In partial or focal seizures the discharge starts in a localised area of the brain and may remain localised or may secondarily spread to affect the whole brain.
Table 23.1
Simplified classification of epileptic seizures
Seizure type | Characteristics |
Partial (focal) seizures | |
Simple partial seizures | Motor, somatosensory or psychic symptoms; consciousness is not impaired |
Complex partial seizures | Temporal lobe, psychomotor; consciousness is impaired |
Secondary generalised seizures | These begin as partial seizures |
Generalised seizures | Affect whole brain with loss of consciousness |
Clonic, tonic or tonic–clonic | Initial rigid extensor spasm, respiration stops, defaecation, micturition and salivation occur (tonic phase, ≈1 min); violent synchronous jerks (clonic phase, 2–4 min) |
Myoclonic | Seizures of a muscle or group of muscles |
Absence | Abrupt loss of awareness of surroundings, little motor disturbance (occur in children) |
Atonic | Loss of muscle tone/strength |
Unclassified seizures |
Identification of the type of seizure is important in the selection of the most appropriate therapy.
The origin of epilepsy is complex. For most people with epilepsy the initial focus of abnormal neuronal activity is structural damage in the brain such as that resulting from trauma, tumours, cerebrovascular disease or haemorrhage. Seizures can also be caused by metabolic disturbance, such as hypoglycaemia or alcohol abuse. In about 30% of cases of epilepsy where there is no identifiable structural or metabolic disorder (idiopathic epilepsy) there is an important genetic component.
Neurotransmitters and epilepsy
Coordinated activity among neurons depends on a controlled balance between excitatory and inhibitory influences on the electrical activity across neuronal cell membranes. Neuronal networks cooperate by oscillatory electrical activity between different parts of the brain. Generalised epilepsy involves a change from these oscillations to abnormally synchronised activity across large-scale neuronal networks, in particular involving both the cortex and subcortical structures such as the thalamus. Structural changes in neuronal networks in the brain are often found in people with epilepsy and this may provide the basis for generation of the abnormal discharges. These arise from focal lesions in the neocortex and limbic structures (especially hippocampus and amygdala) that promote formation of abnormal regional hyperexcitable circuits.
In healthy neuronal circuits, depolarising inward Na+ and Ca2+ ionic currents are mainly activated by excitatory glutamate N-methyl-D-aspartate (NMDA) receptors. Depolarisation is followed by repolarising outward K+ currents activated by GABAB receptors which act as a feedback inhibitory circuit in response to excitation. Influx of Cl− ions into the neuron produced by GABAA receptor activation hyperpolarises the cell and inhibits impulse generation. Neuropeptide Y is co-released with γ-aminobutyric acid (GABA) and potentiates the inhibition. Membrane-bound ATPase pumps contribute to maintenance of the correct resting membrane potential by active transport of ions across the cell membrane in the resting phase (Ch. 8).
An epileptic seizure probably arises from a localised imbalance between excitatory neurotransmission, principally mediated by glutamate, and inhibitory neurotransmission, mediated by GABA, which leads to a focus of neuronal instability. In some forms of epilepsy there may be a defect in the neuronal currents that results in incomplete repolarisation of the cell. This will leave the neuron closer to its threshold potential for firing and create a hyperexcitable state. Such instability could initiate the burst of firing that produces epileptiform activity. Once an electrical discharge is triggered, spontaneous repetitive firing of the focus is maintained by a feedback mechanism known as post-tetanic potentiation. The synchronisation of the electrical charge that is necessary to generate a seizure may also be enhanced by neural plasticity. Remodelling of the neural connections of an individual GABA neuron can lead to simultaneous hyperpolarisation of a large group of glutamatergic neurons. Neurogenesis can be triggered by seizures and potentiate the development of this type of circuitry, creating a group of hyperpolarised cells. Repolarisation activates currents that generate rebound excitation, producing a synchronised discharge. Generalisation of this discharge probably relies on increased synaptic connections from the excitatory cells.
Several inherited epilepsy syndromes have now been characterised at a cellular level, and arise from mutations of proteins involved in ion channel function. Reduction in the activity of membrane-bound ATPases linked to neuronal transmembrane ion pumps has also been found in the brains of people with primary generalised epilepsy. Ion channel dysfunction may therefore provide the basis for the genesis of many types of generalised seizures, but defective GABA-mediated inhibitory neurotransmission also appears to be a key factor. The genesis of partial seizures is less well understood. These circuits may be generated by disruption of glial cell function and changes in the neuronal microenvironment.
Antiepileptic drugs
Most antiepileptic drugs produce their main antiepileptic effects either by blockade of depolarising ion channels, or by enhancing the inhibitory actions of GABA. Many drugs have multiple sites of action, so the drugs below are grouped by their principal mode of action.
Sodium channel blockers
Carbamazepine, oxcarbazepine, eslicarbazepine
Mechanism of action and uses: Carbamazepine and oxcarbazepine are effective in most types of epilepsy, except for myoclonic epilepsy and absences, which they can exacerbate. Eslicarbazepine is the major active metabolite of oxcarbazepine, given as an acetate prodrug. Their mechanisms of action are incompletely understood but include:

Carbamazepine and oxcarbazepine are also used in the management of trigeminal neuralgia (Ch. 19), and carbamazepine in the management of diabetic neuropathy (Ch. 19) and bipolar disorder (Ch. 21).
Pharmacokinetics: Carbamazepine is metabolised in the liver to an active epoxide metabolite. The half-life of carbamazepine is initially very long, at about 1.5 days, but decreases by about a half over the first 2–3 weeks of treatment because of ‘autoinduction’ of its own metabolism in the liver. Seizure control may then require an increase in dose. Transient unwanted neurological effects, which may occur in association with the peak plasma drug concentration when using the conventional formulation of carbamazepine, can be minimised by the use of a modified-release formulation.
Oxcarbazepine is well absorbed orally and is rapidly and extensively converted in the liver to active metabolites, including S-licarbazepine. Eslicarbazepine acetate is a prodrug converted rapidly to S-licarbazepine after oral administration, and is metabolised by glucuronidation and renal excretion.
Unwanted effects: The unwanted effects of oxcarbazepine and eslicarbazepine are less severe than those of carbamazepine.






Phenytoin and fosphenytoin
Mechanism of action and uses: Phenytoin and its prodrug fosphenytoin have a broad spectrum of activity and are effective against all forms of epilepsy, except absences. They have several actions that may contribute to the antiepileptic activity:

Phenytoin is sometimes used in the management of trigeminal neuralgia (Ch. 19).
Pharmacokinetics: Phenytoin is well absorbed from the gut, but this is a slow process. Slow intravenous injection can be used if a rapid onset of action is needed. Intramuscular injection of phenytoin should be avoided since absorption by this route is erratic and muscle damage can occur. Phenytoin is eliminated by hepatic metabolism, but metabolism is readily saturated so its elimination changes from first-order (linear) kinetics to zero-order (non-linear) kinetics (Ch. 2; Fig. 23.1). This occurs in some individuals at plasma drug concentrations below or near the lower end of the therapeutic range. A small change in dose then produces a large change in the plasma concentration (Ch. 2) and the elimination half-life is increased from about 12 h to almost 2 days. Plasma phenytoin concentrations are closely correlated to the clinical effect, and their measurement is useful as a guide to dosing. When the plasma concentration is close to or within the therapeutic range then any increase in dose should be small. Phenytoin is highly protein-bound (about 90%) and can be displaced from its binding sites by sodium valproate and salicylates, which briefly enhance the clinical effect of phenytoin to an unpredictable extent. The concentration of phenytoin in saliva reflects the free drug concentration in plasma, and measurement of the salivary concentration can be useful to guide dose adjustment in pregnancy or renal failure, or to avoid blood sampling in children.
Fig. 23.1 Inter-individual variation in the plasma concentration of phenytoin at steady state in relation to the daily dose.
The figure illustrates the relationship between daily dose and plasma concentrations in three different individuals. The straight line illustrates the increase in plasma concentrations of phenytoin in Patient 1 that would occur if the metabolism were not saturated (i.e. first-order kinetics). Non-linearity within the desired therapeutic range due to saturation of metabolism can lead to difficulties in dosage adjustment.
Fosphenytoin is a prodrug of phenytoin that is only available for parenteral use. It can be given by intramuscular injection (absorption from this route is good, unlike that of phenytoin) or by intravenous infusion, and is completely metabolised to phenytoin.
Unwanted effects: Most unwanted effects of phenytoin and fosphenytoin are dose-related.







Lacosamide
Mechanism of action and uses: Lacosamide is used for adjunctive treatment of refractory partial seizures with or without secondary generalisation. Its major mechanism of action is enhancing the slow inactivation of neuronal Na+ channels, which stabilises cell membranes. It also binds to collapsing response mediator protein-2 (CRMP-2), which is involved in neuronal differentiation and axonal outgrowth, but it is not known whether this is important for the antiepileptic action.
Pharmacokinetics: Lacosamide is well absorbed from the gut and is eliminated by metabolism and also by the kidneys. It has a half-life of about 13 h.
Lamotrigine
Mechanism of action and uses: Lamotrigine has a wide spectrum of efficacy for partial and generalised seizures. It produces use-dependent inhibition of neuronal voltage-gated Na+ channels. Unlike carbamazepine and phenytoin, it selectively targets dendrites of pyramidal neurons that synthesise glutamate and aspartate and lamotrigine reduces glutamate release.
Lamotrigine is also used for prophylaxis of depression in bipolar disorder (Ch. 21).
Pharmacokinetics: Lamotrigine is well absorbed orally and is metabolised in the liver. The half-life is long (15–60 h).



Zonisamide
Mechanism of action and uses: Zonisamide is used for adjunctive treatment of refractory partial seizures with or without secondary generalisation. Its mechanisms of action include:
Pharmacokinetics: Zonisamide is well absorbed from the gut and is eliminated by metabolism. It has a very long half-life in plasma (about 60 h) and binds selectively to red blood cells.
GABA receptor agonists
Mechanism of action and uses: These drugs enhance the action of the inhibitory neurotransmitter GABA (Ch. 20). Clonazepam and clobazam are used orally for prophylaxis, usually as an adjunct to other drugs. Lorazepam, diazepam or clonazepam can be used intravenously to treat individual seizures, or if intravenous access is not available then rectal diazepam or buccal or intranasal midazolam can be used. Intravenous diazepam is formulated as an emulsion to reduce the incidence of thrombophlebitis.
Unwanted effects: These are discussed in Chapter 20. Partial or complete tolerance to the antiepileptic action of benzodiazepines often occurs after about 4–6 months of continuous treatment.
Phenobarbital and primidone
Mechanism of action and effects: These drugs have a wide spectrum of activity and are effective in most forms of epilepsy, but unwanted effects limit their use. Phenobarbital is a barbiturate, and its major mechanism of action is activation of postsynaptic neuronal GABAA receptors (see also Ch. 20). This increases the duration of opening of the transmembrane Cl− channel associated with the receptor, and the neuronal membrane is therefore hyperpolarised and less likely to fire. In contrast to benzodiazepines, the GABA receptors are activated by phenobarbital independently of the presence of the inhibitory amino acid neurotransmitter, but phenobarbital will also potentiate the effect of GABA (Ch. 20). The action of primidone is due in part to its conversion to phenobarbital. Primidone has no advantage over phenobarbital and is generally less well tolerated. People with epilepsy who do not respond to phenobarbital or who tolerate it poorly are unlikely to benefit from primidone.
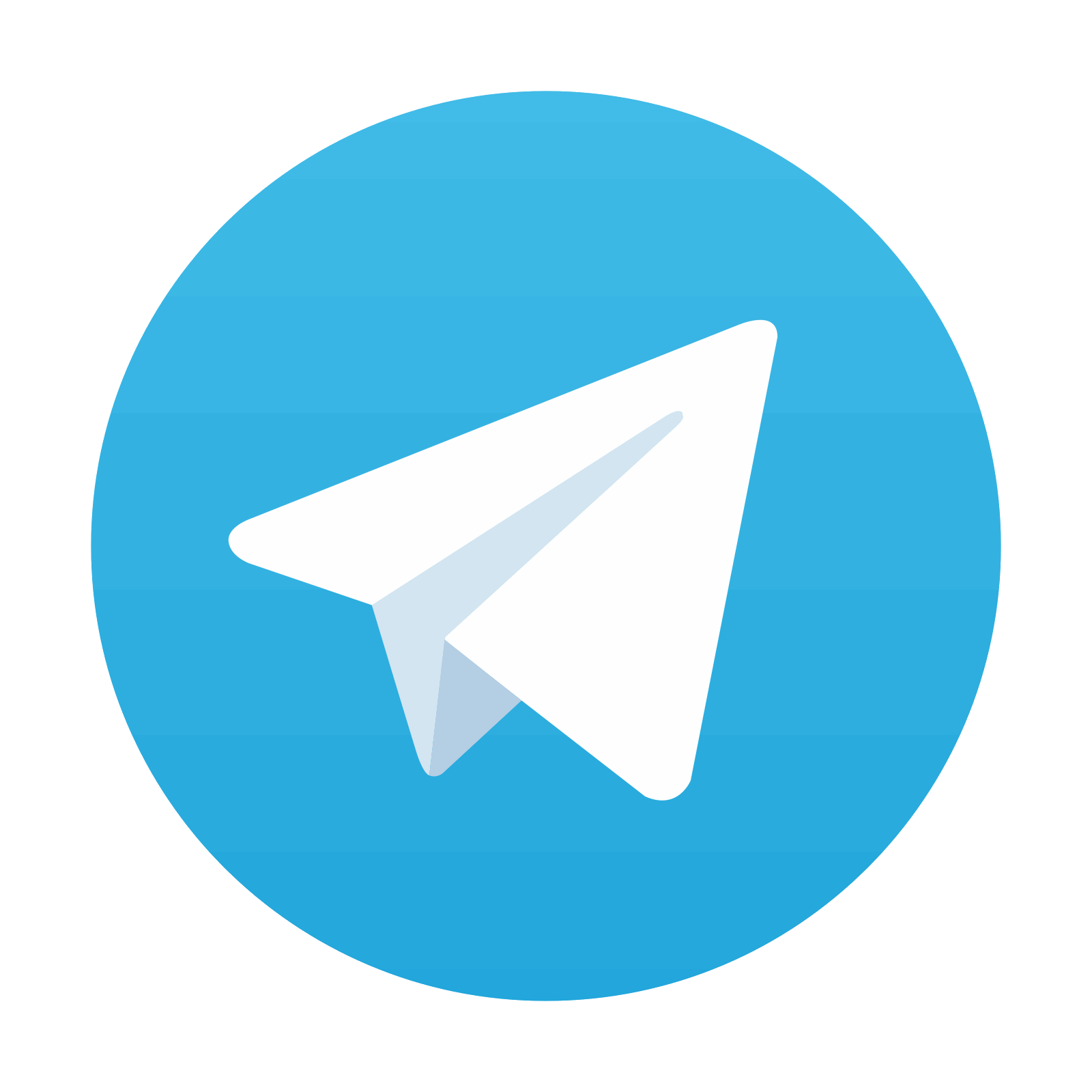
Stay updated, free articles. Join our Telegram channel

Full access? Get Clinical Tree
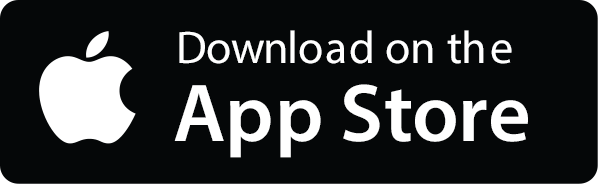
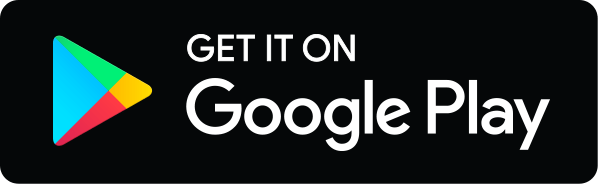