of the subendocardium becomes ischemic, there is a “fail-safe” mechanism that responds, meaning this subendocardial segment reduces its workload or shuts down before becoming permanently damaged. As a result, the remaining and surrounding subendocardium must work harder to compensate. This surrounding area subsequently becomes ischemic, and a domino effect occurs, cascading around the entire subendocardium. Consequently, ST segment depression is usually seen in multiple leads. In fact, this is such a global phenomenon that the coronary vessels involved cannot be predicted by which of the leads are demonstrating ST segment depression. (If knowledge of the coronary vessels involved or the total burden of ischemia is needed, an imaging test [myocardial perfusion or echocardiogram, see Chapter 94, Stress Echocardiography] should be added to the stress test.)
If the entire wall of the myocardium (full thickness) becomes ischemic, such as from severe CAD, ST segment elevation may be noted. It may appear very similar to that seen with a transmural/ST-elevation infarct or a ventricular aneurysm. In this situation, unless the clinician is certain that the finding is due to a ventricular aneurysm (e.g., associated with Q waves, or the clinician previously diagnosed/managed the infarct), the EET should be stopped. If either transmural ischemia or a new infarction is occurring, the potential for an arrhythmia is very high. However, transmural ischemia is rare in a community setting. In almost all positive EETs, ST segment depression is what is noted, the same as with what used to be called a subendocardial infarct. The subendocardial layer is usually the first to become ischemic because it is the “watershed” area of the heart, or the farthest from the arteries that are located in the epicardium (Figs. 93-2 and 93-3).

Figure 93-2 Normal myocardial perfusion. Note perfusion gradient is from 80 mm Hg to 5 to 10 mm Hg.
(Redrawn from Ellestad MH: Stress Testing: Principles and Practice, 4th ed. Philadelphia, FA Davis, 1996.)
Normal Clinical Responses to Exercise ECG Testing
Indications
General Indications

Figure 93-4 Pretest likelihood of coronary artery disease (CAD).
(Redrawn from Diamond GA, Forrester JS: Analysis of probability as an aid in the clinical diagnosis of coronary-artery disease. N Engl J Med 300:1350–1358, 1979.)
NOTE: For diagnosing CAD, determining pretest likelihood is important. This is explained for patients with symptoms by examples in the section “Determination of Pretest Likelihood.”
Specific ACC/AHA Indications
For the sake of completeness of this chapter, most of the indications for EET in adults from the ACC/AHA guidelines will be listed. Guidelines for special cases (e.g., children, EET with expired ventilatory gas analysis) may be found in the ACC/AHA reference or on the AHA website (www.americanheart.org), under “Science and Professional, Scientific Publications, Scientific Statements.” The ACC/AHA guidelines use the following classification system for indications:
Exercise ECG Testing in Diagnosis of Obstructive CAD

Risk Assessment and Prognosis in Patients with Symptoms or a History of CAD
Exercise ECG Testing after Myocardial Infarction
Exercise ECG Testing in Asymptomatic Patients without Known CAD
NOTE: The class IIb indication evokes controversy because of the increased risk of false-positive results. However, with good clinical judgment or consultation of a table (e.g., Fig. 93-4, Table 93-1), the clinician may determine that the patient has a pretest likelihood as high as 15% to 20%, making the patient a reasonable candidate for EET.
Exercise ECG Testing for Valvular Heart Disease
Exercise ECG Testing before and after Revascularization
Exercise ECG Testing for Investigation of Heart Rhythm Disorders
Noninvasive Stress Testing before Noncardiac Surgery
Indications in the Emergency Department
Patient has or had chest pain and fulfills the following requirements:
Indications for Firefighters
Indications before Noncardiac Surgery
Half of serious complications related to noncardiac surgery are cardiovascular. Although older patients have the highest risk of a cardiovascular complication with surgery, they also make up the largest group of patients undergoing surgery. Consequently, as the population ages, the cardiovascular risk of surgery will increase. Guidelines are available for screening patients before noncardiac surgery, and the ACC/AHA guidelines have been studied from an outcomes perspective. Box 93-2 provides some shortcuts for determining need for noninvasive testing. Patients about to undergo low-risk surgery (<1% risk; e.g., endoscopic, superficial, cataract, breast, ambulatory surgery) or with at least a fair functional/exercise capacity do not need further testing. If the patient has undergone revascularization (PCI or CABG) within the past 5 years or a thorough evaluation of the coronary arteries (e.g., EET, stress imaging) within the past 2 years, and there has not been a change in symptoms suggestive of ischemia, then according to ACC/AHA guidelines no further testing is necessary. Conversely, if high-risk (often >5% risk of serious complications) vascular surgery is planned and the patient has three or more clinical risk factors (i.e., CAD, CHF, ASCVD, diabetes, or renal insufficiency [creatinine ≥2 mg/dL]), noninvasive testing is reasonable if the results will change the management. It should be kept in mind that the goal of perioperative cardiac assessment is to detect the patient who would benefit from revascularization anyway, not just to get him or her through surgery. (See also Chapter 230, Preoperative Evaluation.)
Box 93-2 Shortcuts to Determine Indicators for Noninvasive Testing before Noncardiac Surgery
From Fleisher LA, Beckman JA, Brown KA, et al: ACC/AHA 2007 guidelines on perioperative cardiovascular evaluation and care for noncardiac surgery: Executive summary. A report of the American College of Cardiology/American Heart Association Task Force on Practice Guidelines (Writing Committee to Revise the 2002 Guidelines on Perioperative Cardiovascular Evaluation for Noncardiac Surgery). Circulation 116:1971–1996, 2007.
No testing necessary if “Yes” to any of these four questions:
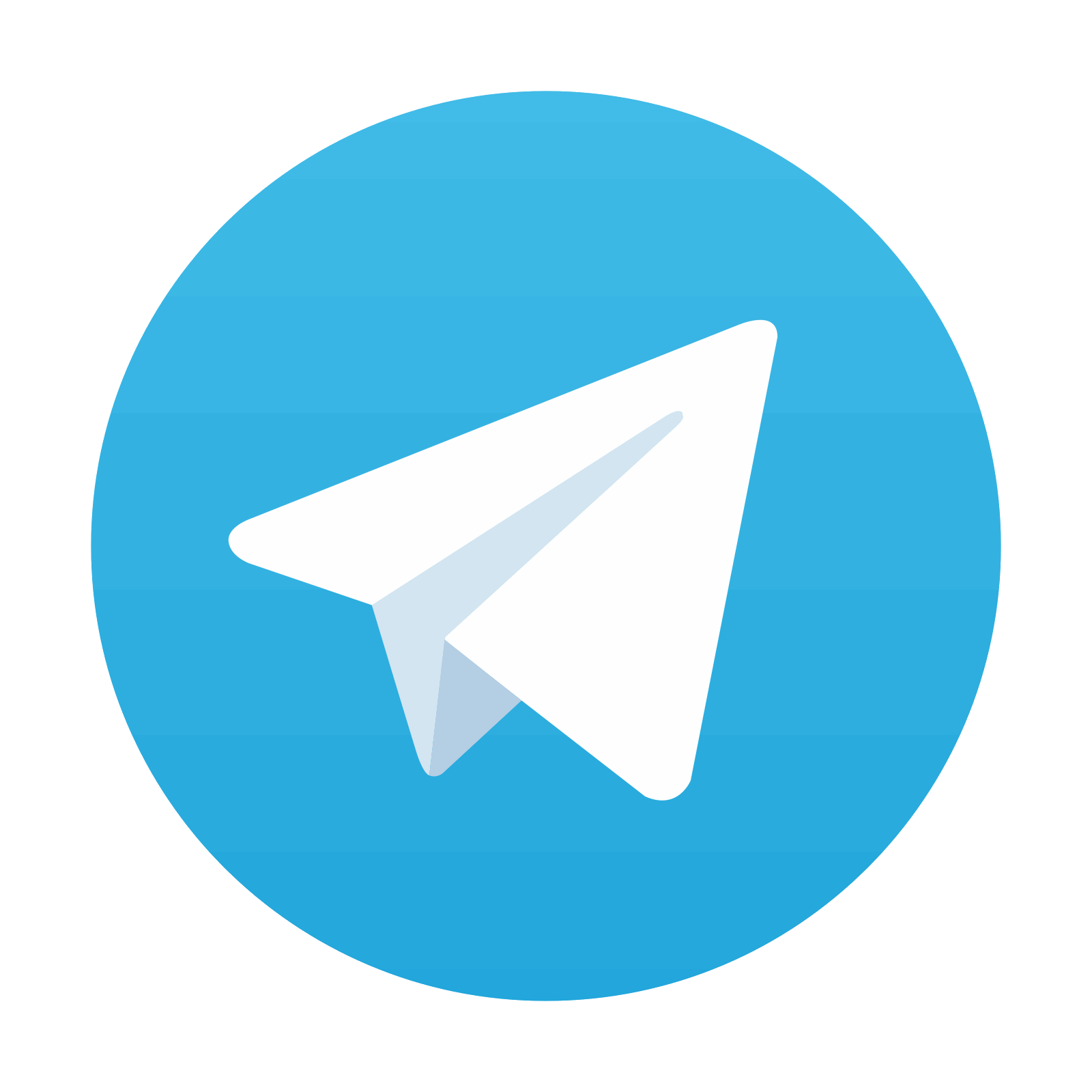
Stay updated, free articles. Join our Telegram channel

Full access? Get Clinical Tree
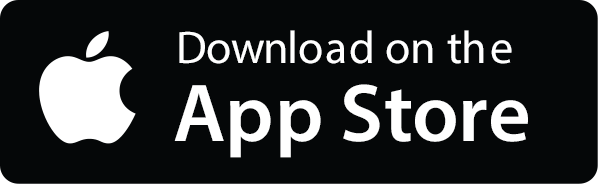
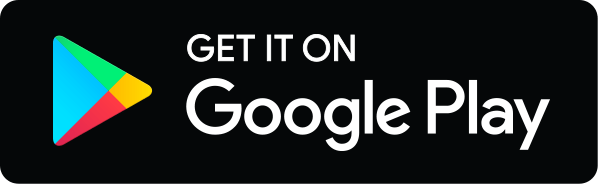