Chapter 3 Genetic, environmental and infectious causes of disease
CAUSES OF DISEASE

In terms of causation, diseases may be:
Features pointing to a significant genetic contribution to the cause of a disease include a high incidence in particular families or races, or an association with a known inherited feature (e.g. gender, blood groups, histocompatibility haplotypes). Environmental factors are suggested by disease associations with occupations or geography. Ultimately, however, only laboratory investigation can provide irrefutable identification of the cause of a disease. The extent to which a disease is due to genetic or environmental causes can often be deduced from some of its main features (Table 3.1).
Table 3.1 Clues to a disease being caused by either genetic or environmental factors
Disease characteristic | Genetic cause | Environmental cause |
---|---|---|
Age of onset | Usually early (often in childhood) | Any age |
Familial incidence | Common | Unusual (unless family exposed to same environmental agent) |
Remission | No (except by gene therapy) | Often (when environmental cause can be eliminated) |
Incidence | Relatively uncommon | Common |
Clustering | In families | Temporal or spatial or both |
Linkage to inherited factors | Common | Relatively rare |
Predisposing factors and precursors of disease
Some diseases predispose to others; for example, ulcerative colitis predisposes to carcinoma of the colon, and hepatic cirrhosis predisposes to hepatocellular carcinoma. Diseases predisposing to tumours are called pre-neoplastic conditions; lesions from which tumours can develop are called pre-neoplastic lesions. Some diseases occur most commonly in those individuals with a congenital predisposition. For example, ankylosing spondylitis, a disabling inflammatory disease of the spinal joints of unknown aetiology, is much more common in people with the HLA-B27 haplotype (Ch. 25).
Some diseases predispose to others because they have a permissive effect allowing environmental agents that are not normally pathogenic to cause disease. For example, opportunistic infections occur in those patients with impaired defence mechanisms, allowing infection by normally non-pathogenic organisms (Ch. 9).
Prenatal factors
Prenatal factors, other than genetic abnormalities, contributing to disease risk are:
The notion that disease risk in adult life could be due to fetal nutritional deprivation has gained support from the work of David Barker. The Barker hypothesis is that an adult’s risk of, for example, ischaemic heart disease and hypertension is programmed partly by nutritional deprivation in utero. This is plausible; nutritional deprivation could have profound effects during critical periods of fetal morphogenesis.
Aetiology and age of disease onset
Do not assume that all diseases manifest at birth have an inherited or genetic basis; as noted previously (Ch. 2), diseases present at birth are classified into those with a genetic basis (further subdivided into those in which the genetic abnormality is inherited and those in which the genetic abnormality is acquired during gestation) and those without a genetic basis. Conversely, although most adult diseases have an entirely environmental cause, genetic influences to disease susceptibility and vulnerability to environmental agents are being increasingly discovered.
The incidence of many diseases rises with age because:
Multifactorial aetiology of disease
Many diseases with no previously known cause are being shown to be due to an interplay of environmental factors and genetic susceptibility (Fig. 3.1). These discoveries are the rewards of detailed family studies and, in particular, application of the new techniques of molecular genetics. Diseases of adults in which there appears to be a significant genetic component include:
Evidence for genetic and environmental factors
Studies on migrants
The unusually high incidence of a particular disease in a country or region could be due either to the higher prevalence of a genetic predisposition in the racial or ethnic group(s) in that country or to some environmental factor such as diet or climatic conditions. Compelling evidence of the relative contributions of genetic and environmental factors in the aetiology and pathogenesis of a disease can be yielded by observations on disease incidence in migrant populations (Fig. 3.2). For example, if a racial group with a low incidence of a particular disease migrates to another country in which the disease is significantly more common, there are two possible outcomes leading to different conclusions:
Association with gene polymorphisms
The polymorphisms of greatest relevance to disease susceptibility are:
HLA types
Clinical and experimental observations on the fate of organ transplants led to the discovery of genes known as the major histocompatibility complex (MHC). In humans, the MHC genes reside on chromosome 6 and are designated HLA genes (human leukocyte antigen genes). HLA genes are expressed on cell surfaces as substances referred to as ‘antigens’, not because they normally behave as antigens in the host that bears them, but because of their involvement in graft rejection (Ch. 9). The body does not normally react to these substances, because it is immunologically tolerant of them and they are recognised as ‘self’ antigens.
HLA types are grouped into classes, principally:
Diseases may be associated with HLA types because:
Diseases associated with HLA types are listed in Table 3.2. They are all chronic inflammatory or immunological disorders. In some instances the association is so strong that HLA testing is important diagnostically: the best example is the association of HLA-B27 with ankylosing spondylitis (Ch. 25).
Table 3.2 Examples of disease associated with HLA types
Disease | HLA type(s) | Comments |
---|---|---|
Allergic disorders (e.g. eczema, asthma) | A23 | Requires environmental allergen |
Ankylosing spondylitis | B27 | Associated in c. 90% of cases |
Coeliac disease | DR3, B8 | Gluten sensitivity |
Graves’ disease (primary thyrotoxicosis) | DR3, B8 | Due to thyroid-stimulating immunoglobulin |
Hashimoto’s thyroiditis | DR5 | Aberrant HLA class II expression on thyroid epithelium |
Insulin-dependent (juvenile onset) diabetes mellitus | DR3, DR4, B8 | Immune injury to beta-cells in pancreatic islets |
Rheumatoid disease | DR4 | Autoimmune disease |
Autoimmune diseases (diseases in which the body’s immunity destroys its own cells) are most frequently associated with specific HLA types. The combination of HLA-DR3 and HLA-B8 is particularly strong in this regard, but it must be emphasised that it is present in only a minority of patients with autoimmune disease. Autoimmune diseases also illustrate a separate feature of the association between HLA types and disease. Normally, class II types are not expressed on epithelial cells. However, in organs affected by autoimmune disease, the target cells for immune destruction are often found to express class II types. This expression enables their immune recognition and facilitates their destruction.
Blood groups
Blood group expression is directly involved in the pathogenesis of a disease only rarely; the best example is haemolytic disease of the newborn due to rhesus antibodies (Ch. 23). A few diseases show a weaker and indirect association with blood groups. This association may be due to genetic linkage; the blood group determinant gene may lie close to the gene directly involved in the pathogenesis of the disease.
Examples of blood group-associated diseases include:
Cytokine genes
Associations have been found between a tumour necrosis factor (TNF) gene polymorphism and Graves’ disease of the thyroid (Ch. 17) and systemic lupus erythematosus (Ch. 25). The TNF gene resides on chromosome 6 between the HLA classes I and II loci, linkage with which may explain an indirect association between TNF gene polymorphism and disease. There are also associations between interleukin-1 gene cluster (chromosome 2) polymorphisms and chronic inflammatory diseases. The associations seem to be stronger with disease severity than with susceptibility.
Racial differences
Racial differences in disease incidence may be genetically determined or attributable to behavioural or environmental factors. Racial differences may also reflect adaptational responses to the threat of disease. A good example is provided by malignant melanoma (Ch. 24). Very strong evidence implicates ultraviolet light in the causation of malignant melanoma of the skin; the highest incidence is in Caucasians living in parts of the world with high ambient levels of sunlight, such as Australia. The tumour is, however, relatively uncommon in Africa, despite its high sunlight levels, because the indigenous population has evolved with an abundance of melanin in the skin; they are classified racially as blacks and benefit from the protective effect of the melanin in the skin.
Some abnormal genes are more prevalent in certain races. For example, the cystic fibrosis gene is carried by 1 in 20 Caucasians, whereas this gene is rare in blacks and Asians. Conversely, the gene causing sickle cell anaemia is more common in blacks than in any other race. These associations may be explained by a heterozygote advantage conferring protection against an environmental pathogen (Table 3.3).
Table 3.3 Associations between disease and race
Disease | Racial association | Explanation |
---|---|---|
Cystic fibrosis | Caucasians | Hypothesised that defective gene increases resistance to intestinal infection by Salmonella bacteria |
Sickle cell anaemia (HbS gene) | Blacks | Sickle cells resist malarial parasitisation |
HbS gene more common in blacks in areas of endemic malaria | ||
Haemochromatosis | Caucasians | Mutant HFE protein may have conferred protection against European plagues caused by Yersinia bacteria |
GENETIC ABNORMALITIES IN DISEASE


Advances in genetics and molecular biology have revolutionised our understanding of the aetiology and pathogenesis of many diseases and, with the advent of gene therapy, may lead to their amelioration in affected individuals (Table 3.4).
Table 3.4 Landmarks in genetics and molecular biology
Date | Discovery |
---|---|
1940s | Genes encoded by combinations of only four nucleotides in nuclear DNA |
1950s | |
1960s | |
1970s | |
1980s | |
1990s | Gene therapy |
Early 21st century |
Genetic damage after birth, for example due to ionising radiation, is not present in the germline and causes neither obvious metabolic defects affecting the entire individual, because the defect is concealed by the invariably larger number of cells with normal metabolism, nor structural abnormalities, because morphogenesis has ceased. The main consequence of genetic damage after birth is, therefore, tumour formation (Ch. 11). There is, however, increasing evidence to suggest that cumulative damage to mitochondrial genes contributes to ageing (Ch. 12).
Gene structure and function
Nuclear DNA
Each of the 23 paired human chromosomes contains, on average, approximately 107 base (nucleotide) pairs arranged on the double helix of DNA; genes are encoded in a relatively small proportion of this DNA. To accommodate this length of DNA within the relatively small nucleus, the DNA is tightly folded. The first level of compaction involves wrapping the double helix around a series of histone proteins; the bead-like structures thus formed are nucleosomes. At the second level of compaction, the DNA strands are coiled to form a chromatin fibre and then tightly looped. During metaphase, when the duplicated chromosomes separate before forming the nuclei of two daughter cells, the DNA is even more tightly compacted.
Nuclear genes
The segments of genes encoding for the final product are known as exons; the segments of anonymous DNA between exons are called introns (Fig. 3.3). The exons comprise sequences of codons, triplets of nucleotides each encoding for an amino acid via messenger RNA (mRNA). In addition, there are start and stop codons defining the limits of each gene. Some genes are regulated by upstream promoters. During mRNA synthesis from the DNA template, the introns are spliced out and the exons may be rearranged.
Gene transcription and translation
Under some circumstances, however, the flow of genetic information is reversed. In the presence of reverse transcriptase, an enzyme present in some RNA viruses, a DNA copy can be made from the RNA (Fig. 3.4).

Fig. 3.4 Reverse transcription of DNA from RNA. Normally, the genetic information encoded in DNA is transcribed to RNA and translated into amino acids from which the protein is synthesised. However, some RNA viruses contain reverse transcriptase, an enzyme that produces a DNA transcript of the RNA; this may then be incorporated into the genome of the cell, possibly altering permanently its behaviour and potentially leading to tumour formation (Ch. 11).
Mitochondrial genes
Mitochondria and ageing
Because mitochondria play a key role in intracellular oxygen metabolism, it is hypothesised that defects of mitochondrial genes and the enzymes encoded by them could lead to the accumulation of free oxygen radical-mediated injury. Such injury could include damage to nuclear DNA, thus explaining not only the phenomenon of ageing (Ch. 12) but also the higher incidence of neoplasia in the elderly (Ch. 11).
Techniques for studying genetic disorders
Genetic disorders can be studied at various complementary levels:
At the population level, one is seeking variations in disease that cannot be explained by environmental factors; the study of migrant populations is particularly useful in disentangling the relative contributions made by genetic and environmental factors to the incidence of a disease (p. 31). In families and individuals, one is seeking evidence of the mode of inheritance—whether it is sex-linked or autosomal, whether it is dominant or recessive (Fig. 3.5); in diseases in which the abnormality is poorly characterised, studies of linkage with neighbouring genes (positional genetics) can lead to elucidation of the structure and function of defective and normal proteins. In cells, expression of the protein can be studied. It is, however, chromosomes and genes that have yielded the greatest advances in recent years.
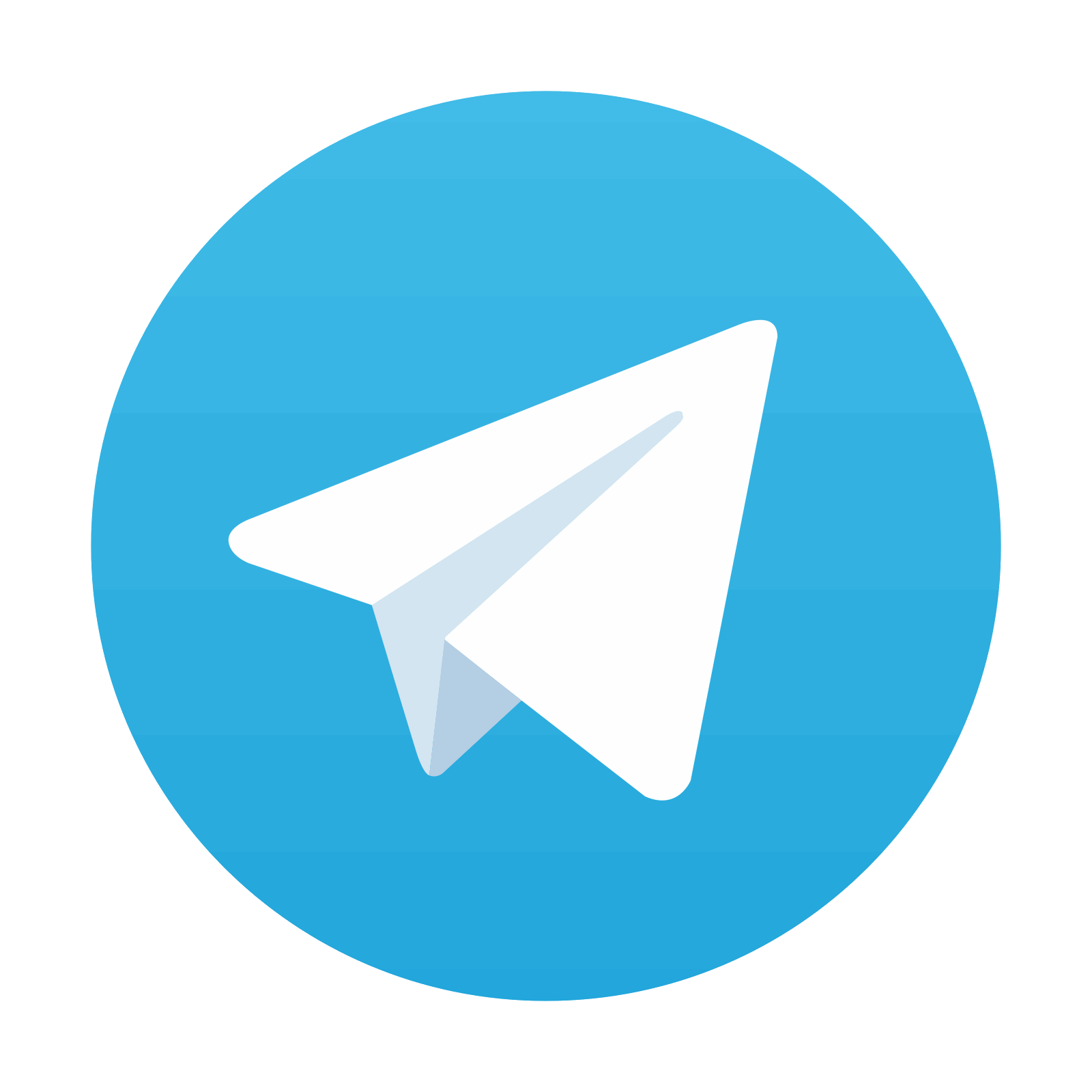
Stay updated, free articles. Join our Telegram channel

Full access? Get Clinical Tree
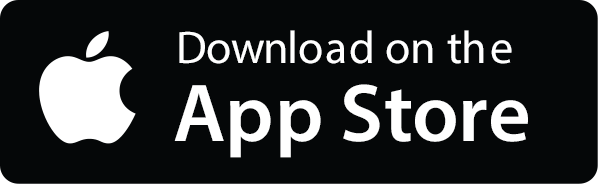
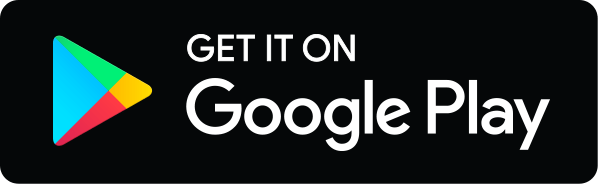