Fig. 10.1
(a) Molecular structure of cocaine in which the arrow points to the ester bond to be broken during butyrylcholinesterase-catalyzed hydrolysis of cocaine. (b) CocE-cocaine binding structure modeled by Liu et al. (2009). (c) Space-filled model of CocE
10.5.1 Bacterial Cocaine Esterase
An inventive group of British investigators (Bresler et al. 2000) found a form of esterase in a strain of the Rhodococcus bacterium growing in the soil around the roots of the coca plant. They isolated and purified this esterase, named it cocaine esterase (CocE), and proceeded to characterize its enzymatic activity. They found that it converts cocaine to ecgonine methyl ester and benzoate, providing an eventual source of nitrogen and carbon as food for the bacterium. The enzyme was highly efficient, much more so than any other naturally occurring enzyme that breaks down cocaine. They sequenced and crystallized the esterase and found that it is composed of 574 amino acids arranged in three domains (Larsen et al. 2002). The active site is located at the intersection of the three domains. Figure 10.1b depicts cocaine located in the active site of the enzyme, and Fig. 10.1c shows a space-filling drawing of the CocE enzyme.
Turner et al. (2002) added significantly to the cellular characterization of CocE by finding that a naturally occurring combination (transesterification) of ethanol and cocaine, called cocaethylene, is also destroyed by CocE; indeed, it did so with almost the same efficiency as it used cocaine as a substrate. Modifications of the structure of cocaine esterase by simple manipulations of single amino acids within the active site completely destroyed its ability to act as an esterase (Turner et al. 2002). This is an enzymatically common fact, but a highly important one, since it illustrates the remarkable selectivity of the enzymes. These investigators also found cocaine esterase to be similarly efficient as did the original discovers of CocE; it is approximately 500 times more efficient than native butyrylcholinesterase in breaking down cocaine. Turner et al. (2002) made a number of important observations regarding the biochemical properties of cocaine esterase. For example, although many enzymes are slowed in efficiency by feedback factors associated with their metabolites, this is not true of cocaine esterase.
Native CocE and modified CocE have been studied extensively in animal models of cocaine toxicity. Native CocE prevents the convulsant and lethal effect of cocaine in mice and rats (Cooper et al. 2006; Ko et al. 2007, 2009). It also reverses the cardiovascular and seizurogenic effects of cocaine in rats (Jutkiewicz et al. 2009; Wood et al. 2010). In addition, Zheng and Zhan (2012) computationally modeled cocaine pharmacokinetics in the presence of a constant concentration of CocE in comparison with native human BChE and CocHs by using available human positron emission tomography (PET) data (Fowler et al. 1989). However, native cocaine esterase is active for only minutes when heated to body temperature (Cooper et al. 2006); presumably, the enzyme evolved to be most efficient at soil temperatures. The short half-life of the wild-type cocaine esterase, considerably less than the half-life of cocaine, may limit its therapeutic usefulness. Gao et al. (2009) and Huang et al. (2012) carried out molecular dynamics (MD) simulations to examine areas of the enzyme that were sensitive to heating and determined amino acid modifications that would enhance its thermostability. These MD simulations were validated by in vivo assessments of esteratic protection against cocaine toxicity in mice (Gao et al. 2009). Slight, critical changes in the structure of the esterase led to durations of action that extended beyond the half-life of cocaine. There were excellent predictions from the model to in vivo function suggesting that thermostability was a major determinant of the enzyme’s half-life in plasma of the mouse (Brim et al. 2010). Interestingly, the single or double exchanges in amino acids that increased thermostability occurred at residues that were far from the active site of the enzyme and therefore did not modify the efficiency of the enzyme (Narasimhan et al. 2010). Because some of these changes in structure extended the enzymatic half-life beyond the normal residence time of cocaine, a single administration of the mutant enzyme was sufficient, in principle, to destroy an intoxicating amount of cocaine.
The mutant, more thermostable, esterase has two amino acids modified (CocE T172R/G173Q; referred to in subsequent descriptions as double mutant or DM CocE). DM CocE is active for 4.5 h in rats; increasing the enzyme’s duration of action does not appear dramatically to slow its onset of action. Collins, Zaks, Cunningham, St Clair, Nichols, Narasimhan et al. (2011) demonstrated that increasing doses of DM CocE reduced the potency of cocaine in producing convulsions and subsequent death in rats. The magnitude of change in potency was on the order of tenfold. A pretreatment dose of 10 mg/kg of DM CocE continued to produce a protective effect against a lethal cocaine dose for 4 h. If DM CocE was administered intravenously after a lethal dose of intraperitoneal cocaine, it could reverse the cardiovascular effects of cocaine as shown in Fig. 10.2, as well as death; it was not determined how close to cocaine-induced death a rat might be and still be rescued by administration of DM CocE. Also of interest, but not studied, was the plasma concentrations of DM CocE and cocaine during the time course of the experiment illustrated in Fig. 10.2.
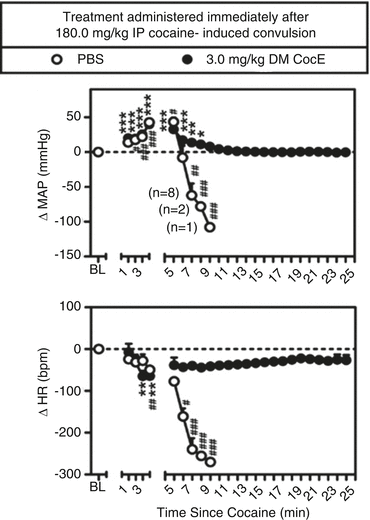
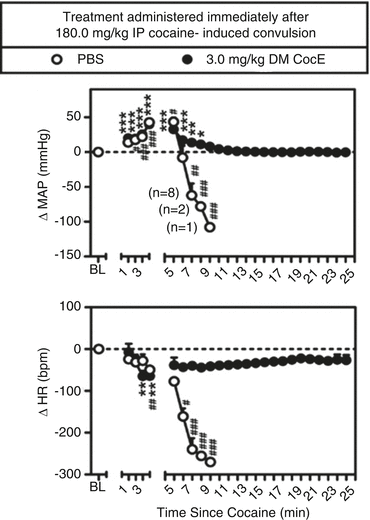
Fig. 10.2
Capacity of DM CocE to rescue rats from the cardiovascular effects of 180.0 mg/kg; IP cocaine. Baseline measures of mean arterial pressure (MAP; mmHg) and heart rate (HR; bpm) were collected for 30 min prior to the IP administration of 180.0 mg/kg cocaine. PBS or 3 mg/kg DM CocE was administered i.v., immediately after the onset of cocaine-induced convulsion, with cardiovascular measures collected for at least 20 min thereafter. Data are expressed as the mean ± SEM (n = 10/group) change in MAP or HR from baseline (1-min bin immediately prior to cocaine administration). Numbers in parentheses represent the number of rats remaining in the group at each time point following the first incidence of lethality. * p < 0.05, ** p < 0.01, *** p < 0.001, # p < 0.05, ## p < 0.01, ### p < 0.001. Significant differences from baseline for the DM CocE (*) and PBS (#) groups were determined using one-way ANOVA with post hoc Dunnett’s tests (Taken with permission from Collins et al. (2011))
DM CocE has been given to rhesus monkeys following the administration of radiolabeled cocaine. PET scans demonstrated that the egress of the labeled cocaine was much faster following DM CocE administration, presumably due to the creation of a strong concentration gradient by CocE-enhanced plasma cocaine elimination. This effect was not obtained with a cocaine analogue without the ester bridge attack point (Howell et al. 2013).
Brim et al. (2011a) reported that DM CocE, given subsequent to cocaine administration, would hydrolyze norcocaine and cocaethylene somewhat less efficiently than it hydrolyzed cocaine, but it did not hydrolyze benzoylecgonine. The latter is a relatively unimportant metabolite, having far weaker capacity to induce cocaine-like effects. This is an important confirmation of an earlier finding with cocaethylene by Turner et al. (2002), and both reports suggest that the enzyme will clear the most active metabolites quickly.
Brim et al. (2011b) determined that DM CocE hydrolytic capacity was not interfered with significantly by a number of commonly abused substances that may be coadministered with cocaine, including alcohol, nicotine, morphine, phencyclidine, ketamine, methamphetamine, naltrexone, naloxone, and midazolam. Diazepam interfered with the enzyme somewhat, but this effect may be unreliable (S. Schwartz, personal communication).
In virtually all cases, the biological fate of the enzymes that utilize cocaine as a substrate has been inadequately studied. An exception is the work of Brim et al. (2012) who found that a labeled form of DM CocE had a half-life of 2.1 h in rats. The primary mechanism of its termination of action was proteolysis, though some was eliminated in urine as well. This suggests the possibility of effective multiple administrations of the enzyme as a potential therapeutic for repeated episodes of toxicity in the same individual.
There may be another advantage to a therapy that works through the elimination of substrate. There may be less species variation relative to, for example, a receptor-based antagonism. This is highly speculative at present given the small number of examples to use as a basis of comparison. Regardless, we are impressed by the lack of differences in effect across species. For example, the effects of DM CocE in rhesus monkey were similar to the rodent examples described above. Collins et al. (2011) showed that in non-human primates, cocaine would produce increases in blood pressure and heart rate that were reduced by the mutant CocE in a dose-dependent fashion over a range of 0.03–3.2 mg/kg i.v. without evoking cardiovascular change on its own. The speed and magnitude of effect were dose dependent. Over the course of studying the enzyme, it was given repeatedly to the monkeys. There were small increases in antibody production to the DM CocE, but they were short-lived and failed to produce a marked change in sensitivity to the hydrolytic activity of the enzyme. Collins et al. (2012) made a more exacting study of repeated dosing to alter cardiovascular effects of cocaine. As shown in Fig. 10.3, repeated administration of 0.32 mg/kg of DM CocE failed to produce a striking change in the hydrolytic capacity of the enzyme rapidly to reduce cocaine blood levels. There were commensurate changes in cardiovascular effects of intravenous cocaine as shown in Fig. 10.4. By the fourth administration of DM CocE, there was a tenfold increase in anti-CocE antibodies. These increases were transient and dissipated within 8 weeks. Both of these studies suggest the possibility that DM CocE might be administered with impunity on more than one occasion to humans for the treatment of intoxication.
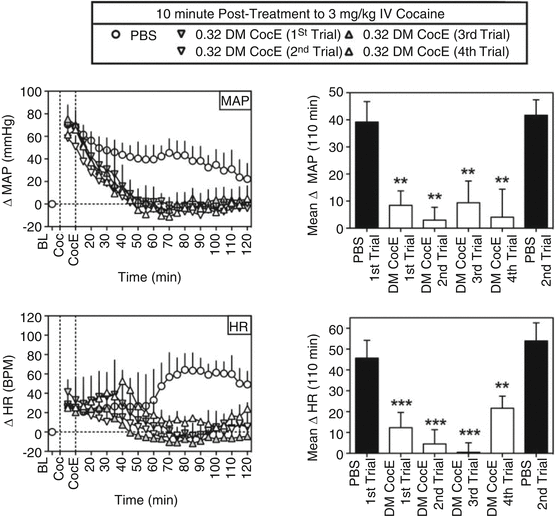
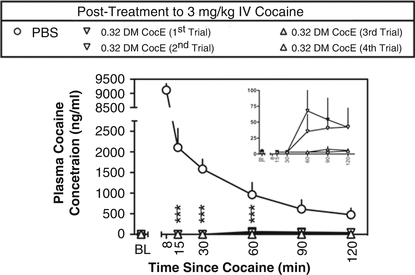
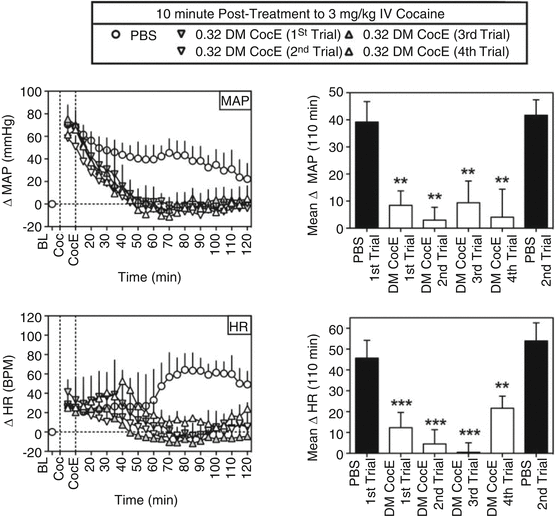
Fig. 10.3
Effects of repeated doses of DM CocE (0.32 mg/kg i.v.) or PBS on the cocaine-induced changes in MAP (top) and HR (bottom) when administered 10 min after an intravenous dose of 3 mg/kg cocaine (n = 3) during six biweekly trials. Left, data points represent the mean ± S.E.M. change from baseline for MAP or HR over successive 5-min blocks of time during sessions in which 3 mg/kg cocaine was followed by PBS (○) or 0.32 mg/kg DM CocE (triangles). Right, data represent the mean ± S.E.M. change from baseline for MAP or HR over the entire 110 min after the administration of PBS (filled bars) or 0.32 mg/kg DM CocE (open bars). **, p < 0.001; ***, p < 0.001. Significant differences in mean change in MAP or HR among conditions were determined by one-way ANOVA with repeated measures with post hoc Bonferroni’s tests (Taken with permission from Collins et al. (2012))
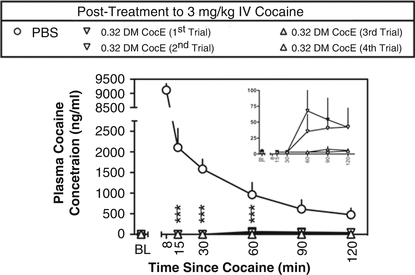
Fig. 10.4
Plasma concentrations of cocaine produced by intravenous doses of 3 mg/kg cocaine during five biweekly trials in two rhesus monkeys. Plasma cocaine concentrations were assessed by mass spectrometry ∼ 2 min before cocaine (baseline; BL) and at 8, 15, 30, 60, 90, and 120 min after the administration of cocaine. ○ represent the mean ± S.E.M. plasma concentrations of cocaine when PBS was administered 10 min after 3 mg/kg cocaine. Plasma concentrations of cocaine during the first (▾), second (▽), third (▴), and fourth (▵) trials in which 0.32 mg/kg DM CocE was administered as a posttreatment to 3 mg/kg cocaine are shown. Data from the 8-min time point were excluded because of differences in the posttreatment times (UR, 10-min posttreatment; BE, 1-min posttreatment). Inset shows plasma cocaine concentrations during the four DM CocE conditions plotted on a y-axis with a smaller range of concentrations. *** p < 0.001. Significant differences in plasma cocaine concentrations were determined by two-way ANOVA with repeated measures and post hoc Bonferroni’s tests (Taken with permission from Collins et al. (2012))
Although a 4 h duration of action is quite satisfactory for the treatment of cocaine toxicity, it is far from satisfactory for the treatment of cocaine abuse. Our group found that DM CocE is active as a dimer in plasma, which is a duplicate and connected version of the enzyme molecule shown in Fig. 10.1 (Narasimhan et al. 2010). Increases in the strength of the linkage between the individual monomers of the pair produced complete thermostability at body temperatures (Narasimhan et al. 2011). The enzyme, however, remained susceptible to peptide degradation in the body. Potential ways to protect against enzyme degradation include additions of polyethylene glycol polymers to the enzymes and/or further enhancement or alteration of the linkage between the monomers. Current research is also being devoted to approaches that provide sustained release of the longer acting versions of the esterases (Sunahara and colleagues, unpublished observations).
10.5.2 Modified Human Butyrylcholinesterase (BChE)
The human endogenous esterase, BChE, has an inefficient capacity to attack cocaine as a substrate. It is by far more efficient at breaking down acetylcholine and the biologically inactive cocaine isomer, (+) cocaine. An early modification of native BChE resulted in a mutant form that had increased capacity to metabolize cocaine (Sun et al. 2002; Table 10.1). Through a series of state-of-the-art molecular dynamic simulations based on the structure of BChE as well as on comparisons of the dynamics of the BChE-(−)-cocaine complex with the BChE-(+)-cocaine complex, modifications of butytylcholinesterase were made that resulted in quadruple and quintuple mutant forms of human BChE that were at least 1000 times more efficient in degrading (−)-cocaine (Pan et al. 2005; Zheng et al. 2008; Zheng and Zhan 2008a, b, 2012; Yang et al. 2010; Xue et al. 2011). Molecular modifications of BChE, in contrast to those made in CocE, were in the active site of the enzyme, far from its surface. Several different forms of mutant BChE, generically termed cocaine hydrolases (CocH), have been described in the literature, as listed and characterized in Table 10.1, and modifications continue to be made to enhance the activity and the duration of action of compounds. The most recently published set of changes resulted in a modified butyrylcholinesterase that surpassed the efficiency of cocaine esterase on a molar basis. Direct comparisons in vivo among the various forms of enzyme would be very interesting, but have not been described. Nevertheless, the altered forms of butyrylcholinesterase are a remarkable improvement in efficiency. The design and implementation of the chemical modifications required to produce these highly efficient anti-cocaine cholinesterases required a number of in silico as well as actual chemical iterations (e.g., Zhan et al. 2003; Zhan and Gao 2005; Zheng et al. 2008; Zheng and Zhan 2009; Zheng et al. 2010).
Table 10.1
Kinetic parameters of human BChE and its mutants against cocaine
Enzyme (BChE or mutant) | k cat (min−1) | K M (μM) | k cat/K M (min−1 M−1) | RCEa |
---|---|---|---|---|
Wild-type BChEb | 4.1 | 4.5 | 9.1 × 10−5 | 1 |
A328W/Y332Ab | 154 | 18 | 8.6 × 106 | 9.4 |
A328W/Y332Gc | 240 | 17 | 1.4 × 107 | 15 |
F227A/S287G/A328W/Y332M (AME359)d | 620 | 20 | 3.1 × 107 | 34 |
A199S/A328W/Y332Ge | 389 | 5.4 | 7.2 × 107 | 79 |
A199S/S287G/A328W/Y332G (CocH1)f | 3060 | 3.1 | 9.9 × 10−8 | 1080 |
A199S/F227A/S287G/A328W/E441D (CocH2)g | 1730 | 1.1 | 1.6 × 10−9 | 1730 |
A199S/F227A/S287G/A328W/Y332G (CocH3)h | 5700 | 3.1 | 1.8 × 10−9 | 2020 |
The Zhan group (Pan et al. 2005) advanced a form of CocH, referred to as CocH1 in Table 10.1. This compound has been fused with human albumin in attempts to increase its in vivo residence time (Gao et al. 2008). At a dose of 10 mg/kg with cocaine delivered 10 min later, CocH1 reduced cocaine toxicity by approximately tenfold. It was effective in both male and female rats. At a dose of 3 mg/kg, it reduced cocaine-induced changes in blood pressure. At the same dose, it reduced cocaine levels in the plasma, heart, and brain of the rat assayed 10 min after cocaine administration (3.5 mg/kg) (Brimijoin et al. 2008).
10.6 Addiction Pharmacotherapy
As noted earlier, the problems of cocaine abuse require a therapy that lasts much, much longer than does therapy for a single episode of intoxication. Although there is no agreement regarding the shortest duration that is necessary and sufficient for treating abuse, the possibility that synthesis of anti-cocaine enzymes can be produced through gene expression raises the possibility of lifetime exposure to the enzyme – certainly an upper limit on duration of protective effect. Although no one is, at present, advocating the possibility of therapy through virally mediated alterations in gene expression, it is at least feasible to entertain this possibility as an eventual therapeutic intervention.
It is clear from the success of long-acting esterases (e.g., DM CocE and CocH1), in reversing or preventing the toxic effects of cocaine, that it might be possible to study the effect of cancellation of cocaine’s stimulus effect in animals. Various assessments have been carried out in rodents that indicate that the reinforcing effects of cocaine can be significantly attenuated with these compounds. It is important to appreciate that there is no way “to erase” the cocaine exposure completely with these pharmacotherapies, but they represent remarkably effective ways of reducing the likelihood that reexposure to cocaine will increase the probability of relapse as long as compliance with therapy administration is maintained.
As described earlier, studies of the reinforcing effects of cocaine are very popular in assessing potential antagonists of cocaine abuse, and these were among the first approaches to be used with anti-cocaine enzymes. When an animal has been trained to administer cocaine and cocaine delivery is then removed by substituting a null substance (e.g., saline), the animal gradually reduces (extinguishes) responding. Not surprisingly, the speed and pattern of decrease in responding depend upon the past history of reinforced responding (e.g., Johanson and Fischman 1989).
10.6.1 CocE
There are two excellent examples of the effects of anti-cocaine enzymes that illustrate the principles of reinforcement theory applied to cocaine. One demonstrates that, as the dose of cocaine esterase is increased, it can produce a partial elimination of cocaine’s reinforcing effect, mimicking a reduction in cocaine dose. If the dose of the enzyme is increased further, it cancels completely the reinforcing effect of cocaine, converting the pattern of cocaine self-administration to one like saline self-administration. These experiments used rats with intravenous catheters that were taught to respond on a lever to deliver i.v. injections of cocaine. Figure 10.5 illustrates the effects of increasing doses of DM CocE on the reinforcing effects of 0.1 mg/kg/inj of cocaine. In the left margin of the figure, the effects of saline substitution for cocaine are shown for comparative purposes. The first active dose of DM CocE (0.3 mg/rat) increased cocaine-maintained responding, just as substituting a smaller dose of cocaine would do. A larger dose of the enzyme (1 mg/rat) reduced cocaine-maintained responding to levels equal to those obtained when saline was substituted for cocaine. If the native, short-acting version of CocE was given prior to a 1-h session of cocaine self-administration, there was a small change in behavior, but only for the period that the cocaine esterase was active (15 min or so). DM CocE failed to change the reinforcing effect of a cocaine analogue without an ester bridge, nor did it change the speed of collecting food reinforcers. The enzyme was therefore acting specifically against cocaine and not acting to suppress behavior in general (Collins et al. 2009).
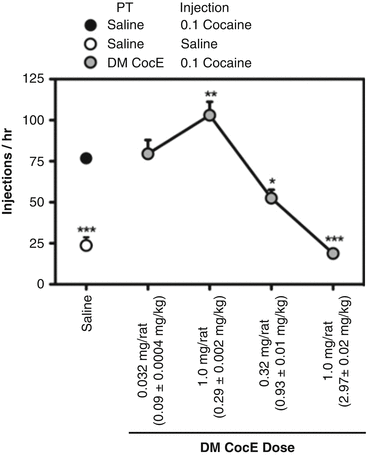
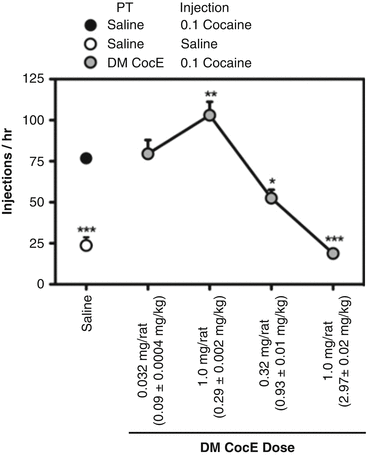
Fig. 10.5
Dose-response analysis of the capacity of CocE T172R/G173Q (DM CocE) to alter responding reinforced by 0.1 mg/kg/injection cocaine. Rats were maintained on 0.1 mg/kg/injection cocaine during daily 60-min sessions in which responding was reinforced under a FR5TO5 schedule of reinforcement. Data represent the mean (±S.E.M.) number of injections earned during 60-min sessions. ● responding under baseline conditions, ○ responding during a single-session saline substitution,
responding during sessions in which rats were pretreated on a milligram per rat basis with 0.032, 0.1, 0.32, or 1.0 mg i.v. DM CocE and allowed to respond for 0.1 mg/kg/injection cocaine. * p < 0.05, ** p < 0.01, *** p < 0.001. Significant differences in responding compared with baseline conditions were determined by one-way ANOVA with post hoc Bonferroni’s tests (Taken with permission from Collins et al. (2009))

Cocaine esterase has been further modified to link the monomers more tightly (CCRQ CocE) and to add polyethylene glycol groups to the enzyme (PEG CCRQ CocE); both approaches extend the residence time of the enzyme without altering the efficiency of the enzyme (Narasimhan et al. 2011). Collins et al. (2012) showed that this form of cocaine esterase, delivered intravenously, was maximally active in reducing the potency of cocaine as a reinforcer immediately after administration. The effect of the esterase was reduced over the course of 3–4 days. At its maximum, the reinforcing potency of cocaine was reduced by more than 30-fold, the reinforcing potency of methylphenidate was unchanged, and rates of food-reinforced responding were unchanged. The discriminative stimulus effects of cocaine were also markedly attenuated by PEG CCRQ CocE; the most effective dose (32 mg/kg) completely eliminated the discriminative stimulus effect of a lethal dose of cocaine (180 mg/kg). A tenfold rightward shift in the potency of cocaine remained 72 h after administration of this dose of PEG CCRQ CocE. The Michigan group is attempting to provide this form of CocE in a sustained-release formulation to gain still further increases in functional, residence time.
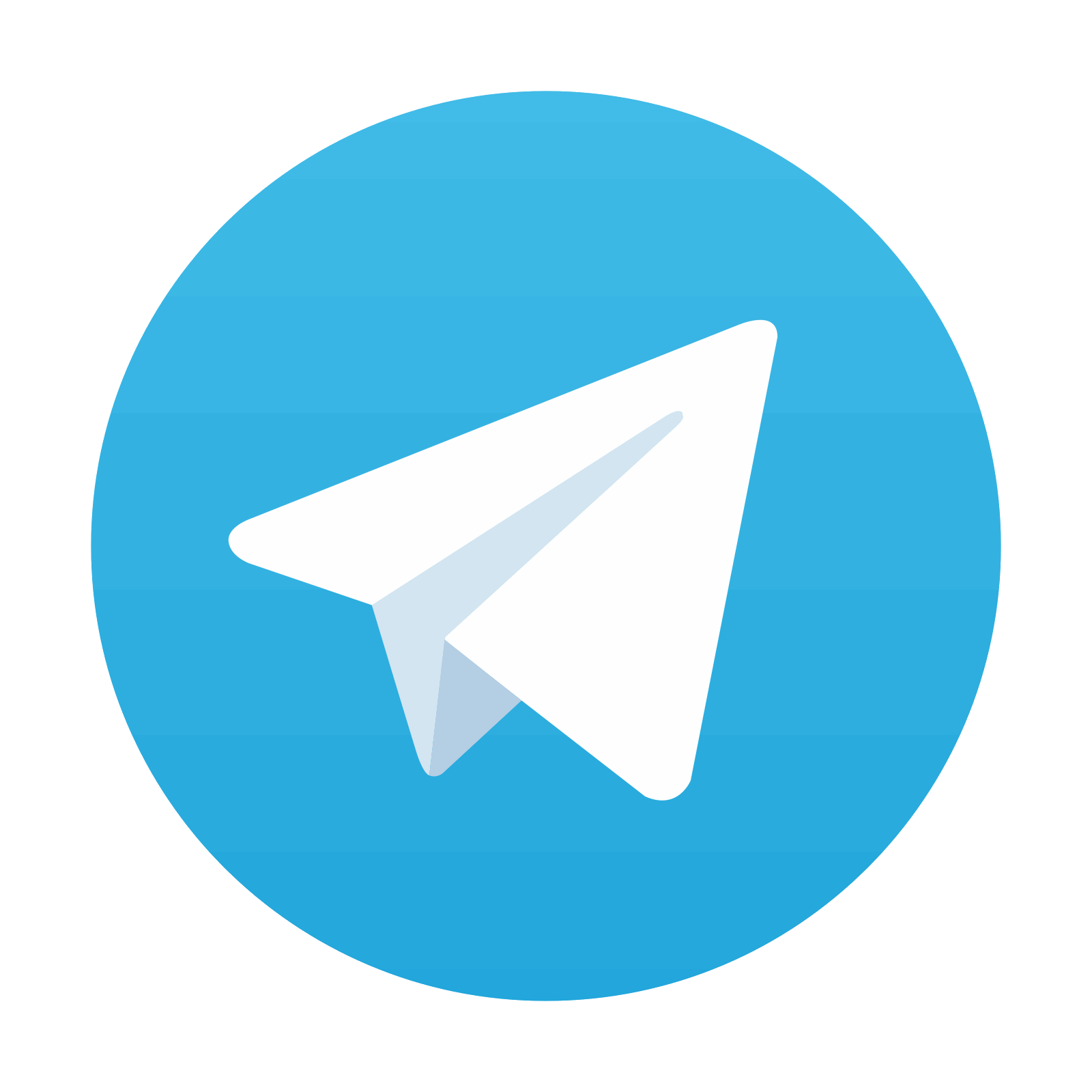
Stay updated, free articles. Join our Telegram channel

Full access? Get Clinical Tree
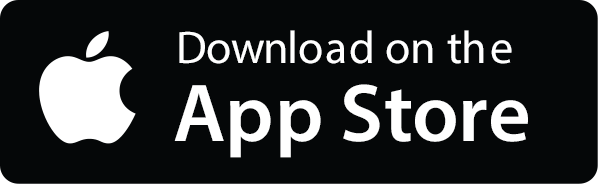
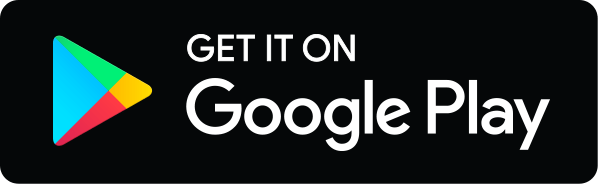