Epilepsy
PREVALENCE
Epilepsy can manifest itself at any age; however, the incidence and prevalence are highest in the very young and the elderly. Depending on age at manifestation, the causes for epilepsy can differ widely. In the United States, there are approximately 1.6 million people who have epilepsy (roughly 0.6% of the population). Epilepsy has a lifetime prevalence of 3%—that is, 7.2 million persons will become affected by this disorder.1 Almost 10% of the population will experience at least one epileptic seizure in 80 years of life.
PATHOPHYSIOLOGY
Two sets of changes can determine the epileptogenic properties of neuronal tissues. Abnormal neuronal excitability is believed to occur as a result of disruption of the depolarization and repolarization mechanisms of the cell (this is termed the excitability of neuronal tissue). Aberrant neuronal networks that develop abnormal synchronization of a group of neurons can result in the development and propagation of an epileptic seizure (this is termed the synchronization of neuronal tissue).2
Various intracellular processes are controlled by genetic information. Neuronal excitability can be preprogrammed by DNA-controlled effects on cell structure, energy metabolism, receptor functions, transmitter release, and ionic channels. The mechanisms that induce these changes, either phasic or long term, appear to be linked to ionic currents, especially Ca2+ influx. Intracellular Ca2+ mediates changes in membrane proteins to initiate transmitter release and ion channel opening; it also activates enzymes to allow neurons to cover or uncover receptor sites that alter neuronal sensitivity. Various plastic or persistent changes in excitability can result by influencing the expression of genetic information through Ca2+ influx. This may occur by selectively inducing genes to synthesize a protein for a specific reason. One example is the induction of the c-fos gene to produce c-fos protein in neurons involved in an epileptic seizure by the administration of pentylenetetrazol. The exact effects of this coupling are not known, but it provides a means to study the effects of neuronal excitation on cell growth and differentiation as a model for epilepsy, learning, and memory.3
In regard to the structural features of neuronal elements in relation to epilepsy, the two primary regions of the brain that are involved in epilepsy are the cerebral neocortex and the hippocampus. In the neocortex, excitatory synapses are made primarily on the dendritic spines and shaft. The release of neurotransmitters at these sites gives rise to excitatory postsynaptic potentials. The inhibitory synapses are more prominent on the soma or proximal dendrites, and give rise to inhibitory postsynaptic potentials. The placement of these synapses effectively prevents distal excitatory events from reaching the axon hillock. Alterations of neuronal morphology, either spontaneously or as a response to injury, could enhance excitability with either an actual increase in the number of excitatory synapses or a decrease in the number of inhibitory synapses. Such alterations could consist of reduced dendritic branching with excitatory synapses placed closer to the axon hillock, or loss of spines, allowing more excitatory synapses to occur directly on the shaft. Lesions in the neuronal cell body or tracts lead to degeneration of the axon terminal and a new terminal may sprout to make contact with the vacated postsynaptic membrane. This may in turn lead to an increase in the excitatory potential of the neuron.4 Ca2+ currents that occur predominantly at the dendrites causing a high-amplitude prolonged depolarization that can evoke a rapid train of Na+ action potentials (burst-firing of Na+), which is followed by a prolonged after-hyperpolarization. These discharges are believed to contribute to the paroxysmal depolarization shifts and after-hyperpolarization in experimental epileptic foci.5
SIGNS AND SYMPTOMS
The diversity of symptoms that can result from an epileptic seizure arises from the differing brain regions that gives rise to the particular features of an individual seizure. The determination of seizure types can often help in the identification of the epileptic syndrome (Table 1). In spite of the technologic advances that have contributed to the understanding and treatment of epilepsy, the initiation and selection of treatment rely on the observed details of the seizure phenomenology. In this regard, obtaining an accurate seizure history from the patient as well as from observers who have witnessed the patient’s seizures is extremely important.
Table 1 Features of Primary Generalized and Partial Epilepsies
Epilepsy | Primary Generalized Epilepsy | Focal or Partial |
---|---|---|
Seizure Features | ||
Auras | Not present | Present |
Prodrome | Occasionally present | Occasionally present |
Starting with LOC | Present | Present |
Starting with automatisms | Not usually present | Present |
Prolonged postictal confusion without generalization | Not usually present | Present |
Generalized tonic-clonic seizure | Present | Present |
True versive head movements | Not present | Present |
Focal motor clonic or tonic seizures | Not usually present | Present |
Risk Factors for Epilepsy | ||
Family history of seizures | May be present | Not usually present |
History of CNS infections, significant head trauma, febrile seizures, CNS tumors, CNS vascular malformation | Not usually present | May be present |
Examination Findings | ||
Neurologic examination | Usually normal | May be abnormal |
Neuroimaging Findings | ||
Brain MRI | Usually normal | May be abnormal |
EEG Findings | ||
Generalized epileptiform activity | Present | Not present |
Focal epileptiform activity | Not present | Present |
CNS, central nervous system; EEG, encephalogram; LOC, loss of consciousness; MRI, magnetic resonance imaging.
Data from Commission on Classification and Terminology of the International League Against Epilepsy. Proposal for revised clinical and electroencephalographic classification of epileptic seizures. Epilepsia 1981;22:489-501.
There have been many attempts at a classification system for epileptic seizures. The most widely used classification system is the one developed by the International League Against Epilepsy (ILAE), which is an electroclinical classification system (Table 2).6 This classification assumes that there is a one-to-one correlation between the phenomenology of the actual seizures and electrical abnormalities on the EEG seen with the seizure. This, however, is not always the case and these exceptions highlight the main weakness of the ILAE classification.
Table 2 International League Against Epilepsy Classification of Epileptic Seizures
Seizure Types | Features |
---|---|
Partial Seizures | *EEG findings suggest focal onset |
Simple Partial Seizures | Consciousness not impaired |
With motor symptoms | |
Focal motor | |
Focal motor march (Jacksonian) | |
Versive | |
Postural | |
Phonatory | Vocalization arrest of speech |
With somatosensory or special sensory symptoms | Simple hallucinations |
Somatosensory | |
Visual | |
Auditory | |
Olfactory | |
Gustatory | |
Vertiginous | |
With autonomic symptoms or signs | Epigastric sensations, pallor, sweating, flushing, piloerection, pupillary dilation |
With psychic symptoms | Disturbance of higher cortical function |
Complex partial seizures | Consciousness impaired |
Absence Seizures | |
Typical absence | Regular and symmetrical 3-Hz †SWC on EEG |
Atypical absence | Irregular slow SWC on EEG |
Myoclonic Seizures | Polyspike or slow SWC on EEG |
Clonic Seizures | Fast activity or slow SWC on EEG |
Tonic Seizures | Low-voltage fast EEG |
Tonic-Clonic Seizures | Rhythm of less than 10 Hz on EEG |
Atonic Seizures | Poly SWC or low-voltage fast |
Data from Commission on Classification and Terminology of the International League Against Epilepsy. Proposal for revised clinical and electroencephalographic classification of epileptic seizures. Epilepsia 1981;22:489-501.
There is an active effort to improve the ILAE classification. One such classification system is already in use in many centers that perform evaluations for epilepsy surgery.7 The advantage of such a semiologic classification is that it does not rely on knowledge of the electrical abnormalities in a patient, which are frequently unavailable. The classification of seizures can be either vague or more specific with this type of classification, depending on the accuracy of the information available.
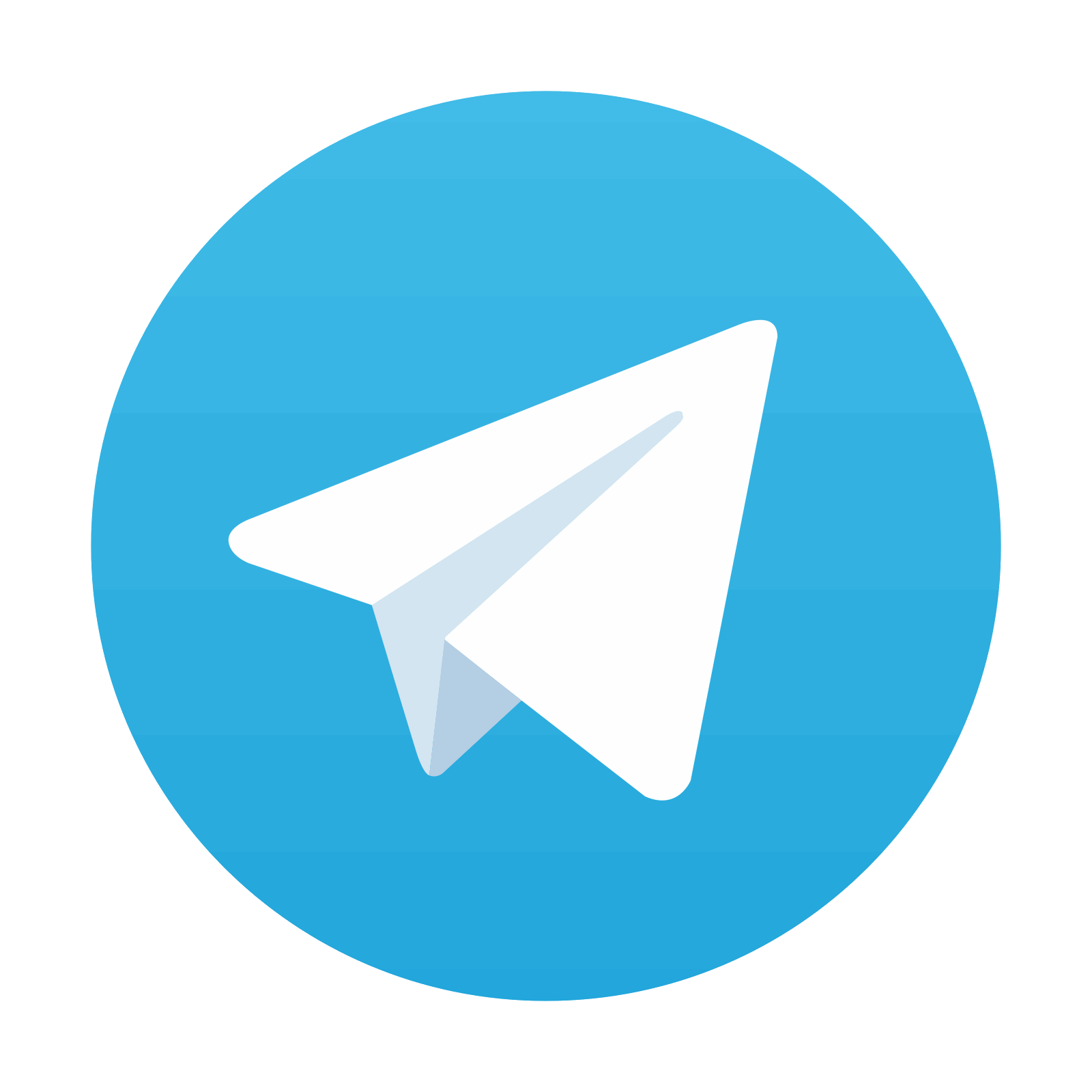
Stay updated, free articles. Join our Telegram channel

Full access? Get Clinical Tree
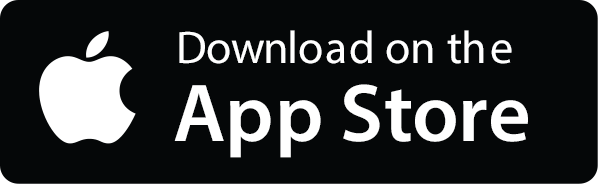
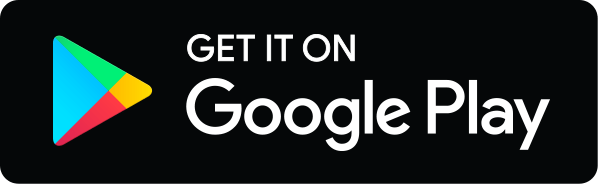