2
Essential oil composition
Essential oils
Aromatic plants and infusions prepared from them have been employed in medicines and cosmetics for many thousands of years, but the use of distilled oils dates back only to the 10th century, when distillation as we know it today was developed (Forbes 1970). Solvent-extracted materials such as absolutes, resinoids and CO2 extracts are much more recent inventions. Plants that produce essential oils belong to many different botanical species and are found throughout the globe. It is estimated that there are 350,000 plant species globally, and that 5% of these (17,500 species) are aromatic (Lawrence 1995g, pp. 187–188). Of these, more than 400 are commercially processed for their aromatic raw materials, about 50% being cultivated, and the rest being obtained either as by-products of a primary industry or harvested in the wild. The principal ten essential oil-bearing plant families are listed in Box 2.1.
Isolation
Although most constituents remain intact during distillation, a few undergo chemical changes. Chamazulene, for example, is not a natural product, but is formed by decomposition of its precursor, matricin, during steam distillation of blue chamomile oil. Garlic oil also contains substances that are formed from reactive precursors on distillation (Lawson et al 1992). Esters, such as linalyl acetate, may partially hydrolyze to alcohols during distillation. In other cases, undesirable ‘artifacts’ are formed during distillation, which are then removed during ‘rectification’ usually by fractional distillation, a process using a tall column that separates out single constituents or mixtures of compounds with similar boiling points. In some cases these constituents are removed because of their toxicity, such as the hydrocyanic acid in bitter almond oil, or the polynuclear hydrocarbons in cade oil. In the case of deterpenated oils, terpenes are removed in order to create an oil with unusual flavor and fragrance qualities.
Composition
Seasonal variations, for example, can be seen in the 1,8-cineole content of Moroccan Eucalyptus globulus oil, which has a low of 62.4% in May and a high of 82.2% in July (Zrira & Benjilali 1996). Great variations in the menthone content of French Mentha x piperita oil can be seen, with lows of 6.1–8.1% in October and highs of 48.8–54.5% in June (Chalchat et al 1997). The α-thujone content of an Italian Salvia officinalis oil ranges from 29.7% in April to 48.8% in October (Piccaglia et al 1997). Elevation can also affect composition. In similar thyme plants grown in Turkey at elevations of 18 m and 1,200 m, oils extracted at full-flowering over three years showed average p-cymol contents of 39.5% (lowland) and 28.3% (mountainous) (Özgüven & Tansi 1998).
Chemotypes
The majority of thyme oils on the market are those rich in thymol and/or carvacrol, compounds with strong antibacterial properties, but which are also moderately irritant. The toxicity of some essential oils depends greatly on chemotype, notably basil, buchu, ho leaf and hyssop. Chemotypes are variants within a single botanical species, but in other cases, such as calamus or sage, a difference in botanical origin also entails significant compositional differences with important toxicological consequences. Clear labeling, with chemotype and botanical species, can therefore be of great importance in distinguishing between apparently similar essential oils.
Contamination
Contaminants are substances that are not natural constituents, artifacts of distillation, or adulterants (adulteration being intentional dilution or fabrication). They can include plasticizers and pesticides, or traces of solvent in solvent-extracted products. Because of their antimicrobial properties, essential oils are not generally subject to microbial contamination. Maudsley & Kerr (1999), for example, reported that eight essential oils were sterile and did not support the growth of seven bacterial species and Candida albicans.
Biocides
There are over 400 chemical biocides (pesticides or herbicides) that might be used on aromatic plants, and many of these do carry over during steam distillation (Briggs & McLaughlin 1974; Belanger 1989; Dikshith et al 1989). The products of solvent extraction (absolutes, resinoids and CO2 extracts) are even more likely to retain any biocides, as are cold-pressed citrus oils. The transfer of the insecticide chlorpyrifos from roses to rose water, rose concrete and rose absolute, was 5.7%, 46.9% and 38.8%, respectively. Rose otto was not tested. Twelve days following application, no traces of chlorpyrifos were detectable in flowers or leaves (Kumar et al 2004).
Of 11 organochlorine pesticides screened in 148 cold-pressed lemon oils, 123 sweet orange oils, 121 mandarin oils and 147 bergamot oils produced in Italy in the years 1991–1996 (~20 samples per oil, per year) two pesticides (tetradifon and difocol) were detected, in addition to 4,4’-dichlorobenzophenone, a decomposition product of difocol. The detected range for a single pesticide was 0–5.95 ppm (Saitta et al 2000). A steady decline in the percentage of contaminated samples was seen over the six year period (Table 2.1). Dugo et al (1997) reviewed the organophosphorus and organochlorine pesticides in Italian citrus oils. They reported that since the 1960s, concentrations of pesticide ranging between 1.5 ppm and 450 ppm had been detected. Between 1983 and 1991, 12 organophosphorus pesticide residues were detected in 33 lemon oils, with total pesticide content ranging from 4.95–49.3 ppm. Of the 33 oils, 97.4% contained methyl parathion, 99.3% ethyl parathion and 98.4% methidathion. Di Bella et al (2004) found 0.26 mg/L and 0.20 mg/L of difocol in Calabrian bergamot oils from 1999 and 2000, respectively. Tetradifon was found at 0.06 mg/L for both years. Certified organic citrus oils are becoming widely available.
Table 2.1
Decline in percentage of citrus oils containing pesticides over a six year period
Data from Saitta et al (2000).
Propiconazole and tebuconazole, fungicides used to control rust in peppermint, were detected in the essential oil at 0.02–0.05 mg/kg and 0.01–0.04 mg/kg, respectively (Garland et al 1999). There is evidence of reproductive, hepatic and immunotoxicity for propiconazole (Wolf et al 2006; Goetz et al 2007; Martin et al 2007). Other investigations have found the insecticides chlorpyrifos and carbofuran in peppermint oil (Inman et al 1981, 1983), but failed to find the nematocide oxamyl in the same oil (Kiigemagi et al 1984). Chlorpyrifos exposure has been associated with low testosterone levels in US adult males, and with CNS (central nervous system) toxicity after neonatal exposure (Aldridge et al 2005; Meeker et al 2006). Overexposure to carbofuran has resulted in acute poisoning in both Nicaragua and Senegal, including some fatalities (McConnell & Hruska 1993; Gomes do Espirito Santo et al 2002).
Biocide use might feasibly alter essential oil composition. In three similar reports, the composition of sage oil and melissa oil was not significantly altered by the use of the pesticide afalon WP50, although only those chemicals expected to occur naturally in the oil were studied (Vaverkova et al 1995a, 1995b; Tekel et al 1997). However, in another study the use of metribuzin increased the linalool content of coriander seed oil (Zheljazkov & Zhalnov 1995).3
Sometimes the origin of xenobiotic substances found in essential oils is open to debate. One study found 2,4,6-trichloroanisole in Mexican lime, French orange leaf, Spanish rue and Bulgarian rue oils, but concluded that it was probably of microbial rather than pesticidal origin (Stoffelsma & De Roos 1973).
According to Hotchkiss (1994) most biocides are poorly absorbed through the skin, though chlorpyrifos and carbofuran are absorbed to a degree (Liu & Kim 2003; Meuling et al 2005). In vitro testing of human skin suggests that absorption depends on the solubility and molecular weight of the substance in question. Methiocarb, for instance, is relatively well absorbed, but dimethoate is not absorbed at all (Nielsen et al 2004). Dermal exposure to methyl parathion in rats resulted in acute toxic effects at 50 mg/kg, and only minimal toxicity at 6.25 or 12.5 mg/kg (Zhu et al 2001). These are very much higher doses than might be encountered from essential oil exposure.
It is feasible that an essential oil might enhance the dermal absorption of a biocide, and exposure through inhalation is also possible. The potential toxicity from biocides in essential oils is minimal, but still contributes to the total xenobiotic load, especially if biocides are also being ingested in foods, and zero exposure is surely preferable. Some of the reported allergic reactions to essential oils may be caused or enhanced by biocide residues, and not by the oils themselves (Wabner 1993).
Solvents
The use of benzene as a solvent for the extraction of concretes has declined considerably, but it is still in use. This well-documented carcinogen is listed under substances “known to be human carcinogens” by the NTP (National Toxicology Program 2005) and it is prohibited as a cosmetic ingredient in the European Union (EU) (Anon 2003a). The International Fragrance Association (IFRA) recommends that the level of benzene should be kept as low as practicable, and should not exceed 1 ppm in fragrance products (IFRA 2009). Traces of benzene may be present in absolutes processed from concretes extracted with it.
Cyclohexane is commonly used today as a replacement for benzene. Cyclohexane is made either by catalytic hydrogenation of benzene or by fractional distillation of petroleum, and may itself contain traces of benzene. Inhalation exposure of cyclohexane in rats indicates a NOAEL (no observed adverse effect level) of 500 ppm for both subchronic and reproductive toxicity (Kreckmann et al 2000; Malley et al 2000). Cyclohexane has not been evaluated for carcinogenicity, but it is not genotoxic. In a 2001 report by the Scientific Committee on Toxicity, Ecotoxicity and the Environment (CSTEE 2001) of the EU, it is suggested that human NOAELs of either 250 ppm or 1,200 ppm cyclohexane in air can be extrapolated from experimental data. n-Hexane, which is also used as a solvent, is neurotoxic (Tahti et al 1997). However, neither cyclohexane nor n-hexane present any risk of toxicity in the trace amounts present in absolutes. Other distillation/extraction solvents include polyethylene glycols, fluorocarbons and chlorofluorocarbons (Burfield, private communication, 2003).
Phthalates
Although there has been some reduction in usage, total phthalate exposure may still be a problem. A study of 85 individuals in Germany found that 10 exceeded the tolerable daily intake value set by the EU for phthalates, and 26 exceeded the Environment Protection Agency’s daily reference dose (Koch et al 2003). As a group, phthalates tend to be hepatotoxic, cause damage to the gastrointestinal tract, and demonstrate varying degrees of reproductive toxicity and carcinogenicity. They are also thought to be hormone disruptors (National Toxicology Program 1995; Duty et al 2003; Shea 2003; Seo KW et al 2004).
Not all phthalates are regarded as toxic. Diethyl phthalate (DEP), which is intentionally added to raw materials such as essential oils or resinoids, is apparently used because of its good safety profile. A comprehensive review of DEP by the Research Institute for Fragrance Materials (RIFM) concluded that, at the level of dermal exposure from its use in fragrance (0.73 mg/kg/day), there was no significant risk to humans with regard to skin reactions, carcinogenicity, reproductive toxicity or estrogenic activity (Api 2001a). The SCCNFP (Scientific Committee on Cosmetic Products and Non-Food Products, the EU regulatory body for cosmetics) concluded: ‘the safety profile of diethyl phthalate supports its use in cosmetic products at current levels. At present the SCCNFP does not recommend any specific warnings or restrictions under the currently proposed conditions of use’ (SCCNFP 2001b, 2003c). However, this remains a controversial subject, and DEP is now widely avoided in the industry.
Phthalates other than DEP are found in essential oils. According to Naqvi & Mandal (1995) di-(2-ethylhexyl) phthalate (DEHP) has been a common adulterant of sandalwood oil. Of eight phthalates screened in Italian lemon, orange and mandarin oils in the years 1994–1996, one or both of two phthalates, diisobutyl phthalate (DIBP) and DEHP, were found in almost all samples, and dibutyl phthalate (DBP) was found in 8 of the total of 87 samples tested. Total phthalate concentrations up to 4 ppm were detected (Di Bella et al 1999). DBP, DIBP and DEHP were all detected in Calabrian bergamot oils from crop years 1999 and 2000, at concentrations ranging from 1.22–1.65 mg/L per phthalate. The phthalates are thought to derive from plastic materials used in the production process of citrus oils (Di Bella et al 2004).
Both DBP and DIBP are genotoxic in human mucosa and DBP is reproductively toxic in rats (Kleinsasser et al 2000, 2001; Zhang et al 2004). DEHP may cause reproductive and developmental toxicity, and is thought to be an endocrine disruptor (Gayathri et al 2004; Latini et al 2004). There is debate about whether it is a carcinogen in humans (Melnick 2001). Phthalate adulteration/contamination in essential oils is declining due to increasing legislation and awareness of phthalate toxicity. Diethyl maleate is not permitted as a cosmetic ingredient in the EU (Anon 2003a).
Adulteration
The purpose of adulteration is to increase profits by adding either odorous or non-odorous substances in order to dilute an essential oil or absolute. Odorous adulterants can include other essential oils, essential oil fractions or residues, synthetic aromachemicals similar to those found in the oil, or aromachemicals not found in the oil. Non-odorous adulterants, or ‘extenders’, include substances such as ethanol, mineral oil, isopropyl myristate, glycols, phthalates, and fixed oils such as rapeseed and cottonseed. Examples of adulteration are shown in Table 2.2.
Table 2.2
Adulterants of some commonly used essential oils
Essential oil | Examples of adulterants used | References |
Bergamot | Other citrus oils or their residues, rectified or acetylated ho oil, synthetic linalool, limonene or linalyl acetate | Burfield 2003; Kubeczka 2002 |
Grapefruit | Orange terpenes, purified limonene | Burfield 2003 |
Jasmine absolute | Indole, α-amyl cinnamic aldehyde, ylang-ylang fractions, artificial jasmine bases, synthetic jasmones, etc | Arctander 1960 |
Lavender | Lavandin oil, spike lavender oil, Spanish sage oil, white camphor oil fractions, rectified or acetylated ho oil, acetylated lavandin oil, synthetic linalool or linalyl acetate | Burfield 2003; Kubeczka 2002 |
Lemon | Natural or synthetic citral or limonene, orange terpenes, lemon terpenes or by-products | Burfield 2003; Kubeczka 2002 |
Lemongrass | Synthetic citral | Singhal et al 1997 |
Patchouli | Gurjun balsam oil, copaiba balsam oil, cedarwood oil, patchouli vetiver and camphor distillate residues, hercolyn D, vegetable oils | Burfield 2003; Kubeczka 2002 |
Peppermint | Cornmint oil | Kubeczka 2002 |
Pine | Turpentine oil, mixtures of terpenes such as α-pinene, camphene and limonene, and esters such as (−)-bornyl acetate and isobornyl acetate | Burfield 2003; Kubeczka 2002 |
Rose | Ethanol, 2-phenylethanol, fractions of geranium oil or rhodinol | Kubeczka 2002 |
Rosemary | Eucalyptus oil, white camphor oil, turpentine oil and fractions thereof | Kubeczka 2002 |
Sandalwood | Australian or East African sandalwood oils, sandalwood terpenes and fragrance chemicals, castor oil, coconut oil, polyethylene glycol, DEHP | See profile |
Ylang-ylang | Gurjun balsam oil, cananga oil, lower grades or tail fractions of ylang-ylang oil, reconstituted oils, synthetics such as benzyl acetate, benzyl benzoate, benzyl cinnamate, methyl benzoate, benzyl benzoate and p-cresyl methyl ether. | Burfield 2003; Kubeczka 2002 |
Impurities in the synthetic chemicals used will also be present in amounts often ranging from 1–5%. For example, the presence of phenyl pentadienal, benzyl alcohol and eugenol in synthetic cinnamaldehyde forms the basis of its detection in cassia oil (Singhal et al 2001).
There are no tests that guarantee purity per se, but analysis by gas chromatography (GC) can be extremely useful (see Analytical techniques, below). The addition of a similar but cheaper essential oil, for example, will result in some compounds showing up on analysis that should not be there at all. The addition of a compound normally present in the oil will correspondingly reduce the percentages of all the other constituents, and some of these may then fall below normal minimum limits. The addition of a synthetic substance that is not in the natural oil will generally show up on a GC trace. One approach is to search for a single constituent that is present in the natural material, but is not commercially available, so it cannot be added. The concentration of this substance may be revealing, if indeed it is there at all.
Degradation
Oxygen
Atmospheric oxygen can change the chemical composition of an essential oil by reacting with some of the constituents. Oxidation tends to occur more readily in essential oils rich in monoterpenes and other more volatile compounds. Most of the monoterpenes listed in Box 2.2 are alkenes, which are susceptible because carbon–carbon double bonds are reactive to oxygen. Unsaturated fats and oils become rancid for the same reason. However, not all oxidized monoterpenoid alkenes are high-risk allergens. In a multicenter study involving 1,511 consecutive dermatitis patients, only one (0.07%) tested positive to a 3% concentration of oxidized β-myrcene (Matura et al 2005).
One safety implication of chemical degradation is that we cannot be certain of the composition of a degraded oil unless it is retested, and we may therefore be using a mixture of uncertain composition in treatments. Oxidation can also affect the efficacy of an essential oil. Orafidiya (1993) found that oxidized lemongrass oil had lost much of its antibacterial activity when compared to fresh, unoxidized oil. In extensively oxidized samples, antibacterial activity was completely lost. Kishore et al (1996) reported that chenopodium oil lost its antifungal activity after 360 days, although storage conditions were not specified.
Another consequence of degradation is that it can render an essential oil more hazardous. Notably, terpene degradation in certain oils leads to compounds being formed that make the oils potential skin sensitizers, while fresh oils are safe to use. This especially applies to oils rich in α-pinene, δ-3-carene or (+)-limonene. Of five oxidation products of limonene identified by Karlberg et al (1992), (−)-carvone, and a mixture of (Z)- and (E)- isomers of (+)-limonene 1,2-oxide were potent skin sensitizers in guinea pigs, while (Z)- and (E)-carveol were not. Subsequent work identified two further oxidation products as allergens in guinea pigs, the (Z)- and (E)- isomers of limonene-2-hydroperoxide (Karlberg et al 1994a).
Cold (4–6°C) and dark storage of (+)-limonene in closed vessels prevents significant oxidation for 12 months. The addition of BHT (butylated hydroxytoluene, an antioxidant) to limonene retards oxidation to an extent depending on the purity of the materials and the ambient temperature, but in two of the tests oxidation was prevented in air-exposed limonene for 34 and 43 weeks, respectively. There was no direct correlation between the amount of BHT used and the time before oxidation could be detected, and after the BHT was consumed, oxidation proceeded at about the same rate as for limonene without BHT. The concentration of sensitizing oxidation products reached a peak after 10–20 weeks of air oxidation, and then declined due to polymerization of the oxidation products. After 48 weeks the identified oxidation products constituted 14% of the material (Karlberg et al 1994b).
The monoterpene content of lemon oil decreased from 97.1% to 30.7% in 12 months when the oil was stored at 25°C with the cap removed for three minutes every day. However, storage at 5°C, with the cap removed for three minutes once a month resulted in minimal degradation (Sawamura et al 2004).
Citrus oils are especially vulnerable, because they are high in (+)-limonene, and are not rich in antioxidant constituents. The most potent antioxidant constituent found in essential oils is eugenol, followed by thymol and carvacrol (Teissedre & Waterhouse 2000). In a study of different basil oils, there was a strong relationship between antioxidant activity and total phenolic content (Juliani & Simon 2002). Non-phenolic antioxidant constituents include benzyl alcohol, 1,8-cineole, menthol, menthyl acetate, methyl salicylate and thymoquinone (see Constituent profiles). Tea tree oils with a relatively high 1,8-cineole content may be less prone to oxidation, and therefore less prone to skin reactions, than those low in 1,8-cineole. If this was so it would be somewhat ironic, since the Australian tea tree industry has put great emphasis on low-cineole tea tree oils, in the mistaken belief that 1,8-cineole was a skin irritant.
Unsurprisingly, essential oils rich in antioxidant constituents invariably demonstrate antioxidant activity. The oil of Achillea millefolium subsp. millefolium showed significant antioxidant activity, even though it only contained 24.6% of 1,8-cineole (Candan et al 2003). The antioxidant capacity of carvacrol-rich Thymbra capitata and 1,8-cineole-rich Thymus mastichina oils was compared to that of BHT in sunflower oil stored at 60°C. Both essential oils were much more potent, with Thymus mastichina showing 59% inhibition, compared with 20% for BHT (Miguel et al 2003b). Also see Table 9.3, especially those oils that are highly active against lipid peroxidation.
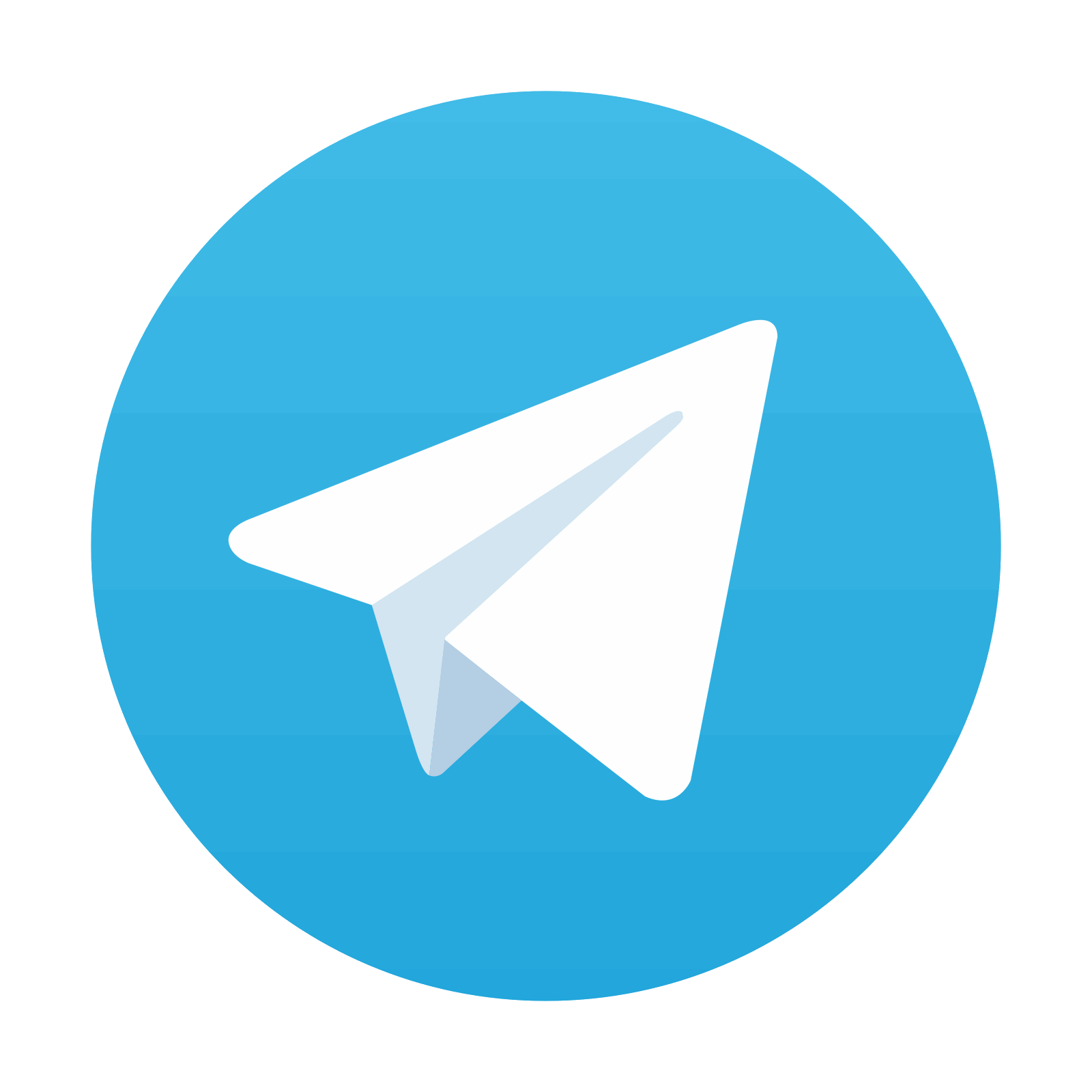
Stay updated, free articles. Join our Telegram channel

Full access? Get Clinical Tree
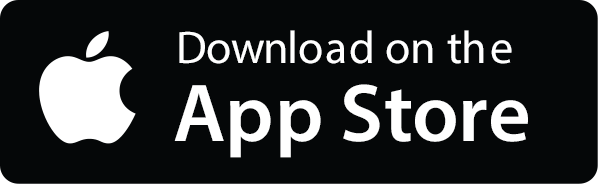
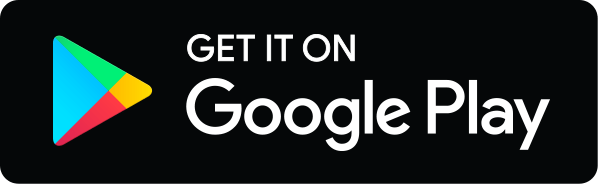