and Jürgen Roth2
(1)
Medical University of Vienna, Vienna, Austria
(2)
University of Zurich, Zurich, Switzerland
Pancreatic Acinus
The pancreas is a combined exocrine and endocrine gland, localized close to the posterior abdominal wall and composed of four anatomic components – a head, a neck, a body, and a tail.
The acinus, as shown cross sectioned in the micrograph on the opposite page, represents the structural and functional secretory unit of the exocrine part of the pancreas (for the endocrine pancreas cf. Figs. 119 and 120).
The exocrine pancreas is the major digestive gland in the human body, draining directly into the duodenum at the ampulla of Vater. It is a tubuloacinar gland, which means that the tubular excretory ducts end up in grape-like bodies, the pancreatic acini, being composed of groups of secretory cells, which produce the precursors of the pancreatic enzymes. The pancreatic fluid (pancreatic juice) contains of a mixture of 22 digestive enzymes. Inactive precursor enzymes are synthesized, processed, and stored in zymogen granules. They are secreted into the acinar lumen in a regulated manner via the vegetative nerve and the endocrine systems, transported along the excretory ducts and finally activated in the lumen of the duodenum, then being able to digest all classes of nutrients.
The grape-like acini are built up by multiple pyramidal secretory cells, which are joined to each other by apical junctional complexes and with their apical surfaces line the lumen in the center of the acinus (AL). The acinar cells’ ultrastructures clearly mirror their functions and remind us that these secretory cells were used as model cells in basic studies of the intracellular pathways of protein synthesis.
Flattened cisternae of the rough endoplasmic reticulum are densely packed in the basal and perinuclear parts of the cells (RER). They define the “ergastoplasm” domain of the cells (cf. Fig. 19) and are the sites of synthesis of the pancreatic enzymes. After co-translational folding, release into the RER-lumen, and passing quality controls, the newly synthesized enzymes are exported out of the ER and taken up into the Golgi apparatus. The Golgi apparatus stacks (Golgi) are in typical supranuclear position. In the Golgi stacks, the newly synthesized enzymes are modified and, at the trans Golgi side, packed into secretory vesicles (for details cf. Fig. 34). The immature secretory vesicles or condensing vacuoles are sites of further processing and condensation of the enzymes; they are intermediates that develop to mature zymogen granules (ZG), containing the densely packed, mostly still inactive precursors of the digestive enzymes. The numerous zymogen granules accumulated in the apical cytoplasm of the cells are the intracellular storage compartments of the proenzymes. The proenzymes are released into the acinar lumen upon specific stimulation after dietary intake.
Situated closely side by side, the acini comprise the major portion of the pancreatic parenchyma. Acini are surrounded by fine connective tissue leading blood capillaries, lymphatics, and unmyelinated nerve fibers (cf. Fig. 148).
References
Palade G (1975) Intracellular aspects of the process of protein synthesis. Science 189:347
Schmidt K, Dartsch H, Linder D, Kern H-F, Kleene R (2000) A submembranous matrix of proteoglycans on zymogen granule membranes is involved in granule formation in rat pancreatic acinar cells. J Cell Sci 113:2233


Fig. 111
Magnification: ×6,200
Acinar Center: Acinar and Centroacinar Cells
The narrow ductuli in the center of the acini build up the initiation of the secretory duct system. Small intercalated canaliculi (intercalated ducts) export the secretion out of the acini and lead to the intralobular excretory ducts, which converge to form interlobular ducts. These, by anastomoses, further build up the main large pancreatic duct.
The micrograph shows details of the center of an acinus. The secretory acinar cells are joined both to each other and to adjacent centroacinar cells (CAC) by junctional complexes (asterisk), composed of a zone of tight junctions, a zone of adhering junctions, and a circle of spot desmosomes (cf. Fig. 98). The apical tight junctions prevent leakage of pancreatic proenzymes from the acinar lumen (AL) into the intercellular spaces.
All parts of the secretory system are displayed in the secretory acinar cells shown in the micrograph: rough endoplasmic reticulum in the basal and lateral parts of the cells, Golgi apparatus (Golgi), condensing vacuoles (CV), and zymogen granules (ZG) dominating in the apical cytoplasm. The increased electron densities of the zymogen granules compared with the condensing vacuoles reflect the condensation processes occurring at this level of the secretory pathway (cf. Figs. 37 and 51). Condensing vacuoles originate from trans Golgi cisternae and therefore are found mostly close to the trans Golgi side. In contrast, the multiple mature zymogen granules are abundant in the entire apical cytoplasm and often take position close to the apical plasma membrane. The contents of the zymogen granules, consisting of mostly still inactive proenzymes, are released into the acinar lumen by regulated exocytosis, which is connected with the fusion of the granule membrane with the apical plasma membrane and formation of a pore (for details cf. Figs. 51, 52, 53, and 54). Proenzyme discharge occurs after dietary intake and is mainly induced by binding of cholecystokinin to specific receptors localized in the basolateral plasma membrane of the acinar cells.
Autophagosomes and lysosomes multiply in the apical cytoplasm of the acinar cells and mirror lysosomal degradation of excessively produced secretory enzymes by a mechanism, common to many secretory cells of the regulated type, called crinophagy. A highly flexible secretory system, with new production of enzymes, storage, release into the acinar lumen, and degradation, is necessary because of the amount of pancreatic fluid produced, and the concentration of enzymes in the zymogen granules varies with the dietary intake. A diet rich in carbohydrates induces a selective production of amylases and a decreased protease synthesis. Insulin produced by the pancreatic islets’ beta cells (cf. Fig. 119) regulates amylase gene expression, an event that stresses the significance of an insulo-acinar portal system, built up by capillaries that leave the endocrine islets and supply blood to the surrounding acini.
Two acinar lumina are on display in the micrograph. They are shown at different functional states and demonstrate the high plasticity of the duct system. The lower acinar ductulus lacks secretory contents, and its wall shows occupation by dense microvilli. In contrast, the upper one apparently is in a state following zymogen discharge. The lumen is filled, the lining plasma membranes are flat, and only a few microvilli protrude into the luminal space. It is evident that the acinar lumina are lined by the apical plasma membranes of both secretory acinar cells and centroacinar cells. Centrocinar cells are the most proximal epithelial cells of the small intercalated ducts and reside in the center of the acini. Centroacinar cells are unique to the pancreas. They lack zymogen granules, contain abundant free polyribosomes and sparse endoplasmic reticulum, and, with their apical domains, together with those of the secretory acinar cells, form the walls of the acinar ductuli.
References
Jahn R, Südhof TC (1999) Membrane fusion and exocytosis. Annu Rev Biochem 68:863
Schmidt K, Dartsch H, Linder D, Kern H-F, Kleene R (2000) A submembranous matrix of proteoglycans on zymogen granule membranes is involved in granule formation in rat pancreatic acinar cells. J Cell Sci 113:2233


Fig. 112
Magnification: ×20,000
Pancreatic Intercalated Duct
The narrow secretory ductuli in the acinar centers (cf. Figs. 111 and 112) continue into small intercalated ducts that lead out of the acini into the connective tissue stroma and further converge to form the intralobular and interlobular excretory ducts. Striated ducts, such as those present in other salivary glands (cf. Fig. 114) and myoepithelial cells, are lacking in the pancreas. Intercalated ducts are surrounded by a basal lamina and are localized within small roads of connective tissue.
Panel A shows a cross section and panel B a longitudinal section through a small pancreatic intercalated duct. In both pictures, pancreatic fluid is present in the lumen and appears as a dense or flocculent content. In panel B, amylase contained in the pancreatic fluid is shown by immunogold labeling.
The pancreatic fluid contains mostly still inactive enzymes, such as trypsin, chymotrypsin, and carboxylpeptidases, which are activated in the duodenal lumen by HCO3 − ions and the alkaline secretion of Brunner’s glands in the submucosa of the duodenum. Trypsin and other proteases are prevented from earlier activation within the ducts by an inhibitor, which is produced and released into the lumen by the secretory acinar cells together with the enzymes.
As occurs in most exocrine glands, the primary secretion is modified in the duct system. Bicarbonate and water are added to the secretion by the intercalated duct epithelium, triggered by specific binding of the small intestinal hormone secretin to plasma membrane receptors.
Multiple basolateral folds, as are visible in the left-hand side of panel A, indicate an involvement of the intercalated duct cells in ion transport. The cells interact with each other by cell-to-cell contacts that build up extensive apical junctional complexes (arrows). In the apical parts of the complexes by a belt of tight junctions, the epithelium is sealed and pancreatic fluid prevented from entering the intercellular spaces.
In the duct lumen shown in panel A, several microvilli and fine cilia appear cross sectioned. Some of them contain microtubuli, resembling the end pieces of kinocilia (cf. Fig. 140).


Fig. 113
Magnification: ×28,000 (A), ×30,000 (B)
Submandibular Gland
This low magnification electron micrograph of a pig submandibular gland shows main functional parts of a compound salivary gland, a secretory end piece with mixed acini (1 and 2), and the proximal portions of the excretory duct system, which include intercalated (3) and striated (4) ducts.
The submandibular gland is a compound tubuloacinar gland that produces a mixed mucous and serous secretion that makes up to 70 % of the saliva. Together with the products of other major and minor salivary glands, the salivary fluid of the submandibular gland has a role that is important in multiple ways in lubricating the surfaces of the oral cavity and forming a thin protective film, dissolving and moistening food, and protecting against microorganisms, with its content of lysozyme, lactoferrin, and immunoglobulin A.
The saliva-producing end-pieces of the gland either are serous acini or form mixed pieces, in which mucous and serous secretory cells coexist in the same acinus. The micrograph shows mixed sero-mucous acini in its right-hand side. Typically, the mucous cells (1), containing abundant, densely packed secretory mucous droplets, reside closer to the lumen of the acinus, compared with the serous cells, and their apical domains build up the luminal walls. The serous cells (2) are localized at the acinus base and form a crescent-like region embracing the mucous cells (serous demilune). Fine intercellular canaliculi connecting the serous cells with the acinar lumen serve to transport the serous secretion. The serous cells are in contact with the basal lamina or are surrounded by myoepithelial cells, which in this very low magnification electron micrograph are hardly discernible. The contractile myoepithelial cells form a kind of basket around the acini and support secretion of the salivary fluid, which is drained sequentially by intercalated ducts, striated ducts, and large interlobular excretory ducts.
Two intercalated ducts (3) are visible in the left upper corner of the micrograph, showing a cuboidal epithelium and flat myoepithelial cells located at the epithelial base. At the left lower corner, a segment of a striated duct (4) is shown. This duct consists of columnar epithelial cells exhibiting multiple basal infoldings that indicate its involvement in ion and water transport. In the striated ducts, kallikrein is secreted, which processes several proteins in the primary saliva. Furthermore, Na+ and Cl− are reabsorbed and the saliva becomes hypo-osmotic. Via transcytosis, IgA secreted by plasma cells in the connective tissue surrounding the acini is transported into both the acinus and striated duct lumina.
The complex duct system of salivary glands is formed during embryonic development by epithelial branching connected with repetitive cleft and bud formation. Growth factors, actin microfilaments, and components of the basement membrane have key roles in the formation of clefts and buds. In particular, fibronectin is important in controlling type III collagen accumulations at clefts. It is assumed that local, developmentally programmed expression of epithelial cell fibronectin might regulate branching morphogenesis associated with the conversion of cell-cell adhesions to cell-matrix adhesions.
Reference
Sakai T, Larsen M, Yamada KM (2003) Fibronectin requirement in branching morphogenesis. Nature 423:876


Fig. 114
Magnification: ×1,500
Goblet Cells: Unicellular Glands
Exocrine glands are highly complex organs composed of single or different secretory cell types, which form secretory units, so-called acini (cf. Figs. 111 and 114). However, there exist secretory cells forming unicellular glands in the mucosa of the respiratory, digestive and urogenital tract as well as in the conjunctiva of the eye and in the gall bladder. The archetype of such a unicellular gland is represented by the flask-shaped, mucus-producing and -storing intestinal goblet cells shown in the electron micrograph. Mucus-producing single secretory cells exist dispersed in the mucosa of various other organs. Mucus, their major secretory product, together with water, ions, and other glycoproteins, forms a highly hydrated, viscoelastic blanket at the surface of the mucosa of various organs. This mucus layer not only protects against mechanical and chemical irritants and bacterial infection, it also prevents dehydration of the mucosa and is a regulator of the specific organ flora.
As seen in the electron micrograph taken from a human duodenal biopsy, a single goblet cell is typically flanked by absorptive enterocytes exhibiting their characteristic apical brush border. The arrows point to intercellular digitations (cf. Fig. 103) formed by the plasma membrane of the neighboring cells. The apical cytoplasm of the goblet cell is filled with mucus droplets. The nucleus and the Golgi apparatus and endoplasmic reticulum cisternae, which has a characteristic wide lumen, are located in the lower half of the cell. The mucus droplets, like other secretory granules (cf. Figs. 51 and 53), are formed in distended parts of trans Golgi cisternae and mature to form membrane-limited, mucin-containing granules (cf. Fig. 52).
The content of the secretory granules of the goblet cells consists of highly glycosylated proteins and is rich in calcium. Mucin proteins consist of a heterogeneous group of secretory and membrane proteins. They all have in common a high content of serine/threonine-linked O-glycans that can make up more than 50 % of their molecular mass. Depending on the tissue and cell type, the apomucin and their O-glycans can differ significantly in structure. Despite this diversity, all mucin proteins have the same kind of domain structure. A central domain consists of repeats of serine and threonine-rich sequences, all of which represent potential glycosylation sites. Both the N- and C-terminal domains are rich in cysteine. De novo synthesized apomucin in the endoplasmic reticulum is modified by N-glycans and becomes dimerized by disulfide bridges at the C-terminal part. Upon transport to the cis Golgi apparatus, apomucin is further modified by O-glycans (cf. Fig. 40). In the trans Golgi apparatus, further dimerization at the C-terminal region takes place, which results in the formation of the mature multimeric mucins.
The content of the mature mucin droplets is released by regulated secretion. In addition to a basal secretion rate, compound secretion can be observed (cf. Fig. 56). Such compound secretion can occur after various stimuli such as nonspecific mechanical and chemical irritation. Specific stimuli involve activators of phospholipase C and of adenylate and guanylate cyclase. Details of the mechanism of regulated secretion are described in Fig. 54.
References
Burgoyne RD, Morgan A (2003) Secretory granule exocytosis. Physiol Rev 83:581
Davis CW, Dickey BF (2008) Regulated airway goblet cell mucin secretion. Annu Rev Physiol 70:487
Dekker J, Rossen JW, Buller HA, Einerhand AW (2002) The MUC family: an obituary. Trends Biochem Sci 27:126
Freeman JA (1966) Goblet cell fine structure. Anat Rec 154:121
Gundelfinger E, Kessels M, Qualmann B (2003) Temporal and spatial coordination of exocytosis and endocytosis. Nat Rev Molec Cell Biol 4:127
Hattrup CL, Gendler SJ (2008) Structure and function of the cell surface (tethered) mucins. Annu Rev Physiol 70:431
Lagow E, DeSouza MM, Carson DD (1999) Mammalian reproductive tract mucins. Hum Reprod Update 5:280
Perez-Vilar J, Hill RL (1999) The structure and assembly of secreted mucins. J Biol Chem 274:31751
Pickett J, Edwardson J (2006) Compound exocytosis: mechanisms and functional significance. Traffic 7:109
Specian RD, Oliver MG (1991) Functional biology of intestinal goblet cells. Am J Physiol 260:C183
Tabak LA (1995) In defense of the oral cavity: structure, biosynthesis and function of salivary mucins. Annu Rev Physiol 57:547
Thornton D, Rousseau K, McGuckin M (2008) Structure and function of the polymeric mucins in airways mucus. Annu Rev Physiol 70:459
Verhage M, Toonen R (2007) Regulated exocytosis: merging ideas on fusing membranes. Curr Opin Cell Biol 19:402


Fig. 115
Magnification: ×10,500
Parietal Cells of Stomach: Secretion of Acid
The parietal cells are located in the middle part of the glands of the fundus-body region of the stomach. They secrete gastric acid, i.e., hydrochloric acid (approximately 0.16 M, pH ≥ 0.8), and the intrinsic factor, which is a vitamin B12-binding protein.
The highly specialized function of the gastric parietal cells is reflected in a particular fine structure (panel A) and it changes dependent on different functional states. Their apical plasma membrane forms numerous channels, the so-called canaliculi, which reach deep into the cytoplasm, as can be easily recognized in longitudinal (asterisks in panel A) and cross sections (asterisk in panel B). The canaliculi are rich in microvilli. Beneath the apical plasma membrane and around the canaliculi is a complex membrane system, which consists of numerous tubulo-vesicles and cisternae (tv in panel C). The tubulo-vesicles are rich in H+, K+-ATPase. Another characteristic feature of parietal cells is their abundance of mitochondria (M). They are required because one ATP molecule is consumed for the coupled export of a single H+ and the import of a single K+. The H+, K+-ATPase of the parietal cells is a P-type ATPase, which consists of a catalytic α-subunit and a β-subunit. The α-subunit contains a recognition sequence for apical sorting and the β-subunit is not only important for the stabilization of the α-subunit but also for recycling because it contains a recognition sequence for endocytosis. The H+, K+-ATPase is the primary gastric proton pump. Under resting conditions, it is preferentially located in the intracellular tubulo-vesicles.
Since its discovery, the exact three-dimensional structure of the membrane system of the parietal cells is a matter of debate. It was and continues to be intensely studied not only by conventional techniques of tissue preparation, but more importantly by high-pressure, rapid-freezing fixation and subsequent freeze-substitution of tissue. The 3D reconstruction is then achieved by serial section analysis. In resting parietal cells, the membrane system was found to be mainly composed of cisternae that were not connected to canaliculi, and of a network of winding tubuli. Following stimulation of acid secretion, translocation of H+, K+-ATPase-containing tubulo-vesicles in the apical plasma membrane occurred, which resulted in an approximately tenfold enlargement of its surface. In parallel, the number of microvilli of the canaliculi increased. In the expanded apical plasma membrane, the presence of H+, K+-ATPase resulted in K+ and Cl− conductance. This led to active proton pumping with secretion of H+ and Cl− and water. Therefore, the H+, K+-ATPase catalyzes the electro-neutral exchange of intracellular protons for extracellular potassium ions, thus generating the enormous proton gradients associated with gastric acid secretion. Upon termination of the stimulus, membrane recycling results in the reestablishment of the elaborate intracellular membrane system. Currently, the recruitment-recycling model of parietal cell activation for gastric acid secretion is most widely accepted.
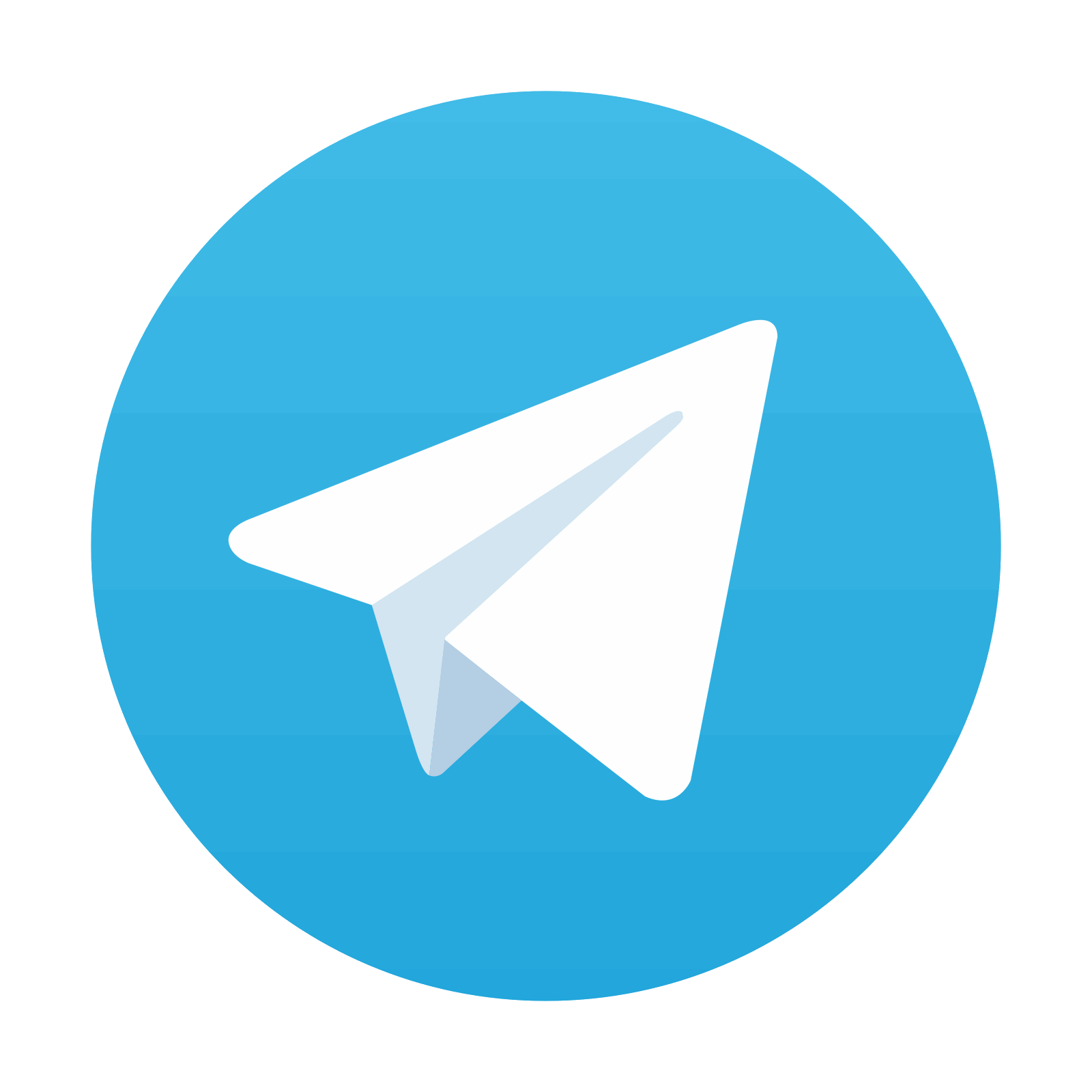
Stay updated, free articles. Join our Telegram channel

Full access? Get Clinical Tree
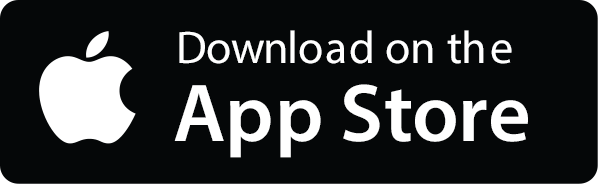
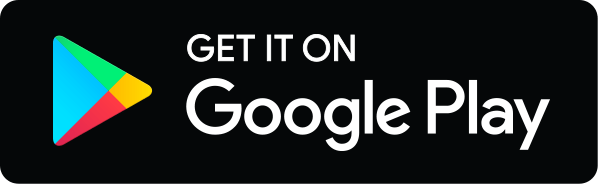