Ewing’s Sarcoma and Related Tumors
In the early 1920s, James Ewing identified a peculiar round-cell tumor that he called diffuse endothelioma of bone.1 The tumor predominantly affected the long tubular bones of young patients. This lesion was distinguished from the general category of unclassified round-cell sarcomas and lymphohematopoietic tumors based on its clinicopathologic features (i.e., small, round-cell morphology), a predilection for the long tubular bones of skeletally immature patients, and the absence of a lymphoma-like or leukemia-like clinical picture.1,2 Although many of the original pathogenetic theories proposed by Ewing and other early investigators have been significantly modified, Ewing’s sarcoma remains recognized as a distinct entity and represents a prototype of nonhematologic round-cell malignancy.10 The delineation of this entity also serves as an example of the brilliant correlations of clinical, radiographic, and microscopic findings that set us on a quest to understand the biology of this unique tumor, providing the foundation for its diagnosis and treatment. Several additional lesions with clinicopathologic features different from classical Ewing’s sarcoma have subsequently been described in the soft tissue and specific anatomic regions; these are referred to as Askin’s tumor and primitive neuroectodermal tumor (PNET). Tumors with microscopic features similar to classical Ewing’s sarcoma or PNET have also been described in parenchymal and reproductive organs. The consistent presence of a chromosomal abnormality—the reciprocal translocation of chromosomes 11 and 22, which involves bands q24 and q12 of the chromosomes, respectively—is a unique finding in this group of tumors.6,64,65 This finding is essential in understanding these malignancies, collectively referred to as the Ewing’s sarcoma/PNET family of tumors. During the past three decades, molecular studies have elucidated the structure of the chromosomal breakpoint and have identified the fusion partners of the chimeric gene. Subsequent investigations and recent genome-based sequencing studies have expanded the list of chromosomal translocations and their specific chimeric genes to over 15 distinct translocations, many of which have multiple variants (Fig. 11-1). This data has expanded our current understanding of the complex biology of these tumors and is the basis of an emerging novel classification of Ewing’s sarcoma and related tumors. There is also hope that the evolving molecular data can guide us to more effective targeted therapies for these tumors. From a diagnostic point of view, Ewing’s sarcoma and related tumors serve as a prototype for a modern diagnostic approach in which clinical, radiographic, microscopic, and molecular data contribute to the diagnosis. The emergence of molecular techniques as a significant aid in the diagnosis of this group of malignancies should be particularly emphasized.
In this chapter conventional descriptions of Ewing’s sarcoma, PNET, and Askin’s tumors are retained, but the reader should understand that in view of modern molecular data, these tumors are considered as variants of the same entity. Moreover, recent genomic data has expanded this group of tumors exponentially by the inclusion of tumors deviating somewhat from the classical Ewing’s sarcoma morphology; these are referred to as Ewing’s sarcoma-like and are characterized by chromosomal translocations and gene fusions differing from those originally identified. The description of molecular features of this group of tumors is focused on the nature of translocations and their genes as potential biomarkers in classification and differential diagnosis. The interested reader is referred to Chapter 3, where the biologic effects of signature hybrid proteins in this group of tumors are described.
Chromosomal Translocations
Breaks in chromosomes may result in the abnormal assembly of genetic material, resulting in the transfer of one fragment of a chromosome to another. These, in turn, can fuse together the coding sequences of genes that, in normal genomes, function as separate independent coding units.21 Such fusions create abnormal hybrid mRNAs that contain coding sequences of both genes and result in the expression of a new protein, which can have profound effects on cell biology, contributing to malignant transformation.32
The unifying feature of the group of tumors described in this chapter is the presence of distinct chromosomal translocations that are clonal and result in the expression of new proteins that do not exist in normal cells. These translocations drive the biology of tumors, are diagnostically specific, and may represent future therapeutic targets. In fact, the Ewing’s sarcoma/PNET family of tumors carry a unique abnormality, t(11;22)(q24;q12), resulting in the expression of a chimeric protein, EWSR1-FLI1, which represents a prototypic chromosomal abnormality in soft tissue and bone tumors. In addition to this prototypic translocation found in the majority of Ewing’s sarcoma family of tumors, over 15 distinct chromosomal translocations and hybrid genes have been identified in this group of tumors, providing new unifying concepts for their diagnosis and treatment.39,52
Prototypic Chromosomal Translocations Involving EWSR1 and ETS Partners
Multiple chromosomal translocations resulting in hybrid genes identified in the Ewing’s family of tumors can be divided into two main groups: those containing hybrid genes with fusion partners that belong to the E-26 transformation specific (ETS) transcription factors and those that contain hybrid genes with fusion partners that do not belong to this family, referred to as non-ETS partners.12,41 The ETS family represents one of the largest groups of transcription factors, which are evolutionary highly conserved and play important roles in cell development and can have major transforming effects contributing to tumor development.26 There are 29 members in this group in humans, with FLI1 and ERG representing the most frequently involved ETS members in the Ewing’s sarcoma/PNET family of tumors.
Translocation t(11;22)(q24;q12) with EWSR1-FLI1 Chimeric Gene
Ewing’s sarcoma and related tumors exhibit a unique karyotypic abnormality, t(11;22)(q24;q12), that results in the expression of the chimeric protein EWSR1-FLI1(Fig. 11-2).13,14,17,58,64 This genetic abnormality occurs in nearly 90% of Ewing’s sarcoma family tumors.22,51,65,67 The reciprocal translocation of portions of chromosomes 11 and 22, involving bands (q24;q12), in Ewing’s sarcoma/PNET represents a prototypic chromosomal abnormality in soft tissue and bone tumors.
An initial molecular analysis showed that the chromosome 22 breakpoint is located within the EWSR1 gene, whereas the chromosome 11 breakpoint is located within the FLI1 gene.9,38,69,70 The reciprocal translocation between these two chromosomes creates a new chimeric protein, consisting of portions of the EWSR1 and FLI1 proteins.70 The EWSR1 gene located within 22q12 plays a recurrent major role in several chromosomal translocations in soft tissue and bone tumors. It is involved in all the alternative translocations identified in Ewing’s sarcoma/PNET, as well as in distinct translocations in desmoplastic small round-cell tumor, clear cell sarcoma (melanoma of soft parts), some cases of extraskeletal myxoid chondrosarcoma, angiomatoid fibrous histiocytoma, myxoid liposarcoma, and myoepithelial tumors of soft tissue and bone (Table 11-1).22,34,41,63,68 The EWSR1 gene has 17 exons and spans approximately 40 kb.45 It encodes a protein with homology to a putative RNA-binding site of RNA polymerase II. Nearly 80% of the breakpoints found in Ewing’s sarcoma/PNET are located within introns 7 and 8.31 A breakpoint in these introns results in a chimeric transcript that includes exons 1 through 7 of the EWSR1 gene. Regardless of whether the breakpoint is within intron 7 or 8, the exon 8 sequence is spliced out of the chimeric transcript. The second component in the most common fusion variant is the human homolog of the murine Fli1 gene located on chromosome 11q24.38 The murine Fli1 gene contains a retrovirus integration site for the Friend murine leukemia virus and is rearranged in erythroleukemias induced by this virus.46 Both human and murine variants of the FLI1 gene belong to a family of DNA-binding proteins.8 The human FLI1 protein shares approximately 70% sequence homology with the protein encoded by the ETS1 gene (which is expressed during cranial neural crest migration and possibly during vasculogenesis) and seems to function primarily as a transcription factor.7,13,14 In the chimeric protein, the RNA-binding domain of the protein encoded by the EWSR1 gene is substituted for the DNA-binding domain of the FLI1 gene product. The chimeric EWSR1-FLI1 gene is transcribed under control of the EWSR1 promoter.9 Expression of both of these individual genes is virtually ubiquitous in normal human tissues. The molecular downstream targets for chimeric proteins are still poorly understood.4 It has been reported that the cyclin E, protein tyrosine phosphatase (PTLP1), cyclin-dependent kinase inhibitor 1A (p21/WAF1/CIP1), and the LAMB3 gene encoding the β3 chain basement membrane laminin 5 are molecular targets for EWSR1-ETS fusion proteins.3,24,33,40 The expression of the EWSR1-FLI1 fusion protein upregulates genes associated with neural crest development and is responsible for the phenotype of Ewing’s sarcoma/PNET family of tumors.23,56 In general, the expression signature of these tumors is characterized by upregulation of the genes that are the targets of the ETS family of transcription factors.
This and other translocations in Ewing’s sarcoma and related tumors can be detected by a variety of cytogenetic and molecular techniques.5,18,37,51,55 In typical cases, conventional cytogenetics identifies the reciprocal rearrangements between chromosomes 11 and 22 characteristic of Ewing’s sarcoma. However, when such a rearrangement occurs in the background of complex chromosomal abnormalities, its conventional cytogenetic identification may be problematic. In such instances, spectral karyotyping (SKY) is useful to demonstrate the specific genetic abnormality (Fig. 11-3). Other techniques that are commonly used to identify the translocations identify the presence of breakpoints within the gene using fluorescence in situ hybridization (FISH) with the so-called break-apart or fusion probes. Both cytogenetic and FISH techniques can be complemented by reverse transcription polymerase chain reaction (RT-PCR) and DNA sequencing, which identify the specific fusion transcripts.11,43 More detailed description of these techniques is provided in Chapter 3.
Translocation t(21;22)(q22;q12) with EWSR1-ERG Chimeric Gene
It has been shown that EWSR1 may be translocated to other locations and can form chimeric genes with alternative partners (Table 11-2). A second alternative cytogenetic abnormality, t(21;22)(q22;q12), is present in approximately 10% of Ewing’s sarcomas and PNETs (Fig. 11-4). This translocation fuses the portion of the EWSR1 gene from 22q12 to the ERG gene located on 21q22.19,54 In some instances, complex multistep rearrangements of chromosomes 19, 21, and 22 are involved in a reciprocal inversion-insertion mechanism that results in the EWS1-ERG fusion.19,36 The ERG gene encodes a protein that belongs to the ETS family of transcription factors, which, in cell physiology, regulates embryonic development, cell proliferation, differentiation, angiogenesis, inflammation, and apoptosis. The major source of lethal effects of loss of function of ERG in mutagenesis animal experiments is related to hematopoietic stem cell defects. In addition to Ewing’s sarcoma, this gene is a common fusion partner of hybrid genes in prostate cancer such as TMPRSS2-ERG and NDRG1-ERG. Its biologic effects and potential contribution to Ewing’s sarcoma depends on its similarity to FLI1. ERG and FLI1 have similar structures and are likely to have similar biologic effects in chimeric fusions with EWSR1. ERG shares 68% of the amino acid sequence identity with FLI1. Moreover, the ETS common domain required for sequence specific binding to DNA shows 98% identity with FLI1. The formation of the EWSR1-ERG chimeric gene develops in the absence of the most frequent EWSR1-FLI1 fusion and is likely to be able to replace EWSR1-FLI1 in the oncogenic pathway contributing to the development of Ewing’s sarcoma. Considering the similarities between these two fusion partners, it is very likely that both chimeras use similar mechanisms to orchestrate an overlapping repertoire of target genes.
Translocations with Alternative ETS Fusion Partners
Several additional translocations with alternative ETS fusion partners have been identified in the Ewing’s sarcoma/PNET family of tumors. Three of them involve EWSR1 combined with ETV1, ETV4, or FEV: t(7;22)(p22;q12) EWRS1-ETV1, t(17:22)(q12;q12) EWRS1-ETV4, and t(2;22)(q33;q12) EWSR1-FEV.16,27,49,66 In addition, two translocations of ERG or FEV with FUS were identified: t(16;21)(p11;q22) FUS-ERG and t(2;16)(q35;p11) FUS-FEV.16,194 All of these genes belong to the ETS family of transcription factors and contain the ETS domain required for sequence specific binding to DNA in the regulatory elements of the target genes. Overall, they have high sequence homology and are nearly identical in terms of the ETS sequence domain (Fig. 11-7). Biologically, they represent functional substitutes for the two main EWSR1 fusion partners, FLI1 and ERG, described previously in this chapter.29 These fusions develop in the absence of the common translocations such as EWSR1-FLI1 and EWSR1-ERG. They appear to functionally replace these more common translocations in oncogenic transformation.
Translocations with Non-ETS Fusion Partners
Multiple translocations with fusion partners that do not belong to the ETS family of transcriptional factors have been identified in Ewing’s sarcoma/PNET family of tumors (Fig. 11-7). They can be divided into two main groups: those that involve EWSR1 as the 5′ partner fused to a non-ETS 3′ partner and those that involve a completely new 5′ partner such as CIC or BCOR combined with a non-ETS 3′ partner. The first group comprises the following translocations: t(6;22)(p21;q12) EWRS1-POU5F1, Inv(22)(q12) EWSR1-ZSG(ZNF278), t(4;22)(q31;q12) EWSR1-SMARCA, t(2,22)(q31;q12) EWSR1-SP3, t(1;22)(p36.1;q12) EWSR1-ZNF278, ring chromosome with amplification and translocations resulting in EWSR1-NFATC2.15,50,60,61,66 The second group of tumors characterized by involvement of non-ETS genes fused to non-EWSR1 5′ partners include: t(4;19)(q35;q13) CIC-DUX4 and an inversion involving Xp11.4; Xp11.22 resulting in BCOR-CCNB3.25,30,44,47,53,57 Preliminary data implicates small cell sarcomas of bone and soft tissue characterized by these abnormalities that have a different gene expression profile from the group of Ewing’s sarcomas containing fusions with ETS partners.57
The 3′ fusion partners in this group of Ewing’s/PNET sarcomas represent a diverse group of transcription factors with distinct DNA sequence binding domains. The POU5F1 gene contains a POU homeodomain that plays a key role in embryonic development and stem cell pluripotency. The ZSG gene encodes an A-T hook DNA binding motif, which plays an important role in chromatin remodeling and transcriptional regulation. It also contains the Poz domain, which functions as a site of protein-to-protein interactions and transcriptional repression, as well as the zinc-fingers DNA binding domain. The SMARCA1 gene encodes a member of the SWI/SNF family of proteins with helicase and ATPase activities that regulate chromatin structure around the target genes. The SP3 gene encodes a protein that regulates transcription by binding to a consensus GC- and GT-box regulatory element in target genes. It contains several other DNA binding domains, including a zinc finger. The ZNF278 gene encodes a protein that contains several transcriptional factor domains, including an A-T hook DNA binding motif that plays an important role in chromatin remodeling and transcription regulation. The NFATC2 gene is a major transcription factor of activated T cells and has several DNA binding domains with REL- and NFAT-homology regions.
The two recently identified fusion genes, CIC-DUX4 and BCOR-CCNB3, are unique because each represents a fusion of two genes with distinct transcription factor activities (Figs 11-5 and 11-6).25,30,44,47,53,57 The 5′ partner of the first fusion, the CIC gene, is a human homolog of a high mobility group (HMG) box transcription factor that has been shown to mediate c-ErbB signaling. The 3′ partner in this fusion is the DUX4 gene, which encodes to two homeoboxes, the repeat-array and ORF. The protein functions as a transcriptional activator. In the second fusion, the 5′ partner is BCOR, encoding the BCL6 corepressor and the 3′ partner, CCNB3, is encoding the testis-specific cyclin B3 and plays an essential role in the control of cell cycle. The group of Ewing’s sarcoma-like tumors is consistently expanding; a new CIC-FOXO4 gene fusion has been recently identified.59 Moreover, new cytogenetic abnormalities continue to be identified, as well as complex chromosomal abnormalities with still uncertain clinical significance.28,42,48
Preliminary data indicate that the diversity of molecular abnormalities in Ewing’s sarcoma and related lesions has an impact on their biologic behavior and may represent clinically useful prognostic factors.4,11,20,42,68,70
Unified Pathogenetic Concept of Ewing’s Sarcoma and Related Tumors
When t(11;22)(q24;q12) was cytogenetically identified, it represented, until the elucidation of the molecular structure of the breakpoint, the only specific finding in Ewing’s sarcoma. However, the tumors were still mostly classified as Ewing’s sarcoma on the basis of their small cell morphology and clinicoradiologic features (i.e., a predilection for long tubular bones of young individuals and the absence of a lymphoma-like or leukemia-like picture clinically). Therefore several lesions with clinicopathologic features distinct from those of classic Ewing’s sarcoma, such as the presence of neural differentiation microscopically or a predilection for the thoracopulmonary region as in Askin’s tumor, were not originally linked to the Ewing’s sarcoma family of tumors.71,85,89,96–98 Subsequent investigations showed that t(11;12)(q24;q12) was a consistent cytogenetic abnormality in these lesions; this contributed to the current understanding of these conditions as variants of Ewing’s sarcoma62,77,85,87,92,93 (Fig. 11-8). Although our current understanding is that these tumors are related pathogenetic entities, there is still a clinical tendency to analyze them separately.72,94 Further investigations revealed that the same cytogenetic abnormality can be identified in at least some of the tumors classified as small cell osteosarcomas.76,78,90 Most likely some of the tumors diagnosed in the past as small cell osteosarcomas would be classified today as variants of Ewing’s sarcoma.73,79,88 The production of tumor bone in a lesion with microscopic, immunohistochemical, and molecular features of Ewing’s sarcoma should be considered as divergent differentiation rather than a feature of a distinct pathologic entity. This is not, however, to deny the existence of small cell osteosarcoma distinct from Ewing’s sarcoma.
The concept that Ewing’s sarcoma originates from undifferentiated mesenchymal cells with a propensity for neural crest lineage differentiation opens the possibility that, occasionally, pathways other than neural differentiation can occur in a tumor with basic small round-cell morphologic features.
Finally, the concept of pluripotential differentiation in tumors with small round-cell components similar to those of Ewing’s sarcoma, such as seen in extremely rare examples of lesions designated as primitive pluripotential sarcoma, polyhistioma, or ectomesenchymoma, has been postulated.80,82,83,91,99 Review of a large series of small cell tumors disclosed the presence of rare cases of ill-defined primitive sarcomas.127 Neural, muscular, and epithelial differentiation was documented immunohistochemically in some of these lesions and was confirmed by ultrastructural studies. It seems that the tendency for pluripotential differentiation is more often seen in lesions involving the thoracopulmonary region. Moreover, the presence of MIC2 gene expression confined to primitive round-cell areas has been shown in some of these lesions.99 The presence of an EWSR1-FLI1 fusion gene has been identified in biphenotypic sarcoma with divergent myogenic/neural differentiation and in tumors exhibiting adamantinoma-like cytoarchitecture, providing objective evidence that at least some of these lesions may have a common pathogenesis with the Ewing’s sarcoma group.74,75,81,84,86,95,100
The identification of multiple variants of reciprocal translocations with the EWSR1 gene and other fusion partners belonging to the ETS and non-ETS transcriptional factor families as well as translocations with gene fusions in which the 5′ partners are different than ESWR1, such as FUS, CIC, and BCOR, is the foundation of novel emerging molecular classifications of this family of tumors (Fig. 11-8). It appears that using this information, the Ewing’s sarcoma/PNET family and related tumors can be divided into the following groups: (1) classical Ewing’s sarcoma/PNET group with most frequent fusion genes (EWSR1-FLI1 and EWSR1-ERG), (2) classical Ewing’s sarcoma/PNET group with alternative 3′ ETS fusion partners (EWSR1-EVT1, EWSR1-EVT4, EWSR1-FEV, FUS-ERG, and FUS-FEV), and (3) Ewing’s sarcoma-like tumors with non-ETS 3′ fusion partners (EWSR1-POU5F1, EWSR1-ZNF287, EWSR1-SMARCA, EWSR1-SP3, EWSR1-NFATC2 , CIC-DUX4, and BCOR-CCNB3). The emerging data suggests that all fusions with the ETS members are functionally similar and that tumors with such characteristics form a uniform group with similar gene expression profiles. On the other hand, the group containing fusions with non-ETS partners is heterogeneous due to the involvement of transcriptional factors belonging to different families and to targeting different sets of genes. Such evidence is available for at least some of the distinct fusion genes, such as CIC-DUX4 and BCOR-CCNB3.47,57 Most interestingly, these tumors appear to have microscopic features somewhat different from those of classical Ewing’s sarcoma/PNET, with a tendency to fall into the atypical category, and may be clinically distinct, exhibiting a propensity for lymph node metastasis rather than the lung metastasis characteristic of classical Ewing’s sarcoma/PNET.47,57
Ewing’s Sarcoma
Definition
Ewing’s sarcoma is a distinct, round-cell sarcoma of bone that occurs predominantly in the long bones of skeletally immature patients. The tumor is composed of undifferentiated, round, mesenchymal cells that are rich in glycogen and exhibit a karyotypic abnormality representing reciprocal t(11;22)(q24;q12) and resulting in the expression of a chimeric EWSR1-FLI1 protein. This genetic abnormality can be documented in approximately 90% of tumors morphologically classified as Ewing’s sarcomas. In a small proportion of Ewing’s sarcomas, alternative chromosomal translocations involving EWSR1 and other genes can occur. In general, the expanded family of Ewing’s sarcoma tumors can be separated into two major groups containing chimeric genes with ETS and non-ETS fusion partners.
Incidence and Location
The peak age incidence and frequent skeletal sites of involvement are shown in Figure 11-9. Ewing’s sarcoma accounts for 6% to 8% of all primary malignant tumors of bone. It is the most frequently occurring primary sarcoma of bone after osteosarcoma and chondrosarcoma. It has a peculiar predilection for whites and practically never occurs in blacks. In the 1973 to 2010 Surveillance, Epidemiology, and End Results (SEER) program series, only approximately 3.5% of Ewing’s sarcoma cases occurred in black patients. This striking difference in the incidence of Ewing’s sarcoma, which shows a tenfold higher incidence in the white population compared to the black population, is potentially of major pathogenetic significance and may provide important clues to the genetic predisposition for this unusual malignancy.114 The peak incidence is during the second decade of life, and there is a male predilection (male-to-female ratio, 1.3 : 1 to 1.4 : 1) (Figs. 11-10 and 11-11). Approximately 80% of all patients are younger than age 20 years, and 50% are affected in the second decade of life. The remaining cases are diagnosed in patients between ages 20 and 30 years. Ewing’s sarcoma is extremely rare in patients older than age 30 years. When confronted with the possibility of Ewing’s sarcoma in patients older than age 30 years, the clinician must first eliminate small cell carcinoma and large cell lymphoma from the differential diagnosis. In very young patients, the possibility of metastatic neuroblastoma or acute leukemia should also be ruled out. These entities are traditionally listed as potential differential diagnostic problems with Ewing’s sarcoma and other round-cell malignancies. With the advent of molecular probes and immunohistochemical techniques, differentiation among these tumors rarely presents a diagnostic problem. Ewing’s sarcoma has a tendency to involve the shafts of long tubular bones, the pelvis, and ribs, but practically every bone can be affected, including the acral skeleton, craniofacial bones, and the clavicle (Fig. 11-12).
Radiographic Imaging
Typically Ewing’s sarcoma presents as an ill-defined, permeative or focally moth-eaten, destructive intramedullary lesion that affects the diaphysis of a major long tubular bone. The presence of a large, ill-defined density in soft tissue in addition to the permeative intramedullary lesion is a frequent feature (Figs. 11-13 to 11-18). The lesion is often accompanied by a prominent multilayered periosteal reaction (onion skin) (Figs. 11-13 and 11-15). The perpendicular “sunburst” type of periosteal new bone formation can be present but is less common compared with its occurrence in osteosarcoma. The cortex overlying the lesion can exhibit diffuse sclerosis and thickening (Fig. 11-19). The radiographic features are nonspecific, but with the clinical features, the diagnosis of Ewing’s sarcoma is correctly suspected in the majority of cases. The lesion involves the medullary cavity and soft tissue more extensively than is usually documented on plain radiographs. Magnetic resonance imaging and computed tomography permit more accurate preoperative assessment of the lesion than plain radiographs. They typically demonstrate intramedullary lesions that involve large segments of the intramedullary cavity and extend beyond the area shown to be involved on plain radiographs. Ewing’s sarcoma can produce a concave defect on the bone surface that is referred to as saucerization (Figs. 11-18 and 11-20). This type of radiographic appearance is typically seen in predominantly subperiosteal lesions. More often, saucerization is seen in intramedullary lesions in which the cortex is permeated and a secondary subperiosteal and soft tissue mass that compresses the outer surface of the cortex is formed (Fig. 11-18). The majority of Ewing’s sarcomas are purely lytic lesions (Figs. 11-20 to 11-24). Occasionally, Ewing’s sarcoma can induce a prominent diffuse thickening of the preexisting bone and may present radiographically as a sclerotic lesion, or it may contain focal cloudy opacities. The sclerotic features or focal opacities are more often seen in lesions involving the metaphyseal portions of long bones or in flat bones and are practically never seen in diaphyseal lesions. On the other hand, diaphyseal lesions of long bones are often associated with diffuse sclerosis and thickening of the overlying cortex. Presentation at an advanced stage with a large mass is a recurring theme for patients with Ewing’s sarcoma (Figs. 11-25 and 11-26).
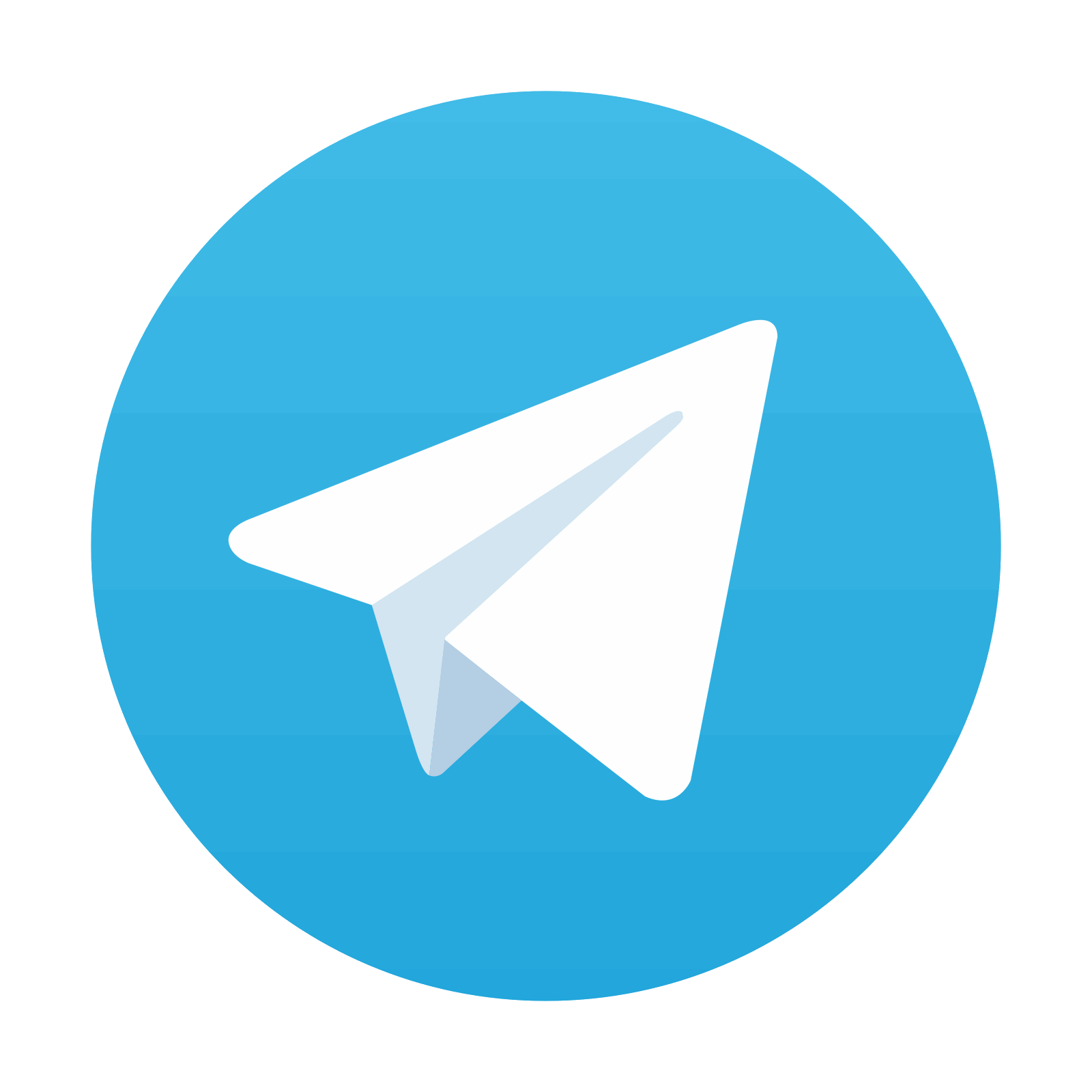
Stay updated, free articles. Join our Telegram channel

Full access? Get Clinical Tree
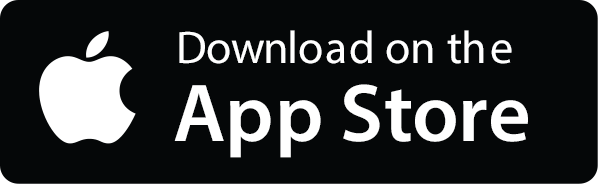
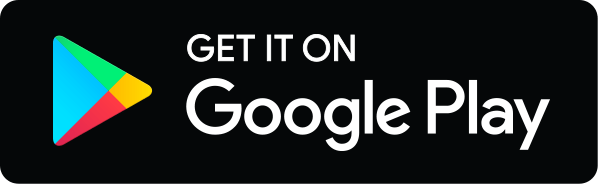